Abstract
Microvesicles (MVs) are released by almost all cells in resting and activated conditions. First described several years ago, it is only recently that their mechanisms of action are being elucidated, and their potential role in health and disease is drawing increasing attention. The main function of MVs is signaling through specific interactions with target cells and the transferring of gene products. Gaining further insights into the molecular specificity of MVs has allowed identification of the cellular source and may provide new diagnostic tools in the future. Indeed, an increasing body of evidence indicates that MVs are capable of mediating tissue repair in models of acute kidney and liver injury. In this review, we will discuss the mechanisms through which MVs from stem cells may act on target cells and may modify the response to injury. Furthermore, MVs from inflammatory cells are suspected to be involved in various diseases, such as cardiovascular and renal diseases, pathological pregnancy, tumors and sepsis. MVs are no doubt also involved in modulating immunity, and future studies will clarify their functional role in negatively modulating the cell response. Their role in physiological and pathological processes is increasingly appreciated. Depending on the cell source and the condition, MVs may be either beneficial or detrimental to the host. The recognition of their pathogenetic role may suggest new approaches to future therapies.
Introduction
Extracellular vesicles, such as exosomes, apoptotic blebs, microvesicles, microparticles, prostasomes and prominosomes were recognized from all cells.Citation1,Citation2 MVs were identified to originate from a large number of cell types in vivo and in vitro: epithelial, fibroblast, hematopoetic, immune, placental, tumor and stem cells.Citation3,Citation4 For long time, MVs were considered to be inert cellular debris and the vesicles frequently observed by electron microscopy in the interstitial space of tissues or in blood were considered the consequence of cell damage or the result of dynamic plasma membrane turnover.Citation5 Recent studies assigned specific functions to vesicles/exosomes released in the microenvironment by various cell types and in biological fluids, such as blood, urine, exudates.Citation6 The recognition of MVs as carriers of genetic information has opened a new era in the field of cell biology, pathophysiology, diagnostics and therapeutics. The role of MVs has been evoked in regenerative medicine and several diseases. In this review, we will delve into the nature of MVs, the mechanisms through which they are known to act on target cells and the experimental evidence that suggests their role in regenerative medicine. We will also very briefly describe the evidence for a role of MVs in tumors and sepsis.
Microvesicles: Biogenesis and Mode of Action
Exosomes are stored as intraluminal vesicles within multivesicular bodies of the late endosome and are released when these multivesicular bodies fuse with the cell membrane. Exosomes have an endosomal origin.Citation7 They are released by exocytosis through a mechanism dependent on cytoskeleton activation and under the regulation of p53 protein.Citation8 While exosomes are a rather homogenous population, with a size of 30 to 120 nm, shedding vesicles are usually larger, with a size ranging from 100 nm to 1 µm. Shedding vesicles originate from the budding of small cytoplasmic protrusions, followed by their detachment from the cell surface, a process dependent on calcium influx, calpain and cytoskeleton reorganization.Citation9 MVs expose on their surface large amounts of phosphatydilserine from the inner leaflet to the outer bilayer of the cell membrane and are enriched in proteins associated with membrane lipid raftsCitation10 by calcium-dependent mechanisms that modify the asymmetric phospholipid distribution of plasma membranes by activation of specific enzymes, named as flippase, floppase and scramblase, and by inhibition of translocase.Citation11 Moreover, the intracellular pathways that activate the reorganization of cytoskeleton induce the detachment of plasma membrane protrusions from the cortical actin. The release of MVs occurs from practically all cells in resting state, upon activation by soluble agonists or upon physical or chemical stress, such as oxidative stress, hypoxia or shear stress.Citation6
The main function of MVs is signaling through specific interactions with target cells and the transfer of genetic information (i.e., mRNA). MVs participate in physiological and pathological processes. Gaining further insights into the molecular specificity of MVs may allow the identification of the cellular source and may provide new diagnostic tools.
MVs influence the behavior of target cells in multiple ways, such as signaling complexes by direct stimulation of target cells by transferring receptors between cells, by delivering proteins to the target cells or by a horizontal transfer of genetic information.
The most recent discovery includes the horizontal transfer of genetic information by MVs. For years, epigenetic changes were frequently reported in co-culture conditions. To explain this phenomenon, the transfer of genetic information between cells was shown.Citation12 MVs derived from human endothelial progenitors (EPC) shuttle mRNA to endothelial cells via interaction with α4- and β1-integrins expressed on their surface, thus activating an angiogenic program.Citation13 The molecular analysis of mRNA indicated that MVs derived from EPC were cargos of a specific subset of cellular mRNA, including mRNA associated with pathways relevant for angiogenesis, such as the PI3K/AKT and eNOS signaling pathways.Citation13 Furthermore, protein expression and functional studies demonstrated that PI3K and eNOS were upregulated in target cells after MV incorporation. More recently, we demonstrated that MVs derived from human stem cells may also deliver in vivo human mRNA to mouse cells, resulting in protein translation.Citation14,Citation15
Besides mRNA, MVs may transfer microRNAs (miRNAs) to target cells.Citation16 Since miRNAs are naturally occurring regulators of protein translation, this observation opens the possibility that stem cells can alter the expression of genes in neighbouring cells by transferring miRNAs contained in MVs. We recently characterized miRNAs shuttled by MVs released by human adult mesenchymal stem cells (MSCs).Citation17 Hierarchical clustering and similarity analysis of miRNAs showed that miRNA compartmentalization and secretion by MVs are both highly regulated processes. Gene ontology analysis of predicted and validated targets showed that the highly expressed miRNAs in MVs derived from MSCs may be involved in multi-organ development, cell survival, differentiation and immune system regulation. It has been suggested that transfer of genetic information by MVs play a pivotal role in stem cell plasticity and tissue regeneration.Citation18–Citation20 This mechanism possibly contributes to the paracrine action of stem cells in the repair of tissue injury.Citation21
Effect of Mesenchymal Stem Cell-Derived MVs in Renal Regeneration
