Abstract
Prion protein (PrP) can be considered a pivotal molecule because it interacts with several partners to perform a diverse range of critical biological functions that might differ in embryonic and adult cells. In recent years, there have been major advances in elucidating the putative role of PrP in the basic biology of stem cells in many different systems. Here, we review the evidence indicating that PrP is a key molecule involved in driving different aspects of the potency of embryonic and tissue-specific stem cells in self-perpetuation and differentiation in many cell types. It has been shown that PrP is involved in stem cell self-renewal, controlling pluripotency gene expression, proliferation, and neural and cardiomyocyte differentiation. PrP also has essential roles in distinct processes that regulate tissue-specific stem cell biology in nervous and hematopoietic systems and during muscle regeneration. Results from our own investigations have shown that PrP is able to modulate self-renewal and proliferation in neural stem cells, processes that are enhanced by PrP interactions with stress inducible protein 1 (STI1). Thus, the available data reveal the influence of PrP in acting upon the maintenance of pluripotent status or the differentiation of stem cells from the early embryogenesis through adulthood.
Prion Protein in Embryonic Development
Although the prion protein (PrP) has been clearly associated with the pathogenesis of the prion diseases, a large number of reports have provided evidence that PrP is a pivotal molecule with fundamental roles in various physiological and developmental processes.Citation1-Citation4
PrP is expressed in a broad range of vertebrate tissues, but is expressed most abundantly in the central and peripheral nervous systems from early stages of development into adulthood.Citation1 During embryogenesis, murine PrP is first highly expressed between embryonic days (E) 7.5 and 8.5, predominately in post-mitotic neural cells that have undergone neuronal differentiation.Citation5 Expression subsequently expands to non-neuronal tissues at E13.5.Citation6 Interestingly, PrP expression in the human forebrain starts at the 11th week and continues to the end of gestation, and occurs predominantly in the axonal tract, suggesting a specific role for this molecule in axonal growth during development.Citation7
Since PrP is highly expressed in different tissues and is conserved among mammals, the identification of the normal function of PrP by studies in PrP-deficient mice strains was highly anticipated.Citation8,Citation9 However, the first studies in PrP-null mice did not reveal an obvious phenotype, implying either that PrP is not essential for normal development or that the function of PrP is compensated by other molecules.Citation8,Citation9 For instance, the potential role of PrP in supporting axonal growth can be compensated by upregulation of integrins in PrP-knockout mice.Citation10 However, subsequent studies have revealed that PrP-deficient mice, after appropriate challenge, present subtle phenotypes, such as alterations in neurotransmission and synaptic plasticity,Citation11-Citation13 hippocampal spatial memoryCitation14 and aversive hippocampal memory in aged animals,Citation15 circadian rhythms,Citation16,Citation17 and immune responses,Citation18,Citation19 as well as higher sensitivity to various stress conditions, which causes increased neuronal death.
Remarkably, diverse phenotypic abnormalities were observed when PrP mutants with specific deletions in the Prnp gene were re-introduced in PrP-deficient mice. Expression of PrP mutants (PrPΔ32–121 or PrPΔ32–134) leads to severe ataxia and apoptosis in the cerebellum.Citation20 Transgenic mice expressing truncated PrPΔ94–134 show extensive central and peripheral myelin degeneration and early ataxia.Citation21 Interestingly, the most significant phenotype was observed in animals expressing PrPΔ105–125. These animals develop an extremely severe illness, with cerebellar atrophy, decreased body size and weight, immobility, and death within one month.Citation22 It is important to note that the degenerative phenotype observed in those mice expressing the PrP truncated forms can be rescued by introducing the wild-type Prnp gene.Citation20-Citation22 Further studies determined that these deleted regions represent functional PrP domains necessary for interactions with several partners known to be associated with trophic functions.Citation23
The first dramatic phenotypes resulting from PrP loss-of-function were observed in zebrafish, in which the development of early and late structures is severely affected.Citation24 Notably, early knockdown of PrP in fish embryos is lethal, characterized by the loss of embryonic cell adhesion and arrested gastrulation, and the phenotype can be partially rescued by expression of mouse PrP.Citation24 Analyses of embryonic cells revealed that PrP is required for the proper membrane localization of E-cadherin adhesion complexes, indicating that PrP could have relevant and conserved roles in cell-cell communication mediated by cell adhesion.Citation24 In fact, the significant differences in PrP-knockdown phenotypes observed in zebrafish and PrP-null mice may be due to the activation of gene compensatory mechanisms in the embryonic stem (ES) cells selected to derive the mouse knockouts strains.Citation11 Hence, an examination of the immediate changes in gene expression profile upon PrP-knockout in mammalian ES cells and embryos might be helpful to clarify this issue.
