Abstract
Transmissible spongiform encephalopathies (TSEs), or prion diseases, are neurological diseases that can be transmitted through a number of different routes. A wide range of mammalian species are affected by the disease. After peripheral exposure, some TSE agents accumulate in lymphoid tissues at an early stage of disease prior to spreading to the nerves and the brain. Much research has focused on identifying the cells and molecules involved in the transmission of TSE agents from the site of exposure to the brain and several crucial cell types have been associated with this process. The identification of the key cells that influence the different stages of disease transmission might identify targets for therapeutic intervention. This review highlights the involvement of mononuclear phagocytes in TSE disease. Current data suggest these cells may exhibit a diverse range of roles in TSE disease from the transport or destruction of TSE agents in lymphoid tissues, to mediators or protectors of neuropathology in the brain.
Transmissible spongiform encephalopathies (TSEs)
The TSEs are a group of fatal neurodegenerative diseases that affect both humans and animals. These diseases can occur under several different forms: spontaneous, genetic, or acquired through various routes of exposure. Examples of the main disease forms and the species they affect are listed in . TSE disease is characteristically associated with vacuolation in the brain (spongiform pathology), neuronal loss, glial cell activation and amyloid deposits of the disease-associated form of prion protein (PrP), which eventually lead to neurodegeneration and death.
Table 1. TSE diseases
Despite being a neurodegenerative disease, natural transmission often occurs in the periphery before spreading to the central nervous system (CNS). Indeed, in some experimental and natural TSEs, such as natural sheep scrapie, disease is characterized by early agent accumulation in the peripheral lymphoid system.Citation1,Citation2 However, it is worth noting that even within a single species such as the sheep, the strain of the TSE agent or PRNP genotype (which encodes the cellular prion protein PrPc) of the animal can significantly influence disease pathogenesis.Citation3,Citation4 Other forms of the disease, such as bovine spongiform encephalopathy (BSE), appear not to be associated with early TSE agent replication in the peripheral lymphoid tissue of cattle,Citation5 but PrPSc and/or infectivity have been detected in the gut of experimentally and naturally infected cattle.Citation6-Citation10 This difference between TSE agent strain targeting in host tissues is interesting since when BSE transmitted to other species, agent accumulation in the lymphoid tissues is a key feature of variant Creutzfeldt-Jakob disease in humans.Citation11,Citation12
The replication of TSE agents by host cells is critically dependent upon the expression of the host encoded, cellular isoform of the prion protein, PrPc.Citation13 PrPc is an endogenous protein which is expressed on a large range of different cell types throughout the body.Citation14-Citation19 The normal cellular form of PrP has a mainly α-helical structureCitation20 and is protease sensitive. PrPc is a 30–35 kDa glycoprotein which is anchored to the outer layer of the cell membrane through a glycosylphosphatidylinositol (GPI) anchor.Citation21 Although early studies determined that PrPc deficient mice do not appear to be adversely affected by the absence of the protein,Citation22 are not wholly immunodeficient,Citation23 and are developmentally normal,Citation24 recent studies using PrP-deficient mice show, that PrPc is associated with suppression of cognitive function caused by brain-derived amyloid-β in Alzheimer disease,Citation25 as well as blocking pain receptors.Citation26 The protein’s protective function is also associated with epilepsy,Citation27,Citation28 and Martins et al. present the view that as well as playing a role in ‘loss-of-function components’ in prion diseases, PrPc might also be a component in the pathogenesis of other neurodegenerative diseases.Citation28 In addition there has been some speculation about its function in the immune system, for example in mediating pro-survival signals in cells in certain circumstances such as rapid memory cell expansion,Citation23 and a potential role in T lymphocyte activation.Citation15,Citation29,Citation30
TSE diseases are characteristically associated with the accumulation of insoluble aggregates of the disease-specific abnormal form of PrP within the CNS and, in some cases, within the lymphoreticular system (LRS). This abnormal form of the protein is a relatively protease resistant, predominantly β-pleated sheet isoform, termed PrPSc.Citation20 Both PrPc and PrPSc are associated with three different isoforms that are glycosylated at zero, one, or both of the protein’s two possible glycosylation sites, situated at residues 181 and 197 of human PrP, and residues 180 and 196 in murine PrP.Citation31 According to the ‘prion hypothesis’,Citation32 PrPSc is the infectious agent in TSE disease and it is this protein that causes PrPc to convert to the disease associated isoform. Quaking-induced conversion (QUIC)Citation33 and protein misfolding cyclic amplification (PMCA)Citation34 are methods which allow for the amplification of protein, in a similar manner to DNA amplification by polymerase chain reaction (PCR).Citation35 These techniques have enabled propagation of infectious PrP using infected brain-derived or recombinant PrP. As well as studying PrP conversion, these techniques have enabled the detection of infectious TSE agents in various tissues at dilutions previously undetected by other methods. This had only been performed in the presence of additional cofactors such as nucleic acids and lipids, but recently, infectious prions were generated by PMCA from recombinant hamster prion protein without any apparent cofactors.Citation36 Data derived from the use of these two techniques lend further weight to the argument of a protein hypothesis in disease transmission.Citation36
An important question that still surrounds these diseases is how the TSE agent is transported from the site of infection to the LRS and onwards into the CNS. Much speculation has surrounded the issue of whether the TSE agent is transported via cell-associated or cell-free mechanisms. Studies in certain species show that when LRS involvement occurs during the early stages of TSE infection, PrPSc accumulates first on PrPc-expressing follicular dendritic cells (FDCs).Citation4,Citation37-Citation41 FDCs are stromal-derived, tissue resident cells, found in the germinal centers of lymphoid follicles.Citation42 These cells express high levels of PrPc on their surface (), and are clearly associated with PrPSc accumulation in peripheral lymphoid tissues (). The FDC has since been identified as an important site of TSE agent accumulation and replication in the LRS.Citation19,Citation43-Citation45 Furthermore, in the absence of FDCs, TSE agent neuroinvasion is impaired and disease susceptibility reduced. FDCs are considered to amplify TSE agents above the threshold required for neuroinvasion.
Figure 1. PrPc is strongly expressed on FDCs in the lymphoid follicles of the spleen. Images taken from mouse spleen immunolabelled with the anti-CD45R (green, A), anti-PrP (1B3) (blue, B), and anti-CD35 (red, C) antibodies. FDCs and CD35-expressing B cells detected with the anti-CD35 specific antibody. B cells detected with the anti-CD45R specific antibody and PrPc expression was detected using the 1B3 polyclonal antibody.Citation126 (D) Merged image of all three antibodies.
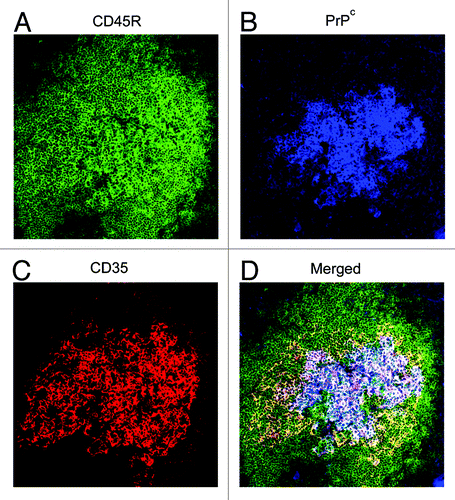
Figure 2. PrPSc accumulates in the draining LN following scrapie infection via the skin. (A) PrPd was detected with the anti-PrP specific antibody 6H4 in the draining inguinal LN five weeks post scrapie infection. (B) Paraffin embedded tissue (PET) blot analysis of adjacent sections confirms PrPd accumulations to be proteinase K resistant PrPSc. (C) Enlarged image of PrPd labeling from boxed area of (A). (D) shows location of FDCs via immostaining with the anti-CD21/CD35 specific antibody. PrPSc is accumulating on FDCs in the LNs.
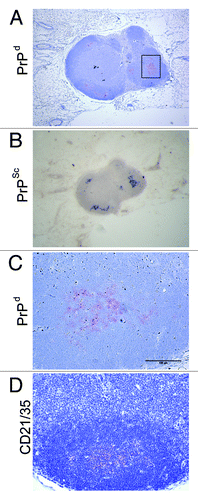
Mononuclear phagocytes (MNPs) and TSE pathogenesis
Even though peripheral lymphoid tissues, and the FDCs within them, have been identified as important sites of TSE agent replication prior to neuroinvasion, little is known of how TSE agents are transported to these sites or what, if any, cells are involved. MNPs are a diverse group of hematopoietically-derived phagocytic cells which includes classical dendritic cells (DCs) and macrophages, but also Langerhans cells (LCs) in the epidermis of the skin and microglia in the brain. The distribution of these cells at the body’s surfaces (intestine, skin, mucosa, etc.) and their ability to phagocytose antigens and deliver them to draining lymphoid tissues, suggested MNPs may either destroy TSE agents or transport them within the host.