Necrosis and loss of tubular epithelial cells is the most common event in acute kidney injury (AKI), and the recovery of renal function following AKI is dependent on the replacement of necrotic tubular cells with functional tubular epithelium. The possible sources of new tubular cells could be adjacent survival tubular cells that re-enter in cell cycle and proliferate, resident kidney stem and progenitor cells and/or extra-renal cells of bone marrow (BM) origin that home to the injured kidney. In particular, the role of MSCs derived from BM in recovery of kidney injury has been widely investigated (). Administration of heterologous MSCs confers a protective effect against AKI and a functional improvement in chronic kidney disease (CKD), but the explanation for these beneficial effects is still debated. Initially, it was thought that MSCs home to the kidney and replace damaged renal cells; in particular, in AKI induced by cisplatinCitation22,Citation23 and glycerol,Citation24,Citation25 a tubular engraftment of the injected MSCs has been observed. Subsequently, in the ischemia-reperfusion injury (IRI) model, evidence of differentiation of MSCs directly into tubular cells was not been detected,Citation26,Citation27 and these data suggested that the MSCs do not replace renal tubular cells but mitigate injury by providing a paracrine support to the repair of injured tissue. In particular, in ischemia-reperfusion AKI, it was demonstrated that MSCs attached in the renal microvascular circulation and significantly decreased apoptosis of adjacent cells.Citation28 MSCs can exert beneficial effects on tubular repair by producing mitogenic and pro-survival growth factors, such as insulin-like growth factor 1 (IGF-1), as demonstrated in experiments based on the administration of IGF-1 gene-silenced MSC in the murine model of cisplatin-induced AKI.Citation29 Moreover, Togel et al.Citation30 demonstrated that MSC administration after ischemia-reperfusion AKI is not associated with adverse events and is renoprotective in animals with severe renal failure. Interestingly, identical doses of autologous MSCs are more effective than allogenic, and there is no long-term fibrotic response in the kidneys attributable to MSC therapy. Furthermore, this work also establishes that vascular endothelial growth factor (VEGF) could be a critical factor mediating renal recovery. Indeed, VEGF knockdown by siRNA reduces effectiveness of MSC infusion.
A paracrine role of MSCs in renal tissue repair is supported by the beneficial effects of conditioned media, which mimic those exerted by the cells of origin.Citation31 These authors suggested that homing is not an absolute requirement for the beneficial effect of MSC-based therapy, as the intraperitoneal administration of MSC-conditioned medium to mice with cisplatin-induced AKI is sufficient to diminish tubular cell apoptosis, to increase tubular cell survival and to limit renal injury.Citation31 These data indicate that factors secreted by MSCs are responsible for their renoprotective effect, suggesting an endocrine action. However, the nature of the factors responsible for the beneficial paracrine effects of MSCs remains elusive. Beside soluble growth factors, it has been demonstrated that microvesicles (MVs) derived from human BM-MSCs stimulate proliferation and inhibit apoptosis of tubular epithelial cells in vitro and are as effective as MSCs in accelerating functional and morphological recovery from glycerol-induced acute kidney injury (AKI) in immunodeficient mice (SCID)Citation17 ( and ). As the efficacy of MVs is comparable to that of MSC administration in inducing renal regeneration, it is conceivable that the beneficial effect of MSCs is, at least in part, due to the release of MVs. In particular, it has been demonstrated that the injection of MSCs or MSC-derived MVs had a significant protective effect on renal functional recovery, as reflected by lower BUN and creatinine concentrations, with concomitant decrease of histopathologic evidence of tubular injury, such as tubular hyaline casts, vacuolization and widespread necrosis of tubular epithelium. This protection is also associated with significant increase of proliferation in kidneys treated with either MVs or MSCs. RNA inactivation in MVs, by treatment with high concentrations of RNase, reduced both the in vitro and the in vivo effects of MVs, suggesting a mechanism dependent on RNA delivery. In this context, it seems that the transfer of RNAs through MVs may stimulate tubular cell regeneration, inducing dedifferentiation of surviving tubular epithelial cells and triggering a proliferative program that may contribute to the repair of renal injury. Similarly, Ratajczak et al.Citation12 showed that MVs derived from embryonic stem cells may reprogram hematopoietic progenitors by an mRNA-dependent mechanism, and Deregibus et al.Citation13 showed that MVs derived from human endothelial progenitor cells are able to trigger angiogenesis in human vascular endothelial cells by a horizontal transfer of mRNA.
A recent paper extends the effect of MSC-derived MVs to a model of kidney injury induced by ischemia-reperfusion (IRI) in immunocompetent rats. It has been shown that a single administration of MVs derived from adult BM-MSCs immediately after induction of IRI protects rats against AKI and CKD development.Citation32 In this in vivo model, MVs seem to act by a mechanism of renal protection that limits the extent of injury as they significantly reduced tubular cell apoptosis and promoted survival tubular cells to proliferate, indicating that limiting the initial renal injury may also protect the kidney from development of chronic injury. The MVs also significantly reduced the impairment of renal function induced by IRI. Also, in this in vivo IRI model, pre-treatment of MVs with RNase to inactivate their RNA cargo, abrogated these protective effects ( and ).
In conclusion, MVs released from MSCs mimic the effect of the cells, suggesting that they could be exploited as a new therapeutic approach for renal regeneration. There is also evidence that MSCs have a low immunogenicity and have immunoregulatory properties,Citation33 such as in vitro increase in the percentage of Treg at co-culture with T lymphocytesCitation34 and inhibition of the proliferative response of antigen-specific memory T cells,Citation35,Citation36 suggesting that MSC infusion might be a novel approach for immunotherapy. Moreover, infusion of autologous or donor-derived MSCs in mice induced tolerance to a semi-allogenic heart transplantation by a regulatory T-cell mechanism.Citation37 A recent study defined the safety and clinical feasibility of autologous MSC transplantation in two human recipients of kidneys from living related donors.Citation38 In this paper, it has been shown that infusion of MSCs after kidney transplantation is feasible and restricts T memory cell expansion while enlarging the T regulatory population, even if graft dysfunction is induced. In addition, evidence from murine studies has indicated that MSCs could maldifferentiate in glomerular adipocytes once injected in a rat model of progressive glomerulonephritis.Citation39 One possible alternative to the MSC infusion in organ transplantation might be MV treatment. One advantage of using MVs rather than the MSCs themselves is to avoid possible long-term maldifferentiation of engrafted cells or tumor generation.Citation40 Although ischemic and nephrotoxic insults are believed to predominantly target the tubular epithelium, it has become increasingly clear that renal endothelial cells in AKI undergo early damage and swelling,Citation41 causing narrowing of the vascular lumen, followed by impaired microvasculature perfusion.Citation42 The most important consequence of this “no-reflow” phenomenon is a delayed functional recovery of the damaged kidney due to prolonged hypoperfusion. Therefore, the renal microvasculature is of significant importance in terms of tissue regeneration in AKI.