To assess the role of PrP in the early stages of mammalian development, a critical and largely inaccessible period, some research groups have used pluripotent ES cells as an unsurpassed in vitro model. ES cells are typically derived from the inner cell mass of the preimplantation blastocyst and can be maintained indefinitely in a self-renewing state.Citation25 Previous studies revealed that the pluripotency state of ES cells is maintained by multiple soluble factors such as leukemia inhibitory factor (LIF),Citation26,Citation27 bone morphogenetic protein (BMP),Citation28 and Wnt,Citation29 as well as nuclear transcription factors such as STAT3,Citation30,Citation31 Oct3/4,Citation32,Citation33 Nanog,Citation34,Citation35 Sox236, c-mycCitation37 and Klf4.Citation38 However, the precise molecular mechanisms dictating whether the cells continue to undergo the process of self-renewal or depart from the self-renewing state remain unclear.
Recently developed methods now allow pluripotent stem cell lines with properties similar to mouse ES cells to be derived from several mammalian species in a variety of developmental stages, and from adult cells reprogrammed by ectopic transcription factors.Citation39 The first evidence showing the involvement of PrP in ES cell biology was reported by Lee and Baskakov using pluripotent human ES cells (hESCs).Citation40 These authors showed that treatment with recombinant PrP delays the spontaneous differentiation of hESCs and helps to maintain their high proliferation activity.Citation40
Additionally, Peralta and coworkers employed mouse ES cells to investigate whether PrP can modulate the neural commitment during early embryogenesis.Citation41 Their findings showed that PrP expression increased gradually as ES cells differentiated. Remarkably, the onset of expression of a neuroepithelium marker, nestin, was delayed in PrP-knockdown cultures, suggesting that PrP influences the differentiation of nestin-expressing cell lineages, including neural lineages.Citation41
Recently, Miranda and colleagues reported that during early ES cell differentiation, PrP is able to regulate mRNA expression levels of Nanog, a gene involved in self-renewal.Citation42 In addition, the authors reported that the regulation of Nanog transcription by PrP involves integrin (αvβ5), a heterodimeric transmembrane protein family involved in cell adhesion, signaling, migration, differentiation, and proliferation.Citation43 In line with these results, another report demonstrated that, during certain processes in embryogenesis, there is some redundancy in PrP interactions and the integrin signaling pathway,Citation10 which is crucial for mouse ES cells self-renewal regulation.Citation44 These results are supported further by previous studies, which revealed that integrin stimulation is related to Nanog expression,Citation45 and that PrP could be involved in integrin activity via E-cadherin, regulating cell adhesion.Citation24
Analysis of gene expression in ES cells revealed that PrP levels are elevated in ES cells during spontaneousCitation46 and cardiogenicCitation47 differentiation, and during directed reprogramming of somatic cells to a pluripotent state (induced-pluripotent stem cells, iPS).Citation48 Interestingly, it has been reported that PrP can be used to separate the cardiomyogenic cellular fraction derived from differentiating ES cells, indicating that PrP is an effective surface marker for identifying cardiomyogenic progenitors, which are able to differentiate into either cardiac or smooth muscle cells.Citation49
Collectively, these data indicate that PrP regulates key functions in modulating the self-renewal/differentiation status of stem cells in early embryogenesis, and provide essential clues about when potential compensatory phenomena could be established.
PrP Guides the Biology of Tissue-Specific Stem Cells
Neural stem cells
A better understanding of the trophic properties of PrP in the basic biology of tissue-specific stem cells in many different systems has emerged in the last few years, indicating that this protein performs pivotal functions not only during embryogenesis, but throughout the lifespan, as well.Citation50 In fact, published data support a role for PrP in the biology of multipotent stem cells, which are found in a variety of organs and have a pronounced capacity for self-renewal and restricted ability to differentiate into tissue-specific stem cell types.
Given the presence of PrP in both developing and adult nervous tissue, it is likely that PrP is involved in neural stem cell (NSC) behavior. NSCs are the primary progenitors that give rise to neurons and glia in the embryonic, neonatal and adult brain.Citation51,Citation52 Specific neural markers can be used to identify the different cell lineages in the brain, including glial fibrillary acidic protein (GFAP), which identifies glia, and βIII-tubulin, which identifies neurons.Citation52
In 2006, Steele and coworkers, found a correlation between the levels of PrP and the differentiation rate for neuronal phenotype acquisition by comparing NSC derived from embryonic brains of PrP-null, PrP-overexpressing, and PrP wild-type mice.Citation50 Additional experiments in this study showed that increasing PrP expression is associated positively with NSC proliferation in neurogenic regions in the adult brain including the subventricular zone and hippocampus.Citation50 These results have major implications for understanding the function of PrP in adult neurogenesis and neural plasticity, since PrP could be one of the molecules involved in maintenance of the adult NSC niche.