The precise ontogeny of classical DCs and macrophages is the subject of much debate. Indeed, there is growing evidence that these two cells types are not as distinct from each other as was once thought.Citation46-Citation48 These cells are often identified or isolated based solely on the expression of a small set of cell surface markers, such as CD11c/Itgax for DCs,Citation49 and CD207/langerin for LCs.Citation50-Citation53 Even under the traditional ‘umbrella terms’ of DCs and macrophages more distinct cellular subsets have been described based on the expression of a long list of different markers. However, the expression of these and other markers has been shown to be less specific than was originally considered.Citation46,Citation54 For example, the temporary depletion of CD11c+ cells in CD11c-DTR transgenic mice, originally considered to specifically deplete DCs,Citation55 has been shown to also deplete many other macrophage subsets, such as the CD169+ macrophages in the spleen and lymph nodes (LN) ().Citation46,Citation54 Much of the data described in this review was generated from in vitro experiments utilizing bone marrow-derived DCs. However, the recent meta-analysis of a large collection of gene expression data from a range of mouse leukocyte lineages shows bone marrow-DCs were clearly identified as phagocytes (macrophages) and were transcriptionally distinct from tissue classical DCs. Indeed, there are few mRNA markers that clearly distinguish classical DCs from macrophages other than low expression of those required for phagocytosis.Citation48 Clearly, without also testing their biological properties (phagocytosis, ability to stimulate naïve T cells etc.) it is difficult to accurately distinguish between classical DCs and macrophages based solely on the expression of a limited set of surfaces markers. This is especially true in immunohistochemistry-based studies. While the authors considered it important to highlight the ambiguity surrounding the discrimination of individual MNP populations, we stick to the traditional terms of macrophages or DCs within this review to avoid further confusion!
Figure 3. Depletion of DCs and macrophages in the spleen of the CD11c-DTR mouse. (B and D) CD11c+ DCs are partially depleted in this transgenic mouse line through the injection of Diphtheria toxin. CD169+ macrophages in the spleen are also depleted through their low level expression of CD11c. (A and C) Normal expression of CD11c and CD169 in control mice. (C and D) are higher magnification images of (A and B).
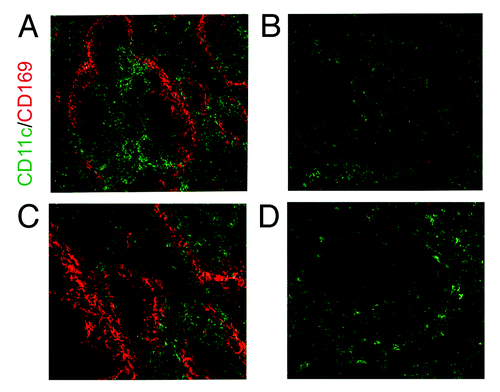
Classical DCs: Taxis for TSE agents?
Haematopoietic, classical DCs are a distinct cell type from mesenchymal-derived FDCs.Citation42 Classical DCs are antigen presenting cells (APCs) and as such they sample their natural environment for foreign antigens, which they process and transport to the nearest lymphoid tissues to initiate a specific immune response. Classical DCs take up antigen through endocytosis or sometimes macropinocytosis.Citation56 Classical DCs travel via the lymphatic system to the secondary lymphoid organs in response to chemokine stimulation where they present antigen to lymphocytes.Citation57 Although the primary role of DCs is to present antigens to T-cells, they are also capable of presenting them to B-cells. This sometimes occurs in the form of intact antigen, in contrast to the processed antigen presented to T-cells.Citation58 These characteristics therefore identify classical DCs as possible candidates for the transport of the intact TSE agents from the site of exposure to the peripheral lymphoid system. Indeed, studies show the retention of PrPSc within bone marrow derived DCs 72 h after in vitro exposure to PrPSc-enriched scrapie associated fibrils.Citation59 However, another study has also suggested that bone marrow-derived DCs rapidly degrade PrPSc,Citation60,Citation61 but as we described above, these cells are highly phagocytic when compared with classical DC from tissues.
Classical DCs could influence TSE pathogenesis at a variety of stages in the disease process: transport from the site of infection to the LRS in the case of LRS involvement; transport between cells within the lymphoid tissues; or the transfer between the lymphoid tissues and the nervous system (neuroinvasion). The argument for a DC-related role in TSE pathogenesis is strengthened by the evidence that the prion protein fragment 106–126 functions as a chemoattractant to immature monocyte-derived DCs.Citation62 Evidence for a possible role of DCs in the delivery of TSE agents to lymphoid tissues was provided by using a transgenic mouse model in which CD11c+ cellsCitation63 or CD8+CD11c+ 64 cells were significantly reduced. The absence of these cells at the time of intraperitoneal scrapie infection significantly prolonged the incubation period of the disease when compared with wild type controls.Citation63,Citation64
DCs in the gut
The oral route is considered a major route of exposure for many naturally acquired TSEs such as natural sheep scrapie, BSE, chronic wasting disease in cervids and variant CJD (vCJD) in man. Studies of experimental transmissions in mice and sheep naturally affected with scrapie show TSE agents accumulate first in the gut-associated lymphoid tissue (GALT) such as the Peyer’s patches and mesenteric LN.Citation4 The accumulation of TSE agents upon FDCs within the GALT is crucial for the efficient spread of disease to the brain.Citation37,Citation40 Studies have also investigated the role of DCs in the transport of the TSE agent from the intestinal lumen to the GALT.Citation59,Citation65 Huang et al.Citation59 used thoracic duct canulation to collect the cells and lymph draining the intestines of rats intra-intestinally injected with PrPSc. Data from this study showed that intestinal DCs could acquire and transport PrPSc from the intestinal lumen, to the mesenteric LN, via the lymph, within hours of intestinal exposure. Furthermore, using CD11c-DTR transgenic mice in which CD11c+ cells can be specifically depleted,Citation55 it was shown that an absence of CD11c+ cells at the time of oral infection blocked the early TSE agent accumulation in the GALT and spleen. Additionally, the overall susceptibility to scrapie disease was reduced in mice where the CD11c+ cells were depleted prior to scrapie challenge. These data imply that CD11c+ cells play a crucial role in the transport of the scrapie agent from the intestinal lumen to the GALT. Whether these data indicate a role for classical DCs or macrophages remains to be determined, since recent analysis of the CD11c-DTR mouse shows that all the MNPs within the gut lamina propria are depleted in this model.Citation46 The migration of MNPs, to and within lymphoid tissues, is regulated by the expression of various chemokines. However, the dissemination of TSE agents from the intestine to the GALT is not dependent on cell migration through the chemokine/receptor system CCL19/CCL21/CCR7.Citation66
Skin and musosa
TSE agents can be readily transmitted via lesions to the skinCitation67-Citation70 and oral mucosa.Citation71-Citation73 Thus, natural TSE infections might also occur via lesions in the mouth and gastrointestinal tract through consumption of rough feed or birth-associated lesions to the skin or mucus membranes. Furthermore, TSE agent infectivity has also been identified in the skin,Citation74-Citation76 as well as in antler velvet.Citation77
The skin provides a first line of defense against infection and is therefore the primary site of residence for a number of MNPs that help to maintain this barrier. These MNPs include LCs in the epidermis and classical dendritic cells and macrophages in the dermis (&5). Classical DCs have been suggested to transport viruses such as HIVCitation78 or DengueCitation79 from the skin, which raises the question whether skin-derived MNPs also play a role in the uptake and transport of the TSE agent from the skin to the LRS.
Figure 4. Detection of LCs and langerin+ dermal DCs in the mouse ear following immunofluorescent labeling of epidermal and dermal sheets. (A) LCs form a dense network of cells within the epidermis. (B) Langerin+ dermal DCs are not abundant within the dermis as the LCs in the epidermis. A small number of these cells may also be migrating LCs.
