Abstract
The ability of abnormal TSE-associated forms of PrP to seed the formation of amyloid fibrils from recombinant PrPSen has served as the basis for several relatively rapid and highly sensitive tests for prion diseases. These tests include rPrP-PMCA (rPMCA), standard quaking-induced conversion (S-QuIC), amyloid seeding assay (ASA), real-time QuIC (RT-QuIC) and enhanced QuIC (eQuIC). Here, we summarize recent improvements in the RT-QuIC-based assays that enhance the practicality, sensitivity and quantitative attributes of assays QuIC and promote the detection of prion seeding activity in dilute, inhibitor-laden fluids such as blood plasma.
Introduction
The pathogen (prion) associated with the transmissible spongiform encephalopathies (TSEs) or prion diseases of mammals is largely a misfolded multimeric form of the host’s prion protein (PrP).Citation1 TSE-associated forms of PrP, e.g., PrPSc, PrPRes, or PrPTSE, can induce or seed the conversion of their normal counterpart, PrPC or PrPSen, to forms that, like PrPSc itself, are higher in β sheet content, polymeric, and more protease-resistant. A key problem in attempting to cope with TSE diseases in humans, livestock and wildlife is the lack of sufficiently practical and sensitive assays for routine detection of prions and early diagnosis of TSEs. The propagation of prions without classical immune responses or the production of pathogen-specific nucleic acids or polypeptides negates the use of many methods that are commonly used for detecting infections of conventional pathogens. However, the distinct seeding activity of PrPSc serves as the basis of recently developed ultrasensitive tests for prion infections such as protein misfolding cyclic amplification (PMCA),Citation2-Citation4 quaking-induced conversion (QuIC),Citation5-Citation8 the amyloid seeding assay (ASA),Citation9 the surround optical fiber immunoassay (SOPHIA),Citation10 and othersCitation11 (). In some cases,Citation2,Citation8 samples containing ≤ 1 ag (10−18 g) PrPRes can seed the formation of ng-µg amounts of protease-resistant PrP that are readily detectable by conventional methods, amounting to signal amplifications of greater than a billion-fold. In this article, we will emphasize recent improvements in assays of this sort that are bringing ultrasensitive prion detection into the realm of practical applicability in medicine, agriculture and wildlife biology.
Table 1. Comparison of selected highly sensitive prion/PrPSc assays
Recombinant PrP PMCA (rPrP-PMCA), standard QuIC (S-QuIC) and ASA
The rPrP-PMCA,Citation4 S-QuIC,Citation5,Citation8 and ASACitation9 assays use bacterially expressed recombinant PrP (rPrP) as a substrate for prion-seeded conversion to rPrP amyloid fibrils. In the former two assays, the rPrP amyloid is detected as specific proteinase K (PK)-resistant bands by immunoblotting, while in the ASA the amyloid is detected using the amyloid-sensitive dye thioflavin T (ThT). Although each of these highly sensitive assays provided more rapid and practical approaches to the detection of prion seeding activity, they had certain practical limitations that have been discussed previously.Citation6,Citation8,Citation12-Citation14 Notably, the rPrP-PMCA requires the sonication of reaction tubes, which can be difficult to control. In the S-QuIC and ASA assays, sonication is replaced with more easily controlled and replicable shaking of reaction vessels. The ability of the rPrP-PMCA and S-QuIC assays to handle large numbers of samples is limited by the need to perform reactions in individual microtubes and to detect reaction products by immunoblotting. The ASA employs a much higher-throughput multi-well plate reaction format with a simple fluorescence readout, but is complicated to some extent by the relatively rapid formation of ThT-positive reactions in negative control samples.Citation9 Consequently discrimination of prion-seeded vs. unseeded reactions in the ASA depends on careful comparisons of lag times.
Real-Time Quaking Induced Conversion (RT-QuIC)
To address these limitations, Atarashi and colleagues originated the RT-QuIC assay which blends the high-throughput multiwell plate format and ThT fluorescence readout of the ASA with conditions that, as in the S-QuIC, were selected to minimize the formation of spontaneous (prion-independent) ThT-positive amyloid fibrils.Citation6,Citation12 Applications of the RT-QuIC for the detection of various types of human CJD showed that it can detect up to 1010-fold dilutions of sporadic CJD (sCJD) brain homogenates containing ~1 fg of PrPRes. Moreover the assay is specific with respect to a variety of non-CJD brain tissue controls.Citation12,Citation15 Curiously, for reasons that remain unknown, the lag phases in RT-QuIC reactions seeded with variant CJD (vCJD) tend to be much longer than those for reactions seeded with comparable dilutions of sCJD brain homogenate.Citation15
sCJD diagnostics using RT-QuIC
A particularly significant application of the RT-QuIC is in the diagnosis of sCJD using cerebrospinal fluid (CSF) samples.Citation12 Antemortem CJD diagnoses can be difficult and relies on a battery of tests including clinical signs, EEG, MRI, and assays for CSF markers such as the 14–3-3 and tau proteins that are not specific for TSE. The RT-QuIC offers the opportunity to test for a TSE-specific marker, i.e., PrPCJD. Analyses of panels of CSF samples from sCJD and non-CJD patients from Japan and Australia showed that the RT-QuIC had > 80% sensitivity and 100% specificity in identifying CJD specimens. Moreover, all of the different sCJD subtypes were reactive in the RT-QuIC assay. Concurrent tests using the 14–3-3 assay revealed that the RT-QuIC was substantially more specific for CJD than the standard 14–3-3 assay. Blinded analyses of other large panels of CSF specimens from sCJD and non-CJD controls by another laboratory have obtained similar results (LI McGuire, AH Peden, CD Orrù, JM Wilham, NE Appleford, G Mallinson, M Andrews, MW Head, B Caughey, RG Will, RSG Knight, AJE Green, Annals of Neurology, in press), fueling efforts to further implement and evaluate RT-QuIC as a diagnostic test for sCJD in multiple centers around the world.
RT-QuIC detection of animal TSEs
RT-QuIC has also been applied to the detection of sheep scrapie, rodent-adapted scrapie and chronic wasting disease (CWD) of cervids.Citation6 With a hamster-adapted scrapie strain (263K), the RT-QuIC sensitivity for detection of brain homogenate dilutions was found to be comparable to bioassay in hamsters by intracerebral inoculation. Moreover, RT-QuIC readily detected prion seeding activity in cerebral spinal fluid and nasal lavages of prion-infected hamsters.
Quantitation of prion seeding activity by end-point dilution RT-QuIC
Analysis of serial dilutions of test samples by RT-QuIC provides a means of quantitating relative concentrations of prion seeding activity (). The approach is conceptually analogous to end-point dilution titrations in animal bioassays in which the sample dose causing disease in 50% of the inoculated animals (i.e., the 50% lethal dose or LD50) is estimated. With RT-QuIC the dose giving positive reactions in 50% of replicate reactions, i.e., the 