At present, the possibility that endothelial progenitor cells, or EPCs, might functionally contribute to the renal tubule regeneration is poorly studied.
EPCs represent a population of precursors of endothelial cells circulating in the blood stream. In humans, EPCs may derive from CD34+ hematopoietic stem cells or even from more immature CD133+ stem cells co-expressing endothelial markers, such as VEGFR2, tie-2 or VE-cadherin, or even from differentiated CD14+ mononuclear cells.Citation43 Mobilization of these cells from their respective niches is accomplished by mechanical injury and ischemic stress through generation of hypoxia-inducible factor.1-regulated release of vascular endothelial growth factor, erythropoietin and stromal cell-derived factor-1 as well as by placental growth factor, granulocyte colony-stimulating factor (G-CSF) and granulocyte macrophage colony-stimulating factor.Citation44,Citation45 The mechanism of vascular homing of EPC has been studied, and the role of L-selectin has been recognized.Citation46 The main sources of EPC are bone marrow, peripheral blood and the umbilical cord vein. The advantage in the use of EPCs is the easy availability of the cells, which can be expanded from the peripheral blood of the patient or of selected donors.
Several evidences indicate that specialized EPCs, derived from either the bone marrow or other sources, participate in the generation of new blood vessels.Citation47 In particular, several experimental studies, including early clinical studies, have been performed in acute cardiac ischemia.
Not much data has been published on EPC and endothelial regeneration in the kidney. Bone marrow-mediated repair of the glomerular endothelium has been reported by several investigators in anti-Thy-1.1 experimental glomerulonephritis.Citation48 The participation of donor-derived cells in glomerular endothelial cell turnover has also been shown in bone marrow-transplanted rats with unilateral nephrectomy and injection of anti-Thy-1 antibodies.Citation49 As pointed out above, the hemodynamic consequences of renal hypoperfusion, as a result of endothelial cell swelling and narrowing of the lumen, are of significant importance in the course of ARF. In ischemic ARF in immunocompetent mice, the administration of human umbilical vein endothelial cells dramatically improved renal function.Citation50 Chimeric cells expressing endothelial nitric oxide synthase, thus mimicking one of the functions of endothelial cells, were also partially protective, although less so than mature endothelial cells. These studies were extended to EPC, expanded from a skeletal muscle stem cell pool and injected in mice with acute renal ischemia. This procedure was associated with significant preservation of renal function.Citation51 On-going studies suggest an important role of MVs from EPCs in AKI.
Effect of stem cell-derived microvesicles in liver regeneration.
Recent findings indicate that liver regeneration triggered by stem cells depends on the release of paracrine factors.Citation52,Citation53 We recently showed that MVs released by adult stem cells residing in the liver (HLSCs) may, in turn, transfer genetic information from stem cells to target cells that may regulate altered functions and repair damaged tissues without directly replacing parenchymal cells.Citation54 HLSCs are a stem cell population resident in the human adult liver with a mesenchymal phenotype and a partial commitment in hepatocytes that makes them able to induce liver regeneration when injected in acetaminophen-treated mice.Citation55 The in vivo administration of MVs derived from HLSCs was found to accelerate liver regeneration in 70% hepatectomized rats, suggesting that the regeneration process also observed with the administration of HLSCs was due to a paracrine action of the released MVs (). The regenerative action of MVs has been related to an in vivo stimulation of residual mature hepatocytes that survived to injury to re-enter into the cell cycle and undergo proliferation, as indicated by the incorporation of BrdU, the expression of PCNA and of cyclin A1 by hepatocytes. This mechanism was confirmed by in vitro experiments showing that MVs stimulate proliferation and resistance to apoptotic stimuli by human mature hepatocytes. MVs derived from HLSCs contained ibonucleoproteins involved in the intracellular traffic of RNA and selected patterns of miRNAs associated with several cell functions involved in the control of transcription, translation and proliferation.Citation17 This suggested a dynamic regulation of RNA compartmentalization in MVs and raised the possibility that hepatic regeneration depends on the delivery of exogenous mRNA and miRNAs by MVs to hepatocytes. In preliminary studies, we found that MVs derived from HLSC are also able to limit liver injury in the lethal model of GalN/LPS in mice, significantly reducing mortality. In this model, while the administration of HLSC was shown to provide partial functional substitution, MVs mainly acted by limiting the apoptotic injury (Herrera et al., manuscript in preparation).
In another study, we showed that after 48 hours in a rotary bioartificial liver (BAL) (), HLSC acquired markers typically expressed by mature hepatocytes like cytochrome P450, maintained the expression of liver tissue-specific markers, such as CK18, CK8 and albumin, and lost fetal liver markers, that is, AFP and MSC markers, suggesting that low gravity allows HLSCs to spontaneously undergo proliferation and differentiation into mature hepatocytes, a characteristic that was not shown in standard adhesion culture conditions.Citation56 Contrary to adhesion culture conditions, the culture in rotary BAL offered a better microenvironment for the cells, ensuing a more uniform concentration of endogenous growth factors due to rotation. This culture condition also increased the production of MVs from HLSCs and could justify a potential application for rotary BAL as a bridge therapy for liver regeneration.
Perspectives of MVs in Human Pathologic States
Since the introduction of cell therapies, researchers, clinicians and regulatory bodies have all become aware of the multiple risks inherent in the administration of heterologous, intact cellular machinery. Maldifferentiation of mesenchymal cells into adipose cells or transformation of stem cells into tumor cells have been reported. These events are still unpredictable and represent a major hindrance to the full and widespread diffusion of cell therapies. MVs, recently classified by EMEA as advanced therapy products, are then considered as drugs and likewise regulated. However, in respect to intact stem cells, MVs provide the great advantage of being produced in very large concentrations (unpublished results) in highly homogenous preparations. At variance with the originating stem cells, MVs can interact with the target cells and provide a one-hit stimulus.