Consistent with previous observations revealing that PrP levels are related to neuronal phenotype in murine embryonic brain, the Witusik and colleagues have shown that PrP expression in NSC derived from human fetuses varies with the progression of neuronal differentiation, supporting the idea that PrP is related to neuronal phenotype.Citation53 However, PrP expression is maintained at consistent levels during gliogenesis.Citation53 Interestingly, our group has shown that fetal brains from PrP-null mice present reduced levels of GFAP and increased levels of nestin and vimentin (immature cell markers) when compared with wild-type counterparts, indicating a delay of astrocyte development.Citation54 These results corroborate with previous findings that showed a slower maturation rate in the PrP-null developing brain.Citation50
Notably, a recent study from our group highlighted the function of PrP in the biology of NSC.Citation55 We showed that NSC derived from PrP-knockout mice and cultured as neurospheres present expression profiles of neuronal or glial markers that are similar to the expression profiles of NSC from wild-type mice. The expression pattern of nestin at the neurosphere periphery is coincident with the proliferative cell zone, suggesting that the sphere margins represent a true niche for uncommitted cells as a result of the continuous exposure to mitogenic factors in the medium. In addition, the self-renewing capacity of NSC can be modulated by PrP expression and activity. Through clonal assays, it was shown that PrP null-derived neurospheres have a lower capacity for self-renewal. This effect was mimicked in wild-type neurospheres using antibodies to impair PrP activity, showing that PrP is important for NSC maintenance.Citation55 As observed in other studies, PrP activity can be modulated by a secretable form of a co-chaperone protein, stress inducible protein 1 (STI1). STI1 specifically binds to PrP and leads to a significant number of biological effects such as neuritogenesis and neuroprotectionCitation56,Citation57 through α7 nicotinic acetylcholine receptor activity,Citation58 and astrocyte development,Citation54 and also alters in vivo processes such as short-term memory formation and long-term memory consolidation.Citation59 We found that STI1 positively modulates the self-renewal of NSC in a PrP-dependent manner and this effect can be disrupted with STI1 antibodies. The mechanism by which STI1 enhances self-renewal requires the induction of cell proliferation, without apparent involvement of cell protectionCitation56 (). Thus, these findings strengthen the concept that the ability of PrP to support self-renewal and proliferation makes it essential for continued maintenance of NSC. However, whether the PrP-STI1 complex also operates in vivo during normal development and/or adult brain function remains to be determined. All these findings combined suggest that PrP is able to orchestrate distinct processes related to NSC fate and maintenance through of the modulation of self-renewal, differentiation and proliferation.
Figure 1. PrP is expressed in neural stem cells and modulates self-renewal and proliferation. (A) Representative images depicting immunofluorescent staining in neurospheres derived from PrP-expressing mice and cultured over laminin for 24h. Staining with specific antibodies was performed to detect GFAP (astrocytes, green) and PrP (red), and the nuclei were stained with DAPI (blue). (B) Scheme showing that neurosphere formation is increased in wild-type (Prnp+/+) cells in the presence of STI1, but is impaired in PrP-null (Prnp−/−) cells. Note that the signaling pathways triggered by PrP-STI1 engagement during proliferation and self-renewal processes (detailed in the inset), as well as the role of the PrP-STI1 complex in the classical differentiation of NSC, require further investigation.