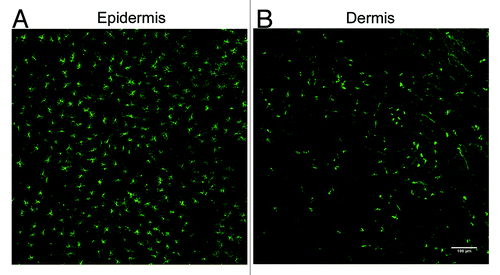
Following scrapie infection via skin scarification in the mouse, agent infectivity and PrPSc accumulation occurs first in the skin draining LN soon after exposure.Citation67,Citation69,Citation80 Experimental scrapie transmission in sheep via the skin failed to find a conclusive link between DCs and the transport of PrPSc.Citation81 However, neutrophils were found to associate with PrPSc. The definite involvement of skin resident MNPs in TSE agent transmission from the skin remains to be determined. Early research in mice also implied the lack of LC involvement.Citation69,Citation82 However, the use of transgenic mouse models that allow for the temporary depletion of LCs, DCs or macrophagesCitation55,Citation83 prior to scrapie infection will help determine whether these cells play a role in TSE transmission from the skin as suggested after oral exposure. Any research looking into the role of skin DCs or macrophages in TSE transmission needs to be put into context with the LC paradigm. Substantial heterogeneity has been revealed between MNP subtypes in different inbred mouse stains.Citation84 For example, LC density in the epidermis is much lower in C57BL/6 mice when compared with BALB/c, 129/Sv, and CBA mice. Such differences could significantly contribute to the differing results observed in many host species exposed to TSE agents.
In 2007 an important novel cell type was identified in the mouse dermis: the langerin+ dermal DC ().Citation85-Citation87 All dermal DCs were thought to be negative for langerin expression, a marker previously considered to be exclusive to LCs. These langerin+ dermal DCs have a much higher turnover rate than LCs and repopulate the skin several weeks faster than the epidermal LCs following depletion.Citation83,Citation88,Citation89 Upon activation, for example by skin immunization, these langerin+ dermal DCs migrate from the skin to the draining LNs much earlier than the LCs.Citation83 Thus, earlier studies addressing the role of LCs would not have been able to factor in the presence of this novel cell type which may have often skewed the interpretation of data in previous studies of LC immunobiology.
Since the identification of langerin+ dermal DCs, a number of publications have questioned the role of LCs in skin immunity, in particular the suggestion that they are the only APCs of the skin. Indeed, langerin+ dermal DCs have been shown to be fully capable of cross-presenting antigens regardless of whether LCs are present or not.Citation90 Experiments where LC migration from the epidermis was blocked, in CD40L−/− mice or via caspase-1 inhibition, were previously used to determine whether LCs played a role in the transport of the scrapie agent from the skin to the draining LNs.Citation69 While the early accumulation of TSE agent infectivity was not affected in the draining LNs, the incubation period of disease was significantly shortened in the CD40L−/− mice. These data implied that instead of aiding pathogenesis by transporting TSE agents from the skin, LCs may impede pathogenesis by phagocytosing and degrading them.Citation69,Citation82 In light of recent findings, further experiments are necessary to distinguish the influence of LCs and langerin+ dermal DC in this process.
Classical DCs as mediators of neuroinvasion
As well as being involved in the transport of the TSE agent from the periphery to the LRS, classical DCs may have a role in the act of neuroinvasion within the lymphoid tissues, for example, the transport of TSE agents to peripheral nerves. Studies show evidence of the intercellular transport of PrPSc through tunnelling nanotubules from DCs to cultured neuronal cells.Citation91 In some TSE diseases where there appears to be little or no LRS involvement (such as BSE in cattle, or sheep of certain PRNP genotypes infected with scrapie) neuroinvasion occurs by an unidentified process. Whether classical DCs fulfil this role as implied in the Gousset studyCitation91 is uncertain. However, studies performed in scrapie infected, FDC-deficient, TNFR1−/− mice, determined that MNPs were unlikely to have directly infected peripheral nerves within lymphoid tissues, as these mice failed to develop disease when injected with scrapie-infected CD11c+ cells (classical DCs).Citation92 These results were in contrast to previous studies in FDC-deficient RAG−/− mice, where scrapie disease was successfully transmitted.Citation93 The discrepancies between these two studies may possibly be due to much higher levels of innervation in the RAG−/− mouse spleens when compared with those from TNFR1−/− mice.Citation92
Following replication within lymphoid tissues, TSE agents are considered to spread to the brain via peripheral sympathetic nerves.Citation94 However, it is plausible that due to their migratory nature, classical DCs carry TSE agents into the CNS across the blood-brain-barrier. Whether the immigration of TSE agent-contaminated classical DCs or circulatory monocytes into the CNS plays an important role in neuroinvasion is uncertain, as significant monocytic infiltration into the brain during TSE disease has not been reported. In sheep infected with scrapie, PrPd accumulation within specific structures in the brain, the circumventricular organs, was an early and consistent feature.Citation95 These sites have fenestrated capillaries, and as such are sites of molecular exchange between the CNS and the blood-stream. However, the lack of monocytic cells within these organs throughout TSE disease is consistent with the hypothesis that cell-associated hematogenous spread is not a major route of neuroinvasion.
Macrophages: Saints or sinners?
Macrophages typically phagocytose and degrade protein antigens more rapidly than classical DCs,Citation96,Citation97 which can retain some protein antigens in their native form up to 36 h after exposure.Citation98 Data from in vitro studies likewise suggest that macrophages also phagocytose and degrade TSE agents including scrapie and BSE.Citation99-Citation101 Furthermore, the depletion of macrophages before scrapie infection increased the accumulation of the scrapie agent in the spleen and accelerated disease pathogenesis.Citation102 Within the macrophage, PrPSc was also found to colocalize with lysosomal and proteasomal proteins,Citation103 implying that, consistent with their biological characteristics, macrophages might play a preventative role in TSE disease when compared with classical DCs. However, data also imply that macrophages might transport orally acquired TSE agents within the GALT.Citation104 Whether the cells described are macrophages or classical DCs is uncertain. As highlighted earlier, it is difficult to classify these cells by immunohistochemistry based on the expression of cell surface markers alone.
Tingible body macrophages are found in close association with FDCs within the germinal centers of follicles within lymphoid tissues, where they clear proteins and apoptotic lymphocytes (tingible bodies).Citation105 Data from ultrastructural studies show high levels of PrPSc within tingible body macrophages in lymphoid tissues of scrapie-affected mice and sheep.Citation106-Citation108 Deposition of PrPd within tingible body macrophages in lymphoid tissues of patients with vCJD have also been described.Citation109 Recent data suggests tingible body macrophages scavenge and degrade PrPSc following synthesis on other infected cells such as FDCs.Citation45
Microglia are the macrophages of the CNS. Gliosis, involving astrocytes and microglia, is one of the neuropathological characteristics of terminal TSE disease. Microglia are the main source of the inflammatory response that is associated with TSE disease, and microglial activation is directly linked to the patterns of PrPSc deposition in the brain, and precedes neuronal cell death.Citation110,Citation111 Significant vacuolar degeneration has been detected in the microglia/macrophages of two vCJD patients.Citation112 Microglia and astrocytes have been associated with granular PrPd deposits, leading speculation that these cells play a role in processing, degrading, or removing PrPSc.Citation113 The in vitro exposure of microglia to murine scrapie brain homogenate or PrP(106–126) severely affected their phagocytic activity.Citation114 In contrast, microglia from ME7-scrapie affected mice were capable of phagocytosis, but were not able to clear PrPSc.Citation115 Within TSE-affected brains the pro-inflammatory activity of microglia is specifically modulated by the anti-inflammatory cytokine TGFβ1. This cytokine appears to play a critical role in the downregulation of pro-inflammatory microglial responses minimizing brain inflammation and thus avoiding exacerbation of brain damage.Citation116 Whether systemic infections and inflammation lead to the dis-regulation of this control and the exacerbation of neurodegeneration remains to be determined.Citation117
A recently identified brain DC population, morphologically similar to microglia is considered to be a new member of the heterogeneous microglia population.Citation118 However, it is uncertain whether these cells are a unique subset of classical DCs. These data indicate that, as in the other tissues discussed in this review, there are a number of different MNP populations that could influence TSE pathogenesis in the brain, depending on the circumstances. Furthermore, some data might relate to a particular subset of cells, rather than to the entirety of MNPs within the brain.