The pathogenesis of sepsis was initially described as an over-production of pro-inflammatory factors in the host. The concept was established on the basis of several studies. The injection of LPS into the experimental animal and healthy human subjects reproduces the initial phase of bacterial infection.Citation57 The concept of sepsis as a simply pro-inflammatory event has been subsequently challenged.Citation58 In sepsis and SIRS, cell-associated cytokines in peripheral blood mononuclear cells are decreased along with the capacity of these cells to produce several cytokines such as TNFα, IL-10, IL-1β, IL-6, IL-10 and IL-12Citation59 but not IL-1 receptor antagonist.Citation60 Terms such as monocyte deactivation, immunoparalysis or, more simply, cell hyporesponsiveness all indicate the inability of cells to respond ex vivo to LPS stimuli, due to overproduction of anti-inflammatory cytokines. Hyporesponsiveness is not only present in mononuclear cells but it occurs in whole blood,Citation61 and it is associated with increased plasma levels of IL-10 and prostaglandin E2, which are potent inhibitors of the production of proinflammatory cytokines.Citation62 Adib-Conquy et al. demonstrated that, upon LPS activation, peripheral mononuclear cells from patients with SIRS show patterns of NFκB expression that resemble those reported during LPS tolerance: global downregulation of NFκB in survivors of sepsis and trauma patients and the presence of large amounts of the inactive homodimer in non-survivors of sepsis. As shown in cancer, MVs significantly modulate the biological activity of monocyte subsets with a pattern similar to tumor cells. Therefore, tumor cell-derived MVs mimic the activating effect of tumor cells on monocytes as assessed by release of cytokines, ROI and RNI. Likewise, in sepsis, we hypothetize that MVs may have a pleiomorphic activity in the course of sepsis. Initially, MVs may trigger and amplify the inflammatory and coagulation loops. Endothelial microparticle levels in the blood, microparticle binding to PMNLs and oxidative activity in PMNLs increased significantly in patients with severe SIRS, as compared to the healthy controls.Citation64 Whether and to what extent MVs might participate in negatively modulating the pro-inflammatory phase leading to immunoparalyis or cell hyporesponsiness is, to date, unclear. It is conceivable that the identification of selected phenotypes expressed by MVs by specific diagnostics may show whether pro- or anti-inflammatory MVs are predominant and change in response to therapy during the course of the sepsis.Citation65,Citation66
The recent availability of diagnostics capable of quantifying MVs of a specific cell origin could be useful for understanding the cells/organs where injury is occurring. This approach will overlap what has been done in oncology. The mRNA from microvesicles is enriched and differentiated so to obtain a result that is indicative of the condition of tissue or organ from which the microvesicle originated.
In neoplastic diseases, these diagnostic tools could identify and stage by differential analysis of one or more distinct miRNAs, optionally together, with identification and analysis of a non-RNA component of the microvesicle (Microvesicle-Based Compositions and Methods United States Patent Application 20100075315).
An increasing amount of evidence is being accrued on the role of MVs in tumors. Tumor cells release large amount of MVs, and the number of circulating MVs is increased in patients with cancer and correlates with poor prognosis.Citation67 This may depend on the pleyotropic effects of MVs. Thrombotic events are common among different cancer types and stages. In this context, TF is emerging as one of the main mediators involved in the hypercoagulability of cancer patients. MVs expressing TF have a central role in triggering the coagulation cascade. It has been shown that the majority of TF-bearing MVs are of tumor origin.Citation68 MVs may also have a role in the formation of tumor niche, angiogenesis and metastasis (reviewed in ref. Citation69).
Conclusions
The main function of MVs is signaling through specific interactions with target cells and transferring gene products. Therefore, they may participate in physiological and pathological processes. Gaining further insights into the molecular specificity of MVs may allow the identification of the cellular source and may provide new diagnostic tools. MVs have shown to protect from acute renal and hepatic injury. MVs derived from stem cells may reprogram altered functions in target cells suggesting that MVs could be exploited in regenerative medicine to repair damaged tissues. Furthermore, the role of MVs in various diseases (e.g., chronic inflammation, cardiovascular and renal diseases, pathological pregnancy, tumors and sepsis) may make us consider the other side of the coin. Novel therapies that selectively reduce MVs in body fluids may have in the future a beneficial effect on the development and the severity of the disease. Many points require further investigation: (1) the stimuli and the molecular pathways that regulate the assembly within MVs of the biologically active molecules that they shuttle; (2) the stimuli that trigger their release; (3) the surface receptors that may confer selective specificity; (4) the full diagnostic potential of MVs in different pathological conditions; (5) the strategy to inhibit formation or to remove potentially harmful MVs from circulation; (6) the therapeutic exploitation in regenerative medicine of MV ability to modify the phenotype and function of target cells. The recognition of MVs has opened a new era, and new perspectives of investigation are at the horizon.
Conflict of Interest
Ciro Tetta and Stefania Bruno are full-time employees at Sis-Ter S.p.A.
Figures and Tables
Figure 1 Effects of i.v. injection of MVs from human MSCs in rats with AKI induced by ischemia-reperfusion and in mice with AKI induced by glycerol injection, treated or not with MVs derived from human MSCs. Representative micrographs of renal histology (Haematoxylin and Eosin staining) at day 2 after IRI of rats treated or not with 30 µg of MVs and at day 4 after glycerol injection of mice treated or not with 15 µg of MVs. Original magnification: x400. (described in ref. Citation32).