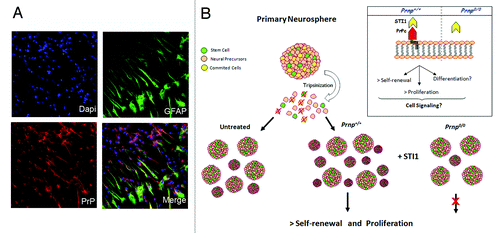
Non-neural stem cells
Since PrP is expressed in a variety of non-neural cells during the lifespan of many species, its role in other tissue-specific stem cells types has been investigated, too. PrP is also readily detected in the hematopoietic cell system, a highly dynamic tissue characterized by continual removal and renewal of all cell types.Citation60 The involvement of PrP in blood cells has been investigated since the early 1990s,Citation61 and one of the first reports that suggested a role for PrP in hematopoietic precursors was published in 1998 by Neil Cashman’s group.Citation62 Using human peripheral blood leukocytes and bone marrow precursor cells, this group demonstrated that PrP expression is maintained throughout lymphocyte and monocyte differentiation, while granulocyte maturation is characterized by PrP downregulation.Citation62 This phenomenon could be mimicked in vitro using a human premyeloid cell line (HL-60), which can be induced to differentiate along either the granulocyte or monocyte lineage using pharmacological inductors.Citation63 In HL-60 cells, PrP is expressed during the undifferentiated state and persists when the cells are induced to differentiate along the monocyte lineage. Conversely, the induction of differentiation along the granulocyte lineage represses PrP expression.Citation62
Distinct conclusions were reached by Liu and coworkers, who investigated PrP expression in mouse hematopoietic precursors.Citation64 The authors showed that mature lymphoid cells in the spleen do not express detectable amounts of PrP. However, PrP expression could be detected in immature thymic T cells, conceivably suggesting that PrP is repressed during T cell maturation.Citation64 Furthermore, this group found that the expression of PrP in bone marrow occurs preferentially in the precursor cell population, including hematopoietic stem cells (HSC) and lymphoid and myeloid progenitors, and is repressed in mature lymphocytes. These data are further supported by finding that in vitro-induced differentiation leads to a decrease in the number of PrP+ precursors cells, indicating that this population contains cells able to give rise to other blood cell types.Citation64
Another important contribution was provided in a study by Zhang and colleagues that demonstrated that PrP also supports self-renewal in HSC.Citation65 They showed that irradiation is lethal for PrP-null mice because their HSC are unable to reconstitute the bone marrow. Furthermore, ectopic PrP expression in PrP-null cells can rescue the malfunction in cell reconstitution assays. These findings provide clear evidence that PrP is important for supporting blood cell repopulating activity.Citation65 Taken together, these data refine the understanding of PrP function in HSC and more restricted progenitors to include the indispensable role of PrP in long-term HSC self-renewal and its potential as a reliable cell marker to identify specific blood cell lineages.
Finally, PrP function has also been investigated in adult myogenic precursors in an animal model of muscle regeneration.Citation66 One of the features of adult stem cells is the ability to regenerate specific tissues, recapitulating mechanisms observed during morphogenesis.Citation67 The authors compared the regeneration of acutely damaged hind-limbs of PrP-expressing and non-expressing mice and observed that the absence of PrP is associated with slower muscle recovery. These observations suggest that PrP could have an important role in the regeneration process, specifically in the proliferation and differentiation of myogenic precursor cells.Citation66
Concluding Remarks
The accumulating evidence associating PrP with a variety of physiological functions during development and adulthood confirms that PrP is not only responsible for mediating neurodegenerative processes. As described in this review, a putative role for PrP as a potent molecule able to regulate many stages in the cell cycle, from the pluripotent state of stem cells to the homeostatic state of adult-tissues, is emerging. The most consistent data regarding the function of PrP in the basic biology of stem cells has been described in neural tissue, perhaps because of the prominent expression and activity of PrP in this tissue. Many of the trophic properties attributed to PrP could be functionally comparable to key classical pathways indispensable for maintenance of stem cell status. However, a number of questions remain to be answered in order to fully explain the contribution of PrP in the control of distinct features inherent to stem cells.
Abbreviations: | ||
PrP | = | prion protein |
ES | = | embryonic stem |
NSC | = | neural stem cells |
HSC | = | hematopoietic stem cells |
Acknowledgments
We dedicate this work to the memory of Professor Ricardo Renzo Brentani, a genuine and tenacious revolutionary scientist, for his extraordinary and genial contribution for Brazilian science. The authors gratefully acknowledge Dr. Flavio Beraldo for helpful contribution on manuscript revision.