Subcapsular sinus macrophages are a distinct, poorly endocytic and degradative macrophage subset.Citation119 These unique cells capture antigen-containing immune complexes arriving in the LN via their cell processes that they extend into the lumen of the subcapsular sinus.Citation119-Citation123 In contrast to other macrophage subsets, subcapsular sinus macrophages retain immune complexes on their surfaces for rapid translocation through the floor of the subcapsular sinus to underlying, non-cognate (non-specific) follicular B cells.Citation119 These B cells then acquire the immune complexes via their complement receptors and deliver them to FDCs. The higher immune complex-binding affinities of FDCs most likely relieve the B cells of their cargo. Thus, the subcapsular sinus macrophage-B cell immune complex relay represents an efficient route through which antigens are delivered to FDCsCitation119-Citation123 (). The demonstration that subcapsular sinus macrophages in LNs (and their counterparts in the spleen) play a key role in the delivery of complement-bound immune complexes to FDCs raises the possibility that these cells might also play an important role in the transport of complement-opsonized TSE agents to FDCs within lymphoid tissues. Indeed, disease-specific PrP has been detected within the subcapsular sinus macrophages of intestinally-exposed sheep.Citation124
Figure 6. Schematic representation of the role of subcapsullar sinus macrophages in the transport of immune complexes to FDC in the LN. (1) Subcapsullar sinus macrophages capture lymph borne immune complexes in the subcapsullar sinus which they transcytose intact across their surfaces to underlying follicular B cells. (2) Non-cognate B cells acquire the immune complexes via their complement receptors and (3) deliver them to FDCs. (4) Cognate (antigen-specific) B cells, in contrast, acquire antigen-containing immune complexes via their B-cell receptors, become activated and (5) migrate to the boundary of the T-cell zone.
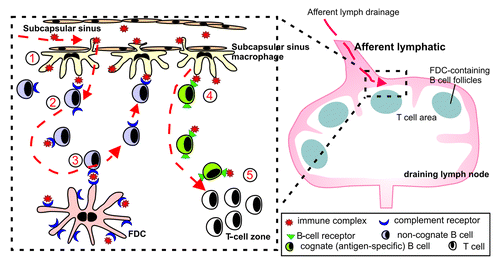
Concluding remarks
This review aimed to highlight the potential involvement of the various MNP populations in TSE pathogenesis. Current data suggest MNPs may exhibit a diverse range of roles in TSE disease from the transport or destruction of TSE agents in lymphoid tissues, to mediators or protectors of neuropathology in the brain. Much of the research described above studies the influence of MNPs on TSE pathogenesis during steady-state conditions. However, under inflammatory conditions MNPs may exacerbate TSE pathogenesis, for example through the release of neurotoxic mediators in the brainCitation117 or the transmission of disease between individuals through the contamination of bodily secretions such as milk.Citation125 While some MNPs may play important roles in TSE pathogenesis, it is equally likely that in some circumstances their involvement is minimal. The route of TSE infection, strain of TSE agent and host species may all influence the role of MNPs in disease pathogenesis. Indeed, it is equally probable that in some instances TSE agents reach the draining lymphoid tissues via a cell-free mechanism such as in a complement-bound immune complex or via the conduit system.Citation123 Recent advances in immunology have identified a long list of new members of the MNP phagocyte system, which may also have an important impact on TSE pathogenesis. Continuing research with the new tools and models available will help to unravel the mysteries that still surround TSE pathogenesis. AUTHOR: Please cite in the text.
Figure 5. Schematic representation of the MNPs in the skin of the mouse. LCs can be found in the epidermis. The dermis is situated below the epidermis and comprises langerin+ as well as langerin- DCs and various macrophage populations. All these cell types migrate from the skin to the draining LN, and therefore may play a potential role in the transport of the TSE agent from the skin to the draining LN, where PrPSc accumulates following infection via the skin.
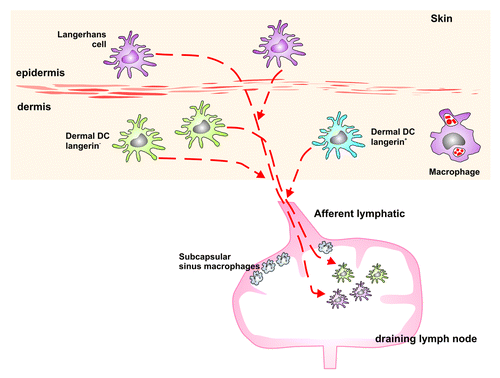
Abbreviations: | ||
BSE | = | bovine spongiform encephalopathy |
CNS | = | central nervous system |
DC | = | dendritic cell |
FDC | = | follicular dendritic cell |
GALT | = | gut-associated lymphoid tissue |
LC | = | Langerhans cell |
LN | = | lymph node |
LRS | = | lympho-reticular system |
MNP | = | mononuclear phagocyte |
PP | = | Peyer’s patch |
PrP | = | Prion protein |
TSE | = | transmissible spongiform encephalopathy |
Acknowledgments
The authors wish to thank Bob Fleming and the Pathology Services Group (The Roslin Institute and R(D)SVS, University of Edinburgh, UK) for excellent technical support. This work was supported by project and Institute Strategic Programme Grant funding from the Biotechnology and Biological Sciences Research Council.
Disclosure of Potential Conflicts of Interest
The authors declare no financial conflict of interest.
References
- Bruce ME, Brown KL, Mabbott NA, Farquhar CF, Jeffrey M. Follicular dendritic cells in TSE pathogenesis. Immunol Today 2000; 21:442 - 6; http://dx.doi.org/10.1016/S0167-5699(00)01696-0; PMID: 10953096
- Brown KL, Ritchie DL, McBride PA, Bruce ME. Detection of PrP in extraneural tissues. Microsc Res Tech 2000; 50:40 - 5; http://dx.doi.org/10.1002/1097-0029(20000701)50:1<40::AID-JEMT7>3.0.CO;2-M; PMID: 10871547
- Heggebø R, Press CM, Gunnes G, González L, Jeffrey M. Distribution and accumulation of PrP in gut-associated and peripheral lymphoid tissue of scrapie-affected Suffolk sheep. J Gen Virol 2002; 83:479 - 89; PMID: 11807242
- Andréoletti O, Berthon P, Marc D, Sarradin P, Grosclaude J, van Keulen L, et al. Early accumulation of PrP(Sc) in gut-associated lymphoid and nervous tissues of susceptible sheep from a Romanov flock with natural scrapie. J Gen Virol 2000; 81:3115 - 26; PMID: 11086143
- Somerville RA, Birkett CR, Farquhar CF, Hunter N, Goldmann W, Dornan J, et al. Immunodetection of PrPSc in spleens of some scrapie-infected sheep but not BSE-infected cows. J Gen Virol 1997; 78:2389 - 96; PMID: 9292029
- Buschmann A, Groschup MH. Highly bovine spongiform encephalopathy-sensitive transgenic mice confirm the essential restriction of infectivity to the nervous system in clinically diseased cattle. J Infect Dis 2005; 192:934 - 42; http://dx.doi.org/10.1086/431602; PMID: 16088845
- Hoffmann C, Eiden M, Kaatz M, Keller M, Ziegler U, Rogers R, et al. BSE infectivity in jejunum, ileum and ileocaecal junction of incubating cattle. Vet Res 2011; 42:21; http://dx.doi.org/10.1186/1297-9716-42-21; PMID: 21314904
- Iwata N, Sato Y, Higuchi Y, Nohtomi K, Nagata N, Hasegawa H, et al. Distribution of PrP(Sc) in cattle with bovine spongiform encephalopathy slaughtered at abattoirs in Japan. Jpn J Infect Dis 2006; 59:100 - 7; PMID: 16632909
- Terry LA, Marsh S, Ryder SJ, Hawkins SAC, Wells GAH, Spencer YI. Detection of disease-specific PrP in the distal ileum of cattle exposed orally to the agent of bovine spongiform encephalopathy. Vet Rec 2003; 152:387 - 92; http://dx.doi.org/10.1136/vr.152.13.387; PMID: 12696704
- Wells GA, Dawson M, Hawkins SA, Green RB, Dexter I, Francis ME, et al. Infectivity in the ileum of cattle challenged orally with bovine spongiform encephalopathy. Vet Rec 1994; 135:40 - 1; http://dx.doi.org/10.1136/vr.135.2.40; PMID: 7975074
- Foster JD, Parnham DW, Hunter N, Bruce M. Distribution of the prion protein in sheep terminally affected with BSE following experimental oral transmission. J Gen Virol 2001; 82:2319 - 26; PMID: 11562525
- Hill AF, Zeidler M, Ironside J, Collinge J. Diagnosis of new variant Creutzfeldt-Jakob disease by tonsil biopsy. Lancet 1997; 349:99 - 100; http://dx.doi.org/10.1016/S0140-6736(97)24002-X; PMID: 8996424
- Büeler H, Aguzzi A, Sailer A, Greiner RA, Autenried P, Aguet M, et al. Mice devoid of PrP are resistant to scrapie. Cell 1993; 73:1339 - 47; http://dx.doi.org/10.1016/0092-8674(93)90360-3; PMID: 8100741
- Burthem J, Urban B, Pain A, Roberts DJ. The normal cellular prion protein is strongly expressed by myeloid dendritic cells. Blood 2001; 98:3733 - 8; http://dx.doi.org/10.1182/blood.V98.13.3733; PMID: 11739179