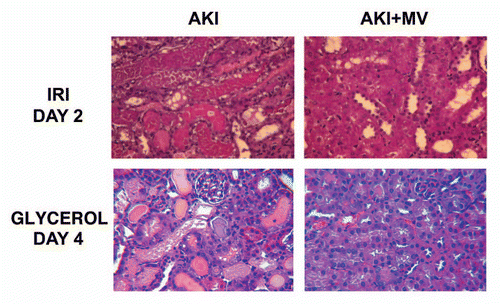
Figure 2 Renal cell proliferation and apoptosis in rats with AKI induced by ischemia-reperfusion and in mice with AKI induced by glycerol injection, untreated or treated with MVs derived from human MSCs. (A) Representative micrographs of PCNA staining and TUNEL assays performed on sections of kidneys 2 days after IRI, treated or not with 30 µg of MVs. Magnification: x400. (B) Representative micrographs of PCNA staining performed on sections of kidneys 4 days after glycerol injection, treated or not with 15 µg of MVs. Magnification: x400. (Described in ref. Citation14).
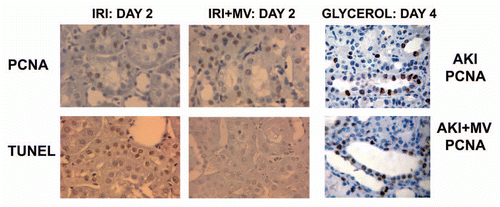
Figure 3 Proliferative effect of HLSC-derived MVs in experimental 70% hepatectomy. Effect of MVs on liver cell proliferation evaluated as BrdU (A and B) and as PCNA (C and D) incorporation in 70% hepatectomized rats. (A and B) Representative micrographs of BrdU uptake performed on sections of liver 24 hrs after 70% hepatectomy in rats treated with vehicle (A) or treated with 30 g MVs (B). BrdU was injected intraperitoneally 2 hrs before rats were killed. Original magnification: x200. (C and D) Representative micrographs of PCNA staining performed on sections of liver 24 hrs after 70% hepatectomy in rats treated with vehicle (C) or treated with 30 g MVs (D). Original magnification: x200. (Described in ref. Citation54).
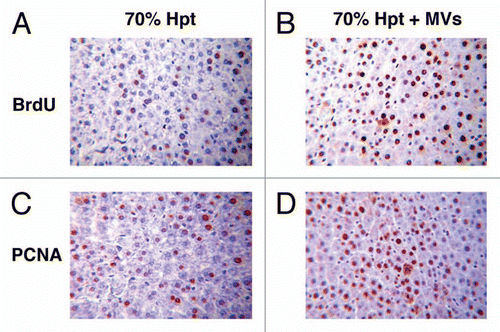
Figure 4 Rotary bioartificial liver device. (A) Photograph of the bioarticificial liver used in the experiments described in reference Citation56. (1) Gas supply: O2, N2, CO2 and pressure air, connected to flowmeter. (2) Pump, regulates media-circulation. (3) Heating device with power supply. (4) Tube system containing bubble traps to avoid air bubbles entering in the circuit. (5) Oxygenator mediates gas flux to media circuit. (6) Chamber containing cells fixed into the reactor's rotation unit. (7) Reactor, housing for chamber and central unit of the rotary bioartificial liver provided by Fresenius Medical Care in cooperation with the University of Innsbruck, Austria. (B) HLSCs are placed in the dialysate compartment followed by their proliferation and aggregate formation around the hollow fibers (insert). HLSCs produce high concentratons of growth factors (e.g., HGF) that are reinfused into the venous line. This makes the rotary bioartificial liver device a source of conditioned media capable of exerting important, hepatotropic effects, such as induction of proliferation and inhibition of apoptosis.
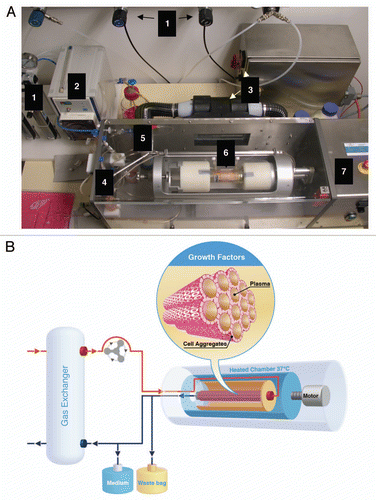
Table 1 Experimental models of acute and chronic kidney injury: Effects of MSCs and their MVs
Table 2 Experimental models of acute and chronic liver injury: Effects of HLSCs and their MVs
Acknowledgments
The studies published by Giovanni Camussi and his co-workers have been funded by grants from Regione Piemonte, Piattaforme Biotecnologiche, project PiSTEM and Oncoprot, from the Ministero dell'Istruzione, dell'Università e della Ricerca (MIUR) project PRIN08 and by Fresenius Medical Care Deutschland GmbH.