References
- Linden R, Martins VR, Prado MA, Cammarota M, Izquierdo I, Brentani RR. Physiology of the prion protein. Physiol Rev 2008; 88:673 - 728; http://dx.doi.org/10.1152/physrev.00007.2007; PMID: 18391177
- Caughey B, Baron GS. Prions and their partners in crime. Nature 2006; 443:803 - 10; http://dx.doi.org/10.1038/nature05294; PMID: 17051207
- Westaway D, Prusiner SB. Conservation of the cellular gene encoding the scrapie prion protein. Nucleic Acids Res 1986; 14:2035 - 44; http://dx.doi.org/10.1093/nar/14.5.2035; PMID: 2870469
- Manson J, West JD, Thomson V, McBride P, Kaufman MH, Hope J. The prion protein gene: a role in mouse embryogenesis?. Development 1992; 115:117 - 22; PMID: 1353438
- Tremblay P, Bouzamondo-Bernstein E, Heinrich C, Prusiner SB, DeArmond SJ. Developmental expression of PrP in the post-implantation embryo. Brain Res 2007; 1139:60 - 7; http://dx.doi.org/10.1016/j.brainres.2006.12.055; PMID: 17292334
- Hajj GN, Santos TG, Cook ZS, Martins VR. Developmental expression of prion protein and its ligands stress-inducible protein 1 and vitronectin. J Comp Neurol 2009; 517:371 - 84; http://dx.doi.org/10.1002/cne.22157; PMID: 19760599
- Adle-Biassette H, Verney C, Peoc’h K, Dauge MC, Razavi F, Choudat L, et al. Immunohistochemical expression of prion protein (PrPC) in the human forebrain during development. J Neuropathol Exp Neurol 2006; 65:698 - 706; http://dx.doi.org/10.1097/01.jnen.0000228137.10531.72; PMID: 16825956
- Büeler H, Fischer M, Lang Y, Bluethmann H, Lipp HP, DeArmond SJ, et al. Normal development and behaviour of mice lacking the neuronal cell-surface PrP protein. Nature 1992; 356:577 - 82; http://dx.doi.org/10.1038/356577a0; PMID: 1373228
- Manson JC, Clarke AR, Hooper ML, Aitchison L, McConnell I, Hope J. 129/Ola mice carrying a null mutation in PrP that abolishes mRNA production are developmentally normal. Mol Neurobiol 1994; 8:121 - 7; http://dx.doi.org/10.1007/BF02780662; PMID: 7999308
- Hajj GN, Lopes MH, Mercadante AF, Veiga SS, da Silveira RB, Santos TG, et al. Cellular prion protein interaction with vitronectin supports axonal growth and is compensated by integrins. J Cell Sci 2007; 120:1915 - 26; http://dx.doi.org/10.1242/jcs.03459; PMID: 17504807
- Collinge J, Whittington MA, Sidle KC, Smith CJ, Palmer MS, Clarke AR, et al. Prion protein is necessary for normal synaptic function. Nature 1994; 370:295 - 7; http://dx.doi.org/10.1038/370295a0; PMID: 8035877
- Maglio LE, Perez MF, Martins VR, Brentani RR, Ramirez OA. Hippocampal synaptic plasticity in mice devoid of cellular prion protein. Brain Res Mol Brain Res 2004; 131:58 - 64; http://dx.doi.org/10.1016/j.molbrainres.2004.08.004; PMID: 15530652
- Prestori F, Rossi P, Bearzatto B, Lainé J, Necchi D, Diwakar S, et al. Altered neuron excitability and synaptic plasticity in the cerebellar granular layer of juvenile prion protein knock-out mice with impaired motor control. J Neurosci 2008; 28:7091 - 103; http://dx.doi.org/10.1523/JNEUROSCI.0409-08.2008; PMID: 18614678
- Criado JR, Sánchez-Alavez M, Conti B, Giacchino JL, Wills DN, Henriksen SJ, et al. Mice devoid of prion protein have cognitive deficits that are rescued by reconstitution of PrP in neurons. Neurobiol Dis 2005; 19:255 - 65; http://dx.doi.org/10.1016/j.nbd.2005.01.001; PMID: 15837581
- Coitinho AS, Roesler R, Martins VR, Brentani RR, Izquierdo I. Cellular prion protein ablation impairs behavior as a function of age. Neuroreport 2003; 14:1375 - 9; PMID: 12876477
- Tobler I, Deboer T, Fischer M. Sleep and sleep regulation in normal and prion protein-deficient mice. J Neurosci 1997; 17:1869 - 79; PMID: 9030645
- Tobler I, Gaus SE, Deboer T, Achermann P, Fischer M, Rülicke T, et al. Altered circadian activity rhythms and sleep in mice devoid of prion protein. Nature 1996; 380:639 - 42; http://dx.doi.org/10.1038/380639a0; PMID: 8602267