- Mabbott NA, Brown KL, Manson J, Bruce ME. T-lymphocyte activation and the cellular form of the prion protein. Immunology 1997; 92:161 - 5; http://dx.doi.org/10.1046/j.1365-2567.1997.00331.x; PMID: 9415021
- Mabbott NA, Farquhar CF, Brown KL, Bruce ME. Involvement of the immune system in TSE pathogenesis. Immunol Today 1998; 19:201 - 3; http://dx.doi.org/10.1016/S0167-5699(98)01253-5; PMID: 9613034
- Sugaya M, Nakamura K, Watanabe T, Asahina A, Yasaka N, Koyama Y-i, et al. Expression of cellular prion-related protein by murine Langerhans cells and keratinocytes. J Dermatol Sci 2002; 28:126 - 34; http://dx.doi.org/10.1016/S0923-1811(01)00160-8; PMID: 11858951
- Horiuchi M, Yamazaki N, Ikeda T, Ishiguro N, Shinagawa M. A cellular form of prion protein (PrPC) exists in many non-neuronal tissues of sheep. J Gen Virol 1995; 76:2583 - 7; http://dx.doi.org/10.1099/0022-1317-76-10-2583; PMID: 7595362
- Brown KL, Stewart K, Ritchie DL, Mabbott NA, Williams A, Fraser H, et al. Scrapie replication in lymphoid tissues depends on prion protein-expressing follicular dendritic cells. Nat Med 1999; 5:1308 - 12; http://dx.doi.org/10.1038/15264; PMID: 10545999
- Pan KM, Baldwin M, Nguyen J, Gasset M, Serban A, Groth D, et al. Conversion of alpha-helices into beta-sheets features in the formation of the scrapie prion proteins. Proc Natl Acad Sci U S A 1993; 90:10962 - 6; http://dx.doi.org/10.1073/pnas.90.23.10962; PMID: 7902575
- Stahl N, Borchelt DR, Hsiao K, Prusiner SB. Scrapie prion protein contains a phosphatidylinositol glycolipid. Cell 1987; 51:229 - 40; http://dx.doi.org/10.1016/0092-8674(87)90150-4; PMID: 2444340
- Büeler H, Fischer M, Lang Y, Bluethmann H, Lipp H-P, DeArmond SJ, et al. Normal development and behaviour of mice lacking the neuronal cell-surface PrP protein. Nature 1992; 356:577 - 82; http://dx.doi.org/10.1038/356577a0; PMID: 1373228
- Isaacs JD, Jackson GS, Altmann DM. The role of the cellular prion protein in the immune system. Clin Exp Immunol 2006; 146:1 - 8; http://dx.doi.org/10.1111/j.1365-2249.2006.03194.x; PMID: 16968391
- Manson JC, Clarke AR, Hooper ML, Aitchison L, McConnell I, Hope J. 129/Ola mice carrying a null mutation in PrP that abolishes mRNA production are developmentally normal. Mol Neurobiol 1994; 8:121 - 7; http://dx.doi.org/10.1007/BF02780662; PMID: 7999308
- Gimbel DA, Nygaard HB, Coffey EE, Gunther EC, Laurén J, Gimbel ZA, et al. Memory impairment in transgenic Alzheimer mice requires cellular prion protein. J Neurosci 2010; 30:6367 - 74; http://dx.doi.org/10.1523/JNEUROSCI.0395-10.2010; PMID: 20445063
- Gadotti VM, Zamponi GW. Cellular prion protein protects from inflammatory and neuropathic pain. Mol Pain 2011; 7:59; http://dx.doi.org/10.1186/1744-8069-7-59; PMID: 21843375
- Walz R, Amaral OB, Rockenbach IC, Roesler R, Izquierdo I, Cavalheiro EA, et al. Increased sensitivity to seizures in mice lacking cellular prion protein. Epilepsia 1999; 40:1679 - 82; http://dx.doi.org/10.1111/j.1528-1157.1999.tb01583.x; PMID: 10612329
- Martins VR, Beraldo FH, Hajj GN, Lopes MH, Lee KS, Prado MM, et al. Prion protein: orchestrating neurotrophic activities. Curr Issues Mol Biol 2010; 12:63 - 86; PMID: 19767651
- Li R, Liu D, Zanusso G, Liu T, Fayen JD, Huang J-H, et al. The expression and potential function of cellular prion protein in human lymphocytes. Cell Immunol 2001; 207:49 - 58; http://dx.doi.org/10.1006/cimm.2000.1751; PMID: 11161453
- Mattei V, Garofalo T, Misasi R, Circella A, Manganelli V, Lucania G, et al. Prion protein is a component of the multimolecular signaling complex involved in T cell activation. FEBS Lett 2004; 560:14 - 8; http://dx.doi.org/10.1016/S0014-5793(04)00029-8; PMID: 14987990
- Lawson VA, Collins SJ, Masters CL, Hill AF. Prion protein glycosylation. J Neurochem 2005; 93:793 - 801; http://dx.doi.org/10.1111/j.1471-4159.2005.03104.x; PMID: 15857383
- Prusiner SB. Novel proteinaceous infectious particles cause scrapie. Science 1982; 216:136 - 44; http://dx.doi.org/10.1126/science.6801762; PMID: 6801762
- Atarashi R, Wilham JM, Christensen L, Hughson AG, Moore RA, Johnson LM, et al. Simplified ultrasensitive prion detection by recombinant PrP conversion with shaking. Nat Methods 2008; 5:211 - 2; http://dx.doi.org/10.1038/nmeth0308-211; PMID: 18309304
- Saborio GP, Permanne B, Soto C. Sensitive detection of pathological prion protein by cyclic amplification of protein misfolding. Nature 2001; 411:810 - 3; http://dx.doi.org/10.1038/35081095; PMID: 11459061
- Soto C, Saborio GP, Anderes L. Cyclic amplification of protein misfolding: application to prion-related disorders and beyond. Trends Neurosci 2002; 25:390 - 4; http://dx.doi.org/10.1016/S0166-2236(02)02195-1; PMID: 12127750
- Kim J-I, Cali I, Surewicz K, Kong Q, Raymond GJ, Atarashi R, et al. Mammalian prions generated from bacterially expressed prion protein in the absence of any mammalian cofactors. J Biol Chem 2010; 285:14083 - 7; http://dx.doi.org/10.1074/jbc.C110.113464; PMID: 20304915
- Glaysher BR, Mabbott NA. Role of the GALT in scrapie agent neuroinvasion from the intestine. J Immunol 2007; 178:3757 - 66; PMID: 17339474
- Hilton DA, Fathers E, Edwards P, Ironside JW, Zajicek J. Prion immunoreactivity in appendix before clinical onset of variant Creutzfeldt-Jakob disease. Lancet 1998; 352:703 - 4; http://dx.doi.org/10.1016/S0140-6736(98)24035-9; PMID: 9728989
- Mabbott NA, Young J, McConnell I, Bruce ME. Follicular dendritic cell dedifferentiation by treatment with an inhibitor of the lymphotoxin pathway dramatically reduces scrapie susceptibility. J Virol 2003; 77:6845 - 54; http://dx.doi.org/10.1128/JVI.77.12.6845-6854.2003; PMID: 12768004
- Prinz M, Huber G, Macpherson AJS, Heppner FL, Glatzel M, Eugster H-P, et al. Oral prion infection requires normal numbers of Peyer’s patches but not of enteric lymphocytes. Am J Pathol 2003; 162:1103 - 11; http://dx.doi.org/10.1016/S0002-9440(10)63907-7; PMID: 12651603
- Sigurdson CJ, Williams ES, Miller MW, Spraker TR, O’Rourke KI, Hoover EA. Oral transmission and early lymphoid tropism of chronic wasting disease PrPres in mule deer fawns (Odocoileus hemionus). J Gen Virol 1999; 80:2757 - 64; PMID: 10573172
- Mabbott NA, Kenneth Baillie J, Kobayashi A, Donaldson DS, Ohmori H, Yoon S-O, et al. Expression of mesenchyme-specific gene signatures by follicular dendritic cells: insights from the meta-analysis of microarray data from multiple mouse cell populations. Immunology 2011; 133:482 - 98; http://dx.doi.org/10.1111/j.1365-2567.2011.03461.x; PMID: 21635249
- Mabbott NA, Mackay F, Minns F, Bruce ME. Temporary inactivation of follicular dendritic cells delays neuroinvasion of scrapie. Nat Med 2000; 6:719 - 20; http://dx.doi.org/10.1038/77401; PMID: 10888894
- Montrasio F, Frigg R, Glatzel M, Klein MA, Mackay F, Aguzzi A, et al. Impaired prion replication in spleens of mice lacking functional follicular dendritic cells. Science 2000; 288:1257 - 9; http://dx.doi.org/10.1126/science.288.5469.1257; PMID: 10818004
- McCulloch L, Brown KL, Bradford BM, Hopkins J, Bailey M, Rajewsky K, et al. Follicular dendritic cell-specific prion protein (PrP) expression alone is sufficient to sustain prion infection in the spleen. PLoS Pathog 2011; 7:e1002402; http://dx.doi.org/10.1371/journal.ppat.1002402; PMID: 22144895
- Bradford BM, Sester DP, Hume DA, Mabbott NA. Defining the anatomical localisation of subsets of the murine mononuclear phagocyte system using integrin alpha X (Itgax, CD11c) and colony stimulating factor 1 receptor (Csf1r, CD115) expression fails to discriminate dendritic cells from macrophages. Immunobiology 2011; 216:1228 - 37; http://dx.doi.org/10.1016/j.imbio.2011.08.006; PMID: 21885153
- Hume DA. Macrophages as APC and the dendritic cell myth. J Immunol 2008; 181:5829 - 35; PMID: 18941170
- Mabbott NA, Kenneth Baillie J, Hume DA, Freeman TC. Meta-analysis of lineage-specific gene expression signatures in mouse leukocyte populations. Immunobiology 2010; 215:724 - 36; http://dx.doi.org/10.1016/j.imbio.2010.05.012; PMID: 20580463
- Austin JM. Dendritic Cells in Spleen and Lymph Node. In: Lotze MT, Thomson AW, eds. Dendritic Cells Biology and Clinical Applications: Academic Press, 1999:179-204.