References
- Théry C, Zitvogel L, Amigorena S. Exosomes: composition, biogenesis and function. Nature Rewievs Immunology 2002; 2:569 - 579
- Redman CW, Sargent IL. Microparticles and immunomodulation in pregnancy. J Reprod Immunol 2007; 76:61 - 67
- Valenti R, Huber V, Iero M, Filipazzi P, Parmiani G, Rivoltini L. Tumor-released microvesicles as vehicles of immunosuppression. Cancer Res 2007; 67:2912 - 2915
- Pap E, Pallinger E, Pasztoi M, Falus A. Highlights of a new type of intercellular communication: microvesicle-based information transfer. Inflam Res 2009; 58:1 - 8
- Siekevitz P. Biological membranes: the dynamics of their organization. Annu Rev Physiol 1972; 34:117 - 140
- Ratajczak J, Wysoczynski M, Hayek F, Janowska-Wieczorek A, Ratajczak MZ. Membrane-derived microvesicles: important and underappreciated mediators of cell-to-cell communication. Leukemia 2006; 20:1487 - 1495
- Heijnen HF, Schiel AE, Fijnheer R, Geuze HJ, Sixma JJ. Activated platelets release two types of membrane vesicles: microvesicles by surface shedding and exosomes derived from exocytosis of multivesicular bodies and alpha-granules. Blood 1999; 94:3791 - 3799
- Yu X, Harris SL, Levine AJ. The regulation of exosome secretion: a novel function of the p53 protein. Cancer Res 2006; 66:4795 - 4801
- Cocucci E, Racchetti G, Meldolesi J. Shedding microvesicles: artefacts no more. Trends Cell Biol 2008; 19:43 - 51
- Del Conde I, Shrimpton CN, Thiagarajan P, López JA. Tissue-factor-bearing microvesicles arise from lipids rafts and fuse with activated platelets to initiate coagulation. Blood 2005; 106:1604 - 1611
- Hugel B, Martinez MC, Kunzelmann C, Freyssinet JM. Membrane microparticles: two sides of the coin. Physiology 2005; 20:22 - 27
- Ratajczak J, Miekus K, Kucia M, Zhang J, Reca R, Dvorak P, Ratajczak MZ. Embryonic stem cell-derived microvesicles reprogram hematopoietic progenitors: evidence for horizontal transfer of mRNA and protein delivery. Leukemia 2006; 20:847 - 856
- Deregibus MC, Cantaluppi V, Calogero R, Lo Iacono M, Tetta C, Biancone L, et al. Endothelial progenitor cell derived microvesicles activate an angiogenic program in endothelial cells by a horizontal transfer of mRNA. Blood 2007; 110:2440 - 2448
- Bruno S, Grange C, Deregibus MC, Calogero RA, Saviozzi S, Collino F, et al. Mesenchymal stem cell-derived microvesicles protect against acute tubular injury. J Am Soc Nephrol 2009; 20:1053 - 1067
- Herrera MB, Fonsato V, Gatti S, Deregibus MC, Sordi A, Cantarella D, Calogero R, et al. Human liver stem cell-derived microvesicles accelerate hepatic regeneration in hepatectomized rats. J Cell Mol Med 2010; 14:1605 - 1618
- Yuan A, Farber EL, Rapoport AL, Tejada D, Deniskin R, Akhmedov NB, Farber DB. Transfer of microRNAs by embryonic stem cell microvesicles. PLoS One 2009; 4:4722
- Collino F, Deregibus MC, Bruno S, Sterpone L, Aghemo G, Viltono L, et al. Microvesicles derived from adult human bone marrow and tissue specific mesenchymal stem cells shuttle selected pattern of miRNAs. PLoS One 2010; 5:11803
- Quesenberry PJ, Dooner MS, Aliotta JM. Stem cell plasticity revisited: the continuum marrow model and phenotypic changes mediated by microvesicles. Exp Hematol 2010; 38:581 - 592
- Deregibus MC, Tetta C, Camussi G. The dynamic stem cell microenvironment is orchestrated by microvesicle-mediated transfer of genetic information. Histol Histopathol 2010; 25:397 - 404
- Quesenberry PJ, Aliotta JM. The paradoxical dynamism of marrow stem cells: considerations of stem cells, niches and microvesicles. Stem Cell Rev 2008; 4:137 - 147
- Camussi G, Deregibus MC, Tetta C. Paracrine/endocrine mechanism of stem cells on kidney repair: role of microvesicle-mediated transfer of genetic information. Curr Opin Nephrol Hypertens 2010; 19:7 - 12
- Morigi M, Imberti B, Zoja C, Corna D, Tomasoni S, Abbate M, et al. Mesenchymal stem cells are renotropic, helping to repair the kidney and improve function in acute renal failure. J Am Soc Nephrol 2004; 15:1794 - 1804
- Morigi M, Introna M, Imberti B, Corna D, Abbate M, Rota C, et al. Human bone marrow mesenchymal stem cells accelerate recovery of acute renal injury and prolong survival in mice. Stem Cells 2008; 26:2075 - 2082
- Herrera MB, Bussolati B, Bruno S, Fonsato V, Mauriello-Romanazzi G, Camussi G. Mesenchymal stem cells contribute to renal repair on acute tubular epithelial injury. Int J Mol Med 2004; 14:1035 - 1041
- Herrera MB, Bussolati B, Bruno S, Morando L, Mauriello-Romanazzi G, Sanavio F, et al. Exogenous mesenchymal stem cells localize to the kidney by means of CD44 following acute tubular injury. Kidney International 2007; 72:430 - 441
- Duffield JS, Bonventre JD. Kidney tubular epithelium is restored without replacement with bone marrow-derived cells during repair after ishemic injury. Kidney Int 2005; 68:1956 - 1961
- Duffield JS, Park KM, Hsiao LL, Kelley VR, Scadden DT, Ichimura T, et al. Restoration of tubular epithelial cells during repair of the postischemic kidney occurs independently of bone marrow-derived stem cells. J Clin Invest 2005; 115:1743 - 1755
- Tögel F, Weiss K, Yang Y, Hu Z, Zhang P, Westenfelder C. Vasculotropic, paracrine actions of infused mesenchymal stem cells are important to the recovery from acute kidney injury. Am J Physiol Renal Physiol 2007; 292:1626 - 1635
- Imberti B, Morigi M, Tomasoni S, Rota C, Corna D, Longaretti L, et al. Insulin-like growth factor-1 sustains stem cell mediated renal repair. J Am Soc Nephrol 2007; 18:2921 - 2928
- Tögel F, Cohen A, Zhang P, Yang Y, Hu Z, Westenfelder C. Autologous and allogeneic marrow stromal cells are safe and effective for the treatment of acute kidney injury. Stem Cells Dev 2009; 18:475 - 485