- de Almeida CJ, Chiarini LB, da Silva JP, E Silva PM, Martins MA, Linden R. The cellular prion protein modulates phagocytosis and inflammatory response. J Leukoc Biol 2005; 77:238 - 46; http://dx.doi.org/10.1189/jlb.1103531; PMID: 15539455
- Bainbridge J, Walker KB. The normal cellular form of prion protein modulates T cell responses. Immunol Lett 2005; 96:147 - 50; http://dx.doi.org/10.1016/j.imlet.2004.08.006; PMID: 15585317
- Shmerling D, Hegyi I, Fischer M, Blättler T, Brandner S, Götz J, et al. Expression of amino-terminally truncated PrP in the mouse leading to ataxia and specific cerebellar lesions. Cell 1998; 93:203 - 14; http://dx.doi.org/10.1016/S0092-8674(00)81572-X; PMID: 9568713
- Baumann F, Tolnay M, Brabeck C, Pahnke J, Kloz U, Niemann HH, et al. Lethal recessive myelin toxicity of prion protein lacking its central domain. EMBO J 2007; 26:538 - 47; http://dx.doi.org/10.1038/sj.emboj.7601510; PMID: 17245436
- Li A, Christensen HM, Stewart LR, Roth KA, Chiesa R, Harris DA. Neonatal lethality in transgenic mice expressing prion protein with a deletion of residues 105-125. EMBO J 2007; 26:548 - 58; http://dx.doi.org/10.1038/sj.emboj.7601507; PMID: 17245437
- Martins VR, Beraldo FH, Hajj GN, Lopes MH, Lee KS, Prado MM, et al. Prion protein: orchestrating neurotrophic activities. Curr Issues Mol Biol 2010; 12:63 - 86; PMID: 19767651
- Málaga-Trillo E, Solis GP, Schrock Y, Geiss C, Luncz L, Thomanetz V, et al. Regulation of embryonic cell adhesion by the prion protein. PLoS Biol 2009; 7:e55; http://dx.doi.org/10.1371/journal.pbio.1000055; PMID: 19278297
- Evans MJ, Kaufman MH. Establishment in culture of pluripotential cells from mouse embryos. Nature 1981; 292:154 - 6; http://dx.doi.org/10.1038/292154a0; PMID: 7242681
- Smith SK, Charnock-Jones DS, Sharkey AM. The role of leukemia inhibitory factor and interleukin-6 in human reproduction. Hum Reprod 1998; 13:Suppl 3 237 - 43, discussion 244-6; PMID: 9755426
- Williams RL, Hilton DJ, Pease S, Willson TA, Stewart CL, Gearing DP, et al. Myeloid leukaemia inhibitory factor maintains the developmental potential of embryonic stem cells. Nature 1988; 336:684 - 7; http://dx.doi.org/10.1038/336684a0; PMID: 3143916
- Ying QL, Nichols J, Chambers I, Smith A. BMP induction of Id proteins suppresses differentiation and sustains embryonic stem cell self-renewal in collaboration with STAT3. Cell 2003; 115:281 - 92; http://dx.doi.org/10.1016/S0092-8674(03)00847-X; PMID: 14636556
- Sato N, Meijer L, Skaltsounis L, Greengard P, Brivanlou AH. Maintenance of pluripotency in human and mouse embryonic stem cells through activation of Wnt signaling by a pharmacological GSK-3-specific inhibitor. Nat Med 2004; 10:55 - 63; http://dx.doi.org/10.1038/nm979; PMID: 14702635
- Niwa H, Burdon T, Chambers I, Smith A. Self-renewal of pluripotent embryonic stem cells is mediated via activation of STAT3. Genes Dev 1998; 12:2048 - 60; http://dx.doi.org/10.1101/gad.12.13.2048; PMID: 9649508
- Matsuda T, Nakamura T, Nakao K, Arai T, Katsuki M, Heike T, et al. STAT3 activation is sufficient to maintain an undifferentiated state of mouse embryonic stem cells. EMBO J 1999; 18:4261 - 9; http://dx.doi.org/10.1093/emboj/18.15.4261; PMID: 10428964
- Nichols J, Zevnik B, Anastassiadis K, Niwa H, Klewe-Nebenius D, Chambers I, et al. Formation of pluripotent stem cells in the mammalian embryo depends on the POU transcription factor Oct4. Cell 1998; 95:379 - 91; http://dx.doi.org/10.1016/S0092-8674(00)81769-9; PMID: 9814708
- Niwa H, Miyazaki J, Smith AG. Quantitative expression of Oct-3/4 defines differentiation, dedifferentiation or self-renewal of ES cells. Nat Genet 2000; 24:372 - 6; http://dx.doi.org/10.1038/74199; PMID: 10742100
- Mitsui K, Tokuzawa Y, Itoh H, Segawa K, Murakami M, Takahashi K, et al. The homeoprotein Nanog is required for maintenance of pluripotency in mouse epiblast and ES cells. Cell 2003; 113:631 - 42; http://dx.doi.org/10.1016/S0092-8674(03)00393-3; PMID: 12787504