- Valladeau J, Duvert-Frances V, Pin J-J, Dezutter-Dambuyant C, Vincent C, Massacrier C, et al. The monoclonal antibody DCGM4 recognizes Langerin, a protein specific of Langerhans cells, and is rapidly internalized from the cell surface. Eur J Immunol 1999; 29:2695 - 704; http://dx.doi.org/10.1002/(SICI)1521-4141(199909)29:09<2695::AID-IMMU2695>3.0.CO;2-Q; PMID: 10508244
- Valladeau J, Ravel O, Dezutter-Dambuyant C, Moore K, Kleijmeer M, Liu Y, et al. Langerin, a novel C-type lectin specific to Langerhans cells, is an endocytic receptor that induces the formation of Birbeck granules. Immunity 2000; 12:71 - 81; http://dx.doi.org/10.1016/S1074-7613(00)80160-0; PMID: 10661407
- Takahara K, Omatsu Y, Yashima Y, Maeda Y, Tanaka S, Iyoda T, et al. Identification and expression of mouse Langerin (CD207) in dendritic cells. Int Immunol 2002; 14:433 - 44; http://dx.doi.org/10.1093/intimm/14.5.433; PMID: 11978773
- Valladeau J, Clair-Moninot V, Dezutter-Dambuyant C, Pin J-J, Kissenpfennig A, Mattéi M-G, et al. Identification of mouse langerin/CD207 in Langerhans cells and some dendritic cells of lymphoid tissues. J Immunol 2002; 168:782 - 92; PMID: 11777972
- Probst HC, Tschannen K, Odermatt B, Schwendener R, Zinkernagel RM, Van Den Broek M. Histological analysis of CD11c-DTR/GFP mice after in vivo depletion of dendritic cells. Clin Exp Immunol 2005; 141:398 - 404; http://dx.doi.org/10.1111/j.1365-2249.2005.02868.x; PMID: 16045728
- Jung S, Unutmaz D, Wong P, Sano G-I, De los Santos K, Sparwasser T, et al. In vivo depletion of CD11c+ dendritic cells abrogates priming of CD8+ T cells by exogenous cell-associated antigens. Immunity 2002; 17:211 - 20; http://dx.doi.org/10.1016/S1074-7613(02)00365-5; PMID: 12196292
- Sallusto F, Cella M, Danieli C. A L. Dendritic cells use macropinocytosis and the mannose receptor to concentrate macromolecules in the major histocompatibility complex class II compartment: downregulation by cytokines and bacterial products. J Exp Med 1995; 182:389 - 400; http://dx.doi.org/10.1084/jem.182.2.389; PMID: 7629494
- Steinman RM. The dendritic cell system and its role in immunogenicity. Annu Rev Immunol 1991; 9:271 - 96; http://dx.doi.org/10.1146/annurev.iy.09.040191.001415; PMID: 1910679
- MacPherson G, Kushnir N, Wykes M. Dendritic cells, B cells and the regulation of antibody synthesis. Immunol Rev 1999; 172:325 - 34; http://dx.doi.org/10.1111/j.1600-065X.1999.tb01376.x; PMID: 10631957
- Huang F-P, Farquhar CF, Mabbott NA, Bruce ME, MacPherson GG. Migrating intestinal dendritic cells transport PrP(Sc) from the gut. J Gen Virol 2002; 83:267 - 71; PMID: 11752724
- Luhr KM, Wallin RPA, Ljunggren H-G, Löw P, Taraboulos A, Kristensson K. Processing and degradation of exogenous prion protein by CD11c(+) myeloid dendritic cells in vitro. J Virol 2002; 76:12259 - 64; http://dx.doi.org/10.1128/JVI.76.23.12259-12264.2002; PMID: 12414965
- Lai L, Alaverdi N, Maltais L, Morse HC 3rd. Mouse cell surface antigens: nomenclature and immunophenotyping. J Immunol 1998; 160:3861 - 8; PMID: 9558091
- Kaneider NC, Kaser A, Dunzendorfer S, Tilg H, Wiedermann CJ. Sphingosine kinase-dependent migration of immature dendritic cells in response to neurotoxic prion protein fragment. J Virol 2003; 77:5535 - 9; http://dx.doi.org/10.1128/JVI.77.9.5535-5539.2003; PMID: 12692258
- Cordier-Dirikoc S, Chabry J. Temporary depletion of CD11c+ dendritic cells delays lymphoinvasion after intraperitonal scrapie infection. J Virol 2008:JVI.02440-07.