- Bi B, Schmitt R, Israilova M, Nishio H, Cantley LG. Stromal cells protect against acute tubular injury via an endocrine effect. J Am Soc Nephrol 2007; 18:2486 - 2496
- Gatti S, Bruno S, Deregibus MC, Sordi A, Cantaluppi V, Tetta C, et al. Microvesicles derived from human adult mesenchymal stem cells protect against ischemia-reperfusion-induced acute and chronic kidney injury. Nephrol Dial Transplant 2011; 26:1474 - 1483
- Crop M, Baan C, Weimar W, Hoogduijn M. Potential of mesenchymal stem cells as immune therapy in solid organ transplantation. Transpl Int 2009; 22:365 - 376
- English K, Ryan JM, Tobin L, Murphy MJ, Barry FP, Mahon BP. Cell contact, prostaglandin E(2) and transforming growth factor beta 1 play non-redundant roles in human mesenchymal stem cell induction of CD4+CD25(High) forkhead box P3+ regulatory T cells. Clin Exp Immunol 2009; 15:149 - 160
- Krampera M, Glennie S, Dyson J, Scott D, Laylor R, Simpson E, Dazzi F. Bone marrow mesenchymal stem cells inhibit the response of naive and memory antigen-specific T cells to their cognate peptide. Blood 2003; 101:3722 - 3729
- Karlsson H, Samarasinghe S, Ball LM, Sundberg B, Lankester AC, Dazzi F, et al. Mesenchymal stem cells exert differential effects on alloantigen and virus-specific T-cell responses. Blood 2008; 112:532 - 541
- Casiraghi F, Azzollini N, Cassis P, Imberti B, Morigi M, Cugini D, et al. Pretransplant infusion of mesenchymal stem cells prolongs the survival of a semiallogeneic heart transplant through the generation of regulatory T cells. J Immunol 2008; 181:3933 - 3946
- Perico N, Casiraghi F, Introna M, Gotti E, Todeschini M, Cavinato RA, et al. Autologous mesenchymal stromal cells and kidney transplantation: A pilot study of safety and clinical feasibility. CJASN 2010;
- Kunter U, Rong S, Boor P, Eitner F, Müller-Newen G, Djuric Z, et al. Mesenchymal stem cells prevent progressive experimental renal failure but maldifferentiate into glomerular adipocytes. J Am Soc Nephrol 2007; 18:1754 - 1764
- Thirabanjasak D, Tantiwongse K, Thorner PS. Angiomyeloproliferative lesions following autologous stem cell therapy. J Am Soc Nephrol 2010; 21:1218 - 1222
- Flores J, DiBona DR, Beck CH, Leaf A. The role of cell swelling in ischemic renal damage and the protective effect of hypertonic solute. J Clin Invest 1972; 51:118 - 126
- Leaf A. Cell swelling. A factor in ischemic tissue injury. Circulation 1973; 48:455 - 458
- Caplice NM, Doyle B. Vascular progenitor cells: origin and mechanisms of mobilization, differentiation, integration and vasculogenesis. Stem Cells Dev 2005; 14:122 - 139
- Ceradini DJ, Kulkarni AR, Callaghan MJ, Tepper OM, Bastidas N, Kleinman ME, et al. Progenitor cell trafficking is regulated by hypoxic gradients through HIF-1 induction of SDF-1. Nat Med 2004; 10:858 - 864
- Bruno S, Bussolati B, Scacciatella P, Marra S, Sanavio F, Tarella C, et al. Combined administration of G-CSF and GM-CSF stimulates monocyte-derived pro-angiogenic cells in patients with acute myocardial infarction. Cytokine 2006; 34:56 - 65
- Biancone L, Cantaluppi V, Duo D, Deregibus MC, Torre C, Camussi G. Role of L-selectin in the vascular homing of peripheral blood-derived endothelial progenitor cells. J Immunol 2004; 15; 173:5268 - 5274
- Asahara T, Murohara T, Sullivan A, Silver M, van der Zee R, Li T, et al. Isolation of putative progenitor endothelial cells for angiogenesis. Science 1997; 275:964 - 967
- Rookmaaker MB, Verhaar MC, van Zonneveld AJ, Rabelink TJ. Progenitor cells in the kidney: Biology and therapeutic perspectives. Kidney Int 2004; 66:518 - 522
- Ikarashi K, Li B, Suwa M, Kawamura K, Morioka T, Yao J, et al. Bone marrow cells contribute to regeneration of damaged glomerular endothelial cells. Kidney Int 2005; 67:1925 - 1933
- Brodsky SV, Yamamoto T, Tada T, et al. Endothelial dysfunction in ischemic acute renal failure: Rescue by transplanted endothelial cells. Am J Physiol Renal Physiol 2002; 282:1140 - 1149
- Arriero M, Brodsky SV, Gealekman O, et al. Adult skeletal muscle stem cells differentiate into endothelial lineage and ameliorate renal dysfunction after acute ischemia. Am J Physiol Renal Physiol 2004; 287:621 - 627
- Parekkadan B, van Poll D, Suganuma K, Carter EA, Berthiaume F, Tilles AW, et al. Mesenchymal stem cell-derived molecules reverse fulminant hepatic failure. Plos One 2007; 2:941
- van Poll D, Parekkadan B, Cho CH, Berthiaume F, Nahmias Y, Tilles AW, Yarmush ML. Mesenchymal stem cell-derived molecules directly modulate hepatocellular death and regeneration in vitro and in vivo. Hepatology 2008; 47:1634 - 1643
- Herrera MB, Fonsato V, Gatti S, Deregibus MC, Sordi A, Cantarella D, et al. Human liver stem cell-derived microvesicles accelerate hepatic regeneration in hepatectomized rats. J Cell Mol Med 2010; 14:1605 - 1618
- Herrera MB, Bruno S, Buttiglieri S, Tetta C, Gatti S, Deregibus MC, et al. Isolation and characterization of a stem cell population from adult human liver. Stem Cells 2006; 24:2840 - 2850
- Fonsato V, Herrera MB, Buttiglieri S, Gatti S, Camussi G, Tetta C. Use of a rotary bioartificial liver in the differentiation of human liver stem cells. Tissue Eng Part C Methods 2010; 16:123 - 132
- Suffredini AF, Fromm RE, Parker MM, et al. The cardiovascular response of normal humans to the administration of endotoxin. N Engl J Med 1989; 321:280 - 287
- Volk HD, Reinke P, Krausch D, et al. Monocyte deactivation: Rationale for a new therapeutic strategy in sepsis. Intensive Care Med 1996; 4:474 - 481
- Munoz C, Carlet J, Fitting C, Misset B, Bleriot JP, Cavaillon JM. Dysregulation of in vitro cytokine production by monocytes during sepsis. J Clin Invest 1991; 88:1747 - 1754