- Chambers I, Colby D, Robertson M, Nichols J, Lee S, Tweedie S, et al. Functional expression cloning of Nanog, a pluripotency sustaining factor in embryonic stem cells. Cell 2003; 113:643 - 55; http://dx.doi.org/10.1016/S0092-8674(03)00392-1; PMID: 12787505
- Avilion AA, Nicolis SK, Pevny LH, Perez L, Vivian N, Lovell-Badge R. Multipotent cell lineages in early mouse development depend on SOX2 function. Genes Dev 2003; 17:126 - 40; http://dx.doi.org/10.1101/gad.224503; PMID: 12514105
- Cartwright P, McLean C, Sheppard A, Rivett D, Jones K, Dalton S. LIF/STAT3 controls ES cell self-renewal and pluripotency by a Myc-dependent mechanism. Development 2005; 132:885 - 96; http://dx.doi.org/10.1242/dev.01670; PMID: 15673569
- Li Y, McClintick J, Zhong L, Edenberg HJ, Yoder MC, Chan RJ. Murine embryonic stem cell differentiation is promoted by SOCS-3 and inhibited by the zinc finger transcription factor Klf4. Blood 2005; 105:635 - 7; http://dx.doi.org/10.1182/blood-2004-07-2681; PMID: 15358627
- Ralston A, Rossant J. The genetics of induced pluripotency. Reproduction 2010; 139:35 - 44; http://dx.doi.org/10.1530/REP-09-0024; PMID: 19605512
- Lee YJ, Baskakov IV. Treatment with normal prion protein delays differentiation and helps to maintain high proliferation activity in human embryonic stem cells. J Neurochem 2010; 114:362 - 73; http://dx.doi.org/10.1111/j.1471-4159.2010.06601.x; PMID: 20089130
- Peralta OA, Huckle WR, Eyestone WH. Expression and knockdown of cellular prion protein (PrPC) in differentiating mouse embryonic stem cells. Differentiation 2011; 81:68 - 77; http://dx.doi.org/10.1016/j.diff.2010.09.181; PMID: 20926176
- Miranda A, Pericuesta E, Ramírez MA, Gutierrez-Adan A. Prion protein expression regulates embryonic stem cell pluripotency and differentiation. PLoS One 2011; 6:e18422; http://dx.doi.org/10.1371/journal.pone.0018422; PMID: 21483752
- Huveneers S, Truong H, Danen HJ. Integrins: signaling, disease, and therapy. Int J Radiat Biol 2007; 83:743 - 51; http://dx.doi.org/10.1080/09553000701481808; PMID: 17852562
- Hayashi Y, Furue MK, Okamoto T, Ohnuma K, Myoishi Y, Fukuhara Y, et al. Integrins regulate mouse embryonic stem cell self-renewal. Stem Cells 2007; 25:3005 - 15; http://dx.doi.org/10.1634/stemcells.2007-0103; PMID: 17717067
- Lee ST, Yun JI, Jo YS, Mochizuki M, van der Vlies AJ, Kontos S, et al. Engineering integrin signaling for promoting embryonic stem cell self-renewal in a precisely defined niche. Biomaterials 2010; 31:1219 - 26; http://dx.doi.org/10.1016/j.biomaterials.2009.10.054; PMID: 19926127
- Hailesellasse Sene K, Porter CJ, Palidwor G, Perez-Iratxeta C, Muro EM, Campbell PA, et al. Gene function in early mouse embryonic stem cell differentiation. BMC Genomics 2007; 8:85; http://dx.doi.org/10.1186/1471-2164-8-85; PMID: 17394647
- Faustino RS, Behfar A, Perez-Terzic C, Terzic A. Genomic chart guiding embryonic stem cell cardiopoiesis. Genome Biol 2008; 9:R6; http://dx.doi.org/10.1186/gb-2008-9-1-r6; PMID: 18184438
- Mikkelsen TS, Hanna J, Zhang X, Ku M, Wernig M, Schorderet P, et al. Dissecting direct reprogramming through integrative genomic analysis. Nature 2008; 454:49 - 55; http://dx.doi.org/10.1038/nature07056; PMID: 18509334
- Hidaka K, Shirai M, Lee JK, Wakayama T, Kodama I, Schneider MD, et al. The cellular prion protein identifies bipotential cardiomyogenic progenitors. Circ Res 2010; 106:111 - 9; http://dx.doi.org/10.1161/CIRCRESAHA.109.209478; PMID: 19910576
- Steele AD, Emsley JG, Ozdinler PH, Lindquist S, Macklis JD. Prion protein (PrPc) positively regulates neural precursor proliferation during developmental and adult mammalian neurogenesis. Proc Natl Acad Sci U S A 2006; 103:3416 - 21; http://dx.doi.org/10.1073/pnas.0511290103; PMID: 16492732