- Sethi S, Kerksiek KM, Brocker T, Kretzschmar H. Role of the CD8+ dendritic cell subset in transmission of prions. J Virol 2007; 81:4877 - 80; http://dx.doi.org/10.1128/JVI.02345-06; PMID: 17301133
- Raymond CR, Aucouturier P, Mabbott NA. In vivo depletion of CD11c+ cells impairs scrapie agent neuroinvasion from the intestine. J Immunol 2007; 179:7758 - 66; PMID: 18025222
- Levavasseur E, Metharom P, Dorban G, Nakano H, Kakiuchi T, Carnaud C, et al. Experimental scrapie in ‘plt’ mice: an assessment of the role of dendritic-cell migration in the pathogenesis of prion diseases. J Gen Virol 2007; 88:2353 - 60; http://dx.doi.org/10.1099/vir.0.82816-0; PMID: 17622642
- Glaysher BR, Mabbott NA. Role of the draining lymph node in scrapie agent transmission from the skin. Immunol Lett 2007; 109:64 - 71; http://dx.doi.org/10.1016/j.imlet.2007.01.003; PMID: 17292972
- Mohan J, Brown KL, Farquhar CF, Bruce ME, Mabbott NA. Scrapie transmission following exposure through the skin is dependent on follicular dendritic cells in lymphoid tissues. J Dermatol Sci 2004; 35:101 - 11; http://dx.doi.org/10.1016/j.jdermsci.2004.05.005; PMID: 15265522
- Mohan J, Bruce ME, Mabbott NA. Neuroinvasion by scrapie following inoculation via the skin is independent of migratory Langerhans cells. J Virol 2005; 79:1888 - 97; http://dx.doi.org/10.1128/JVI.79.3.1888-1897.2005; PMID: 15650212
- Taylor DM, McConnell I, Fraser H. Scrapie infection can be established readily through skin scarification in immunocompetent but not immunodeficient mice. J Gen Virol 1996; 77:1595 - 9; http://dx.doi.org/10.1099/0022-1317-77-7-1595; PMID: 8758004
- Bartz JC, Kincaid AE, Bessen RA. Rapid prion neuroinvasion following tongue infection. J Virol 2003; 77:583 - 91; http://dx.doi.org/10.1128/JVI.77.1.583-591.2003; PMID: 12477862
- Carp RI. Transmission of scrapie by oral route: effect of gingival scarification. Lancet 1982; 1:170 - 1; http://dx.doi.org/10.1016/S0140-6736(82)90421-4; PMID: 6119548
- Denkers ND, Telling GC, Hoover EA. Minor oral lesions facilitate transmission of chronic wasting disease. J Virol 2011; 85:1396 - 9; http://dx.doi.org/10.1128/JVI.01655-10; PMID: 21084472
- Cunningham AA, Kirkwood JK, Dawson M, Spencer YI, Green RB, Wells GAH. Bovine spongiform encephalopathy infectivity in greater kudu (Tragelaphus strepsiceros). Emerg Infect Dis 2004; 10:1044 - 9; PMID: 15207051
- Notari S, Moleres FJ, Hunter SB, Belay ED, Schonberger LB, Cali I, et al. Multiorgan detection and characterization of protease-resistant prion protein in a case of variant CJD examined in the United States. PLoS One 2010; 5:e8765; http://dx.doi.org/10.1371/journal.pone.0008765; PMID: 20098730
- Thomzig A, Schulz-Schaeffer W, Wrede A, Wemheuer W, Brenig B, Kratzel C, et al. Accumulation of pathological prion protein PrPSc in the skin of animals with experimental and natural scrapie. PLoS Pathog 2007; 3:e66; http://dx.doi.org/10.1371/journal.ppat.0030066; PMID: 17530923
- Angers RC, Seward TS, Napier D, Green M, Hoover EA, Spraker T, et al. Chronic wasting disease prions in elk antler velvet. Emerg Infect Dis 2009; 15:696 - 703; http://dx.doi.org/10.3201/eid1505.081458; PMID: 19402954
- de Jong MAWP, Geijtenbeek TBH. Langerhans cells in innate defense against pathogens. Trends Immunol 2010; 31:452 - 9; http://dx.doi.org/10.1016/j.it.2010.08.002; PMID: 21030306
- Wu S-JL, Grouard-Vogel G, Sun W, Mascola JR, Brachtel E, Putvatana R, et al. Human skin Langerhans cells are targets of dengue virus infection. Nat Med 2000; 6:816 - 20; http://dx.doi.org/10.1038/77553; PMID: 10888933
- Mohan J, Bruce ME, Mabbott NA. Follicular dendritic cell dedifferentiation reduces scrapie susceptibility following inoculation via the skin. Immunology 2005; 114:225 - 34; http://dx.doi.org/10.1111/j.1365-2567.2004.02074.x; PMID: 15667567
- Gossner A, Hunter N, Hopkins J. Role of lymph-borne cells in the early stages of scrapie agent dissemination from the skin. Vet Immunol Immunopathol 2006; 109:267 - 78; http://dx.doi.org/10.1016/j.vetimm.2005.08.021; PMID: 16169089
- Mohan J, Hopkins J, Mabbott NA. Skin-derived dendritic cells acquire and degrade the scrapie agent following in vitro exposure. Immunology 2005; 116:122 - 33; http://dx.doi.org/10.1111/j.1365-2567.2005.02207.x; PMID: 16108824
- Kissenpfennig A, Henri S, Dubois B, Laplace-Builhé C, Perrin P, Romani N, et al. Dynamics and function of Langerhans cells in vivo: dermal dendritic cells colonize lymph node areas distinct from slower migrating Langerhans cells. Immunity 2005; 22:643 - 54; http://dx.doi.org/10.1016/j.immuni.2005.04.004; PMID: 15894281
- Flacher V, Douillard P, Aït-Yahia S, Stoitzner P, Clair-Moninot V, Romani N, et al. Expression of langerin/CD207 reveals dendritic cell heterogeneity between inbred mouse strains. Immunology 2008; 123:339 - 47; http://dx.doi.org/10.1111/j.1365-2567.2007.02785.x; PMID: 18217955
- Bursch LS, Wang L, Igyarto B, Kissenpfennig A, Malissen B, Kaplan DH, et al. Identification of a novel population of Langerin+ dendritic cells. J Exp Med 2007; 204:3147 - 56; http://dx.doi.org/10.1084/jem.20071966; PMID: 18086865
- Ginhoux F, Collin MP, Bogunovic M, Abel M, Leboeuf M, Helft J, et al. Blood-derived dermal langerin+ dendritic cells survey the skin in the steady state. J Exp Med 2007; 204:3133 - 46; http://dx.doi.org/10.1084/jem.20071733; PMID: 18086862
- Poulin LF, Henri S, de Bovis B, Devilard E, Kissenpfennig A, Malissen B. The dermis contains langerin+ dendritic cells that develop and function independently of epidermal Langerhans cells. J Exp Med 2007; 204:3119 - 31; http://dx.doi.org/10.1084/jem.20071724; PMID: 18086861
- Bennett CL, van Rijn E, Jung S, Inaba K, Steinman RM, Kapsenberg ML, et al. Inducible ablation of mouse Langerhans cells diminishes but fails to abrogate contact hypersensitivity. J Cell Biol 2005; 169:569 - 76; http://dx.doi.org/10.1083/jcb.200501071; PMID: 15897263
- Nagao K, Ginhoux F, Leitner WW, Motegi SI, Bennett CL, Clausen BE, et al. Murine epidermal Langerhans cells and langerin-expressing dermal dendritic cells are unrelated and exhibit distinct functions. Proc Natl Acad Sci U S A 2009; 106:3312 - 7; http://dx.doi.org/10.1073/pnas.0807126106; PMID: 19218433
- Henri S, Poulin LF, Tamoutounour S, Ardouin L, Guilliams M, de Bovis B, et al. CD207+ CD103+ dermal dendritic cells cross-present keratinocyte-derived antigens irrespective of the presence of Langerhans cells. J Exp Med 2010; 207:189 - 206; http://dx.doi.org/10.1084/jem.20091964; PMID: 20038600
- Gousset K, Schiff E, Langevin C, Marijanovic Z, Caputo A, Browman DT, et al. Prions hijack tunnelling nanotubes for intercellular spread. Nat Cell Biol 2009; 11:328 - 36; http://dx.doi.org/10.1038/ncb1841; PMID: 19198598
- Raymond CR, Mabbott NA. Assessing the involvement of migratory dendritic cells in the transfer of the scrapie agent from the immune to peripheral nervous systems. J Neuroimmunol 2007; 187:114 - 25; http://dx.doi.org/10.1016/j.jneuroim.2007.05.006; PMID: 17561271
- Aucouturier P, Geissmann F, Damotte D, Saborio GP, Meeker HC, Kascsak R, et al. Infected splenic dendritic cells are sufficient for prion transmission to the CNS in mouse scrapie. J Clin Invest 2001; 108:703 - 8; PMID: 11544275
- Glatzel M, Heppner FL, Albers KM, Aguzzi A. Sympathetic innervation of lymphoreticular organs is rate limiting for prion neuroinvasion. Neuron 2001; 31:25 - 34; http://dx.doi.org/10.1016/S0896-6273(01)00331-2; PMID: 11498048