- Marie C, Muret J, Fitting C, et al. Interleukin-1 receptor antagonist production during infectious and non-infectious systemic inflammatory response syndrome. Crit Care Med 2000; 28:2277 - 2282
- Granowitz EV, Porat R, Mier JW, Orencole SF, Kaplanski G, Lynch EA. Intravenous endotoxin suppresses the cytokine response of peripheral blood mononuclear cells in healthy humans. J Immunol 1993; 151:1637 - 1645
- Shapiro L, Panayotatos N, Meydani SN, Wu D, Dinarello CA. Ciliary neurotrophic factor combined with solubile receptor inhibits synthesis of proinflammatory cytokines and prostaglandin-E2 in vitro. Exp Cell Res 1994; 215:51 - 56
- Adib-Conquy M, Asehnoune K, Moine P, Cavaillon JM. Long-term-impaired expression of nuclear factorkappaB and IkappaB alpha in peripheral blood mononuclear cells of trauma patients. J Leukoc Biol 2001; 70:30 - 38
- Hiroshi Ogura H, Tanaka H, Koh T, Fujita K, Fujimi S, Nakamori Y, et al. Enhanced production of endothelial microparticles with increased binding to leukocytes in patients with severe systemic inflammatory response syndrome. J Trauma 2004; 56:823 - 831
- Ardoin SP, Shanahan JC, Pisetsky DS. The role of microparticles in inflammation and thrombosis. Scand J Immunol 2007; 66:159 - 165
- Soriano AO, Jy W, Chirinos JA, Valdivia MA, Velasquez HS, Jimenez JJ, et al. Levels of endothelial and platelet microparticles and their interactions with leukocytes negatively correlate with organ dysfunction and predict mortality in severe sepsis. Crit Care Med 2005; 33:2540 - 2546
- Kim HK, Song KS, Park YS, Kang YH, Lee YJ, Lee KR, et al. Elevated levels of circulating platelet microparticles, VEGF, IL-6 and RANTES in patients with gastric cancer: possible role of a metastasis predictor. Eur J Cancer 2003; 39:184 - 191
- Zwicker JI, Liebman HA, Neuberg D, Lacroix R, Bauer KA, Furie BC, Furie B. Tumor-derived tissue factor-bearing microparticles are associated with venous thromboembolic events in malignancy. Clin Cancer Res 2009; 15:6830 - 6840
- Camussi G, Deregibus MC, Bruno S, Grange C, Fonsato V, Tetta C. Exosome/microvesicle-mediated epigenetic reprogramming of cells. Am J Cancer Res 2011; 1:98 - 100
- Lange C, Tögel F, Ittrich H, Clayton F, Nolte-Ernsting C, Zander AR, et al. Administered MSC enhance recovery from ischemia reperfusion induced acute renal failure in rats. Kidney Inter 2005; 68:1613 - 1617
- Tögel F, Hu Z, Weiss K, Isaac J, Lange C, Westenfelder C. Administered mesenchymal stem cells protect against ischemic acute renal failure through differentiation-independent mechanisms. Am J Physiol Renal Physiol 2005; 289:31 - 42
- Ninichuk V, Gross O, Segerer S, Hoffmann R, Radomska E, Buchstaller A, et al. Multipotent mesenchymal stem cells reduce interstitial fibrosis but do not delay progression of chronic kidney disease in collagen4A3-deficient mice. Kidney Int 2006; 70:121 - 129
- Kunter U, Rong S, Boor P, Eitner F, Müller-Newen G, Djuric Z, et al. Mesenchymal stem cells prevent progressive experimental renal failure but maldifferentiate into glomerular adipocytes. JASN 2007; 18:1754 - 1764
- Behr L, Hekmati M, Fromont G, Borenstein N, Noel LH, Lelievre-Pegorier M, et al. Intra renal arterial injection of autologos MSC in an ovine model in the post-ischemic kidney. Nephron Physiol 2007; 107:65 - 76
- Semedo P, Wang PM, Andreucci TH, Cenedeze MA, Teixeira VP, Reis MA, et al. Mesenchymal stem cells ameliorate tissue damages triggered by renal ischemic and reperfusion injury. Tranpl Proc 2007; 39:421 - 423
- Nemeth K, Leelahavanichkul A, Yuen PS, Mayer B, Parmelee A, Doi K, et al. Bone marrow mesenchymal stem cells attenuate sepsis via prostaglandin E2-dependent reprogramming of host macrophages to increase their interleukin-10 production. Nat Med 2009; 15:42 - 49
- Choi S, Park M, Kim J, Hwang S, Park S, Lee Y. The role of mesenchymal stem cells in the functional improvement of chronic renal failure. Stem Cells Dev 2009; 18:521 - 529
- Semedo P, Correa-Costa M, Antonio Cenedeze M, Maria Avancini Costa Malheiros D, Antonia dos Reis M, et al. Mesenchymal stem cells attenuate renal fibrosis through immune modulation and remodelling properties in a rat remnant kidney model. Stem Cells 2009; 27:3063 - 3073
- Matsuo R, Nakano Y, Ohkohchi N. Platelet administration via the portal vein promotes liver regeneration in rats after 70% hepatectomy. Ann Surg 2011; 253:759 - 763
- Zhang D, Jiang M, Miao D. Transplanted human amniotic membrane-derived mesenchymal stem cells ameliorate carbon tetrachloride-induced liver cirrhosis in mouse. PLoS One 2011; 6:16789
- Nikeghbalian S, Pournasr B, Aghdami N, Rasekhi A, Geramizadeh B, Hosseini Asl SM, et al. Autologous transplantation of bone marrow-derived mononuclear and CD133(+) cells in patients with decompensated cirrhosis. Arch Iran Med 2011; 14:12 - 17
- Khan AA, Shaik MV, Parveen N, Rajendraprasad A, Aleem MA, Habeeb MA, et al. Human fetal liver-derived stem cell transplantation as supportive modality in the management of end-stage decompensated liver cirrhosis. Cell Transplant 2010; 19:409 - 418
- Mark AL, Sun Z, Warren DS, Lonze BE, Knabel MK, Melville Williams GM, et al. Stem cell mobilization is life saving in an animal model of acute liver failure. Ann Surg 2010; 252:591 - 596
- Wang J, Zhou X, Cui L, Yan L, Liang J, Cheng X, et al. The significance of CD14+ monocytes in peripheral blood stem cells for the treatment of rat liver cirrhosis. Cytotherapy 2010; 12:1022 - 1034
- Yu Y, Lu L, Qian X, Chen N, Yao A, Pu L, et al. Antifibrotic effect of hepatocyte growth factor-expressing mesenchymal stem cells in small-for-size liver transplant rats. Stem Cells Dev 2010; 19:903 - 914