- Alvarez-Buylla A, Seri B, Doetsch F. Identification of neural stem cells in the adult vertebrate brain. Brain Res Bull 2002; 57:751 - 8; http://dx.doi.org/10.1016/S0361-9230(01)00770-5; PMID: 12031271
- Gage FH. Mammalian neural stem cells. Science 2000; 287:1433 - 8; http://dx.doi.org/10.1126/science.287.5457.1433; PMID: 10688783
- Witusik M, Gresner SM, Hulas-Bigoszewska K, Krynska B, Azizi SA, Liberski PP, et al. Neuronal and astrocytic cells, obtained after differentiation of human neural GFAP-positive progenitors, present heterogeneous expression of PrPc. Brain Res 2007; 1186:65 - 73; http://dx.doi.org/10.1016/j.brainres.2007.10.039; PMID: 17996224
- Arantes C, Nomizo R, Lopes MH, Hajj GN, Lima FR, Martins VR. Prion protein and its ligand stress inducible protein 1 regulate astrocyte development. Glia 2009; 57:1439 - 49; http://dx.doi.org/10.1002/glia.20861; PMID: 19243016
- Santos TG, Silva IR, Costa-Silva B, Lepique AP, Martins VR, Lopes MH. Enhanced neural progenitor/stem cells self-renewal via the interaction of stress-inducible protein 1 with the prion protein. Stem Cells 2011; 29:1126 - 36; http://dx.doi.org/10.1002/stem.664; PMID: 21608082
- Lopes MH, Hajj GN, Muras AG, Mancini GL, Castro RM, Ribeiro KC, et al. Interaction of cellular prion and stress-inducible protein 1 promotes neuritogenesis and neuroprotection by distinct signaling pathways. J Neurosci 2005; 25:11330 - 9; http://dx.doi.org/10.1523/JNEUROSCI.2313-05.2005; PMID: 16339028
- Chiarini LB, Freitas AR, Zanata SM, Brentani RR, Martins VR, Linden R. Cellular prion protein transduces neuroprotective signals. EMBO J 2002; 21:3317 - 26; http://dx.doi.org/10.1093/emboj/cdf324; PMID: 12093733
- Beraldo FH, Arantes CP, Santos TG, Queiroz NG, Young KF, Rylett RJ, et al. Role of alpha7 nicotinic acetylcholine receptor in calcium signaling induced by prion protein interaction with stress-inducible protein 1. J Biol Chem 2010; 285:36542 - 50; http://dx.doi.org/10.1074/jbc.M110.157263; PMID: 20837487
- Coitinho AS, Lopes MH, Hajj GN, Rossato JI, Freitas AR, Castro CC, et al. Short-term memory formation and long-term memory consolidation are enhanced by cellular prion association to stress-inducible protein 1. Neurobiol Dis 2007; 26:282 - 90; http://dx.doi.org/10.1016/j.nbd.2007.01.005; PMID: 17329112
- Domen J, Weissman IL. Self-renewal, differentiation or death: regulation and manipulation of hematopoietic stem cell fate. Mol Med Today 1999; 5:201 - 8; http://dx.doi.org/10.1016/S1357-4310(99)01464-1; PMID: 10322312
- Cashman NR, Loertscher R, Nalbantoglu J, Shaw I, Kascsak RJ, Bolton DC, et al. Cellular isoform of the scrapie agent protein participates in lymphocyte activation. Cell 1990; 61:185 - 92; http://dx.doi.org/10.1016/0092-8674(90)90225-4; PMID: 1969332
- Dodelet VC, Cashman NR. Prion protein expression in human leukocyte differentiation. Blood 1998; 91:1556 - 61; PMID: 9473220
- Rovera G, O’Brien TG, Diamond L. Induction of differentiation in human promyelocytic leukemia cells by tumor promoters. Science 1979; 204:868 - 70; http://dx.doi.org/10.1126/science.286421; PMID: 286421
- Liu T, Li R, Wong BS, Liu D, Pan T, Petersen RB, et al. Normal cellular prion protein is preferentially expressed on subpopulations of murine hemopoietic cells. J Immunol 2001; 166:3733 - 42; PMID: 11238614
- Zhang CC, Steele AD, Lindquist S, Lodish HF. Prion protein is expressed on long-term repopulating hematopoietic stem cells and is important for their self-renewal. Proc Natl Acad Sci U S A 2006; 103:2184 - 9; http://dx.doi.org/10.1073/pnas.0510577103; PMID: 16467153
- Stella R, Massimino ML, Sandri M, Sorgato MC, Bertoli A. Cellular prion protein promotes regeneration of adult muscle tissue. Mol Cell Biol 2010; 30:4864 - 76; http://dx.doi.org/10.1128/MCB.01040-09; PMID: 20679477
- Tanaka EM, Reddien PW. The cellular basis for animal regeneration. Dev Cell 2011; 21:172 - 85; http://dx.doi.org/10.1016/j.devcel.2011.06.016; PMID: 21763617