- Sisó S, Jeffrey M, González L. Neuroinvasion in sheep transmissible spongiform encephalopathies: the role of the haematogenous route. Neuropathol Appl Neurobiol 2009; 35:232 - 46; http://dx.doi.org/10.1111/j.1365-2990.2008.00978.x; PMID: 19473292
- Delamarre L, Pack M, Chang H, Mellman I, Trombetta ES. Differential lysosomal proteolysis in antigen-presenting cells determines antigen fate. Science 2005; 307:1630 - 4; http://dx.doi.org/10.1126/science.1108003; PMID: 15761154
- Bergtold A, Desai DD, Gavhane A, Clynes R. Cell surface recycling of internalized antigen permits dendritic cell priming of B cells. Immunity 2005; 23:503 - 14; http://dx.doi.org/10.1016/j.immuni.2005.09.013; PMID: 16286018
- Wykes M, Pombo A, Jenkins C, MacPherson GG. Dendritic cells interact directly with naive B lymphocytes to transfer antigen and initiate class switching in a primary T-dependent response. J Immunol 1998; 161:1313 - 9; PMID: 9686593
- Carp RI, Callahan SM. In vitro interaction of scrapie agent and mouse peritoneal macrophages. Intervirology 1981; 16:8 - 13; http://dx.doi.org/10.1159/000149241; PMID: 6799420
- Carp RI, Callahan SM. Effect of mouse peritoneal macrophages on scrapie infectivity during extended in vitro incubation. Intervirology 1982; 17:201 - 7; http://dx.doi.org/10.1159/000149289; PMID: 6813286
- Sassa Y, Inoshima Y, Ishiguro N. Bovine macrophage degradation of scrapie and BSE PrPSc. Vet Immunol Immunopathol 2010; 133:33 - 9; http://dx.doi.org/10.1016/j.vetimm.2009.06.018; PMID: 19647878
- Beringue V, Demoy M, Lasmézas CI, Gouritin B, Weingarten C, Deslys J-P, et al. Role of spleen macrophages in the clearance of scrapie agent early in pathogenesis. J Pathol 2000; 190:495 - 502; http://dx.doi.org/10.1002/(SICI)1096-9896(200003)190:4<495::AID-PATH535>3.0.CO;2-T; PMID: 10700001
- Sassa Y, Yamasaki T, Horiuchi M, Inoshima Y, Ishiguro N. The effects of lysosomal and proteasomal inhibitors on abnormal forms of prion protein degradation in murine macrophages. Microbiol Immunol 2010; 54:763 - 8; http://dx.doi.org/10.1111/j.1348-0421.2010.00272.x; PMID: 21223366
- Takakura I, Miyazawa K, Kanaya T, Itani W, Watanabe K, Ohwada S, et al. Orally administered prion protein is incorporated by m cells and spreads into lymphoid tissues with macrophages in prion protein knockout mice. Am J Pathol 2011; 179:1301 - 9; http://dx.doi.org/10.1016/j.ajpath.2011.05.058; PMID: 21763679
- Swartzendruber DC, Congdon CC. ELECTRON MICROSCOPE OBSERVATIONS ON TINGIBLE BODY MACROPHAGES IN MOUSE SPLEEN. J Cell Biol 1963; 19:641 - 6; http://dx.doi.org/10.1083/jcb.19.3.641; PMID: 14086143
- Jeffrey M, Martin S, Thomson JR, Dingwall WS, Begara-McGorum I, González L. Onset and distribution of tissue prp accumulation in scrapie-affected suffolk sheep as demonstrated by sequential necropsies and tonsillar biopsies. J Comp Pathol 2001; 125:48 - 57; http://dx.doi.org/10.1053/jcpa.2001.0476; PMID: 11437516
- Jeffrey M, McGovern G, Martin S, Goodsir CM, Brown KL. Cellular and sub-cellular localisation of PrP in the lymphoreticular system of mice and sheep. Arch virol Supp 2000; 16:23-8.
- Ryder SJ, Dexter GE, Heasman L, Warner R, Moore SJ. Accumulation and dissemination of prion protein in experimental sheep scrapie in the natural host. BMC Vet Res 2009; 5:9; http://dx.doi.org/10.1186/1746-6148-5-9; PMID: 19243608
- Hilton DA, Ghani AC, Conyers L, Edwards P, McCardle L, Ritchie D, et al. Prevalence of lymphoreticular prion protein accumulation in UK tissue samples. J Pathol 2004; 203:733 - 9; http://dx.doi.org/10.1002/path.1580; PMID: 15221931
- Rezaie P, Lantos PL. Microglia and the pathogenesis of spongiform encephalopathies. Brain Res Brain Res Rev 2001; 35:55 - 72; http://dx.doi.org/10.1016/S0165-0173(01)00042-X; PMID: 11245886
- Perry VH, Cunningham C, Boche D. Atypical inflammation in the central nervous system in prion disease. Curr Opin Neurol 2002; 15:349 - 54; http://dx.doi.org/10.1097/00019052-200206000-00020; PMID: 12045736
- Rezaie P, Al-Sarraj S. Vacuolar degeneration affecting brain macrophages/microglia in variant CJD: a report on two cases. Acta Neuropathol 2007; 114:651 - 8; http://dx.doi.org/10.1007/s00401-007-0294-6; PMID: 17943296
- Kovács GG, Preusser M, Strohschneider M, Budka H. Subcellular localization of disease-associated prion protein in the human brain. Am J Pathol 2005; 166:287 - 94; http://dx.doi.org/10.1016/S0002-9440(10)62252-3; PMID: 15632020
- Ciesielski-Treska J, Grant NJ, Ulrich G, Corrotte M, Bailly Y, Haeberle A-M, et al. Fibrillar prion peptide (106-126) and scrapie prion protein hamper phagocytosis in microglia. Glia 2004; 46:101 - 15; http://dx.doi.org/10.1002/glia.10363; PMID: 15042579
- Hughes MM, Field RH, Perry VH, Murray CL, Cunningham C. Microglia in the degenerating brain are capable of phagocytosis of beads and of apoptotic cells, but do not efficiently remove PrPSc, even upon LPS stimulation. Glia 2010; 58:2017 - 30; http://dx.doi.org/10.1002/glia.21070; PMID: 20878768
- Boche D, Cunningham C, Docagne F, Scott H, Perry VH. TGFbeta1 regulates the inflammatory response during chronic neurodegeneration. Neurobiol Dis 2006; 22:638 - 50; http://dx.doi.org/10.1016/j.nbd.2006.01.004; PMID: 16510291
- Perry VH, Cunningham C, Holmes C. Systemic infections and inflammation affect chronic neurodegeneration. Nat Rev Immunol 2007; 7:161 - 7; http://dx.doi.org/10.1038/nri2015; PMID: 17220915
- Bulloch K, Miller MM, Gal-Toth J, Milner TA, Gottfried-Blackmore A, Waters EM, et al. CD11c/EYFP transgene illuminates a discrete network of dendritic cells within the embryonic, neonatal, adult, and injured mouse brain. J Comp Neurol 2008; 508:687 - 710; http://dx.doi.org/10.1002/cne.21668; PMID: 18386786
- Phan TG, Green JA, Gray EE, Xu Y, Cyster JG. Immune complex relay by subcapsular sinus macrophages and noncognate B cells drives antibody affinity maturation. Nat Immunol 2009; 10:786 - 93; http://dx.doi.org/10.1038/ni.1745; PMID: 19503106
- Phan TG, Grigorova I, Okada T, Cyster JG. Subcapsular encounter and complement-dependent transport of immune complexes by lymph node B cells. Nat Immunol 2007; 8:992 - 1000; http://dx.doi.org/10.1038/ni1494; PMID: 17660822
- Carrasco YR, Batista FD. B cells acquire particulate antigen in a macrophage-rich area at the boundary between the follicle and the subcapsular sinus of the lymph node. Immunity 2007; 27:160 - 71; http://dx.doi.org/10.1016/j.immuni.2007.06.007; PMID: 17658276
- Junt T, Moseman EA, Iannacone M, Massberg S, Lang PA, Boes M, et al. Subcapsular sinus macrophages in lymph nodes clear lymph-borne viruses and present them to antiviral B cells. Nature 2007; 450:110 - 4; http://dx.doi.org/10.1038/nature06287; PMID: 17934446
- Roozendaal R, Mempel TR, Pitcher LA, Gonzalez SF, Verschoor A, Mebius RE, et al. Conduits mediate transport of low-molecular-weight antigen to lymph node follicles. Immunity 2009; 30:264 - 76; http://dx.doi.org/10.1016/j.immuni.2008.12.014; PMID: 19185517
- Jeffrey M, González L, Espenes A, Press CM, Martin S, Chaplin M, et al. Transportation of prion protein across the intestinal mucosa of scrapie-susceptible and scrapie-resistant sheep. J Pathol 2006; 209:4 - 14; http://dx.doi.org/10.1002/path.1962; PMID: 16575799
- Ligios C, Cancedda MG, Carta A, Santucciu C, Maestrale C, Demontis F, et al. Sheep with scrapie and mastitis transmit infectious prions through the milk. J Virol 2011; 85:1136 - 9; http://dx.doi.org/10.1128/JVI.02022-10; PMID: 21084475
- Farquhar CF, Somerville RA, Ritchie LA. Post-mortem immunodiagnosis of scrapie and bovine spongiform encephalopathy. J Virol Methods 1989; 24:215 - 21; http://dx.doi.org/10.1016/0166-0934(89)90023-2; PMID: 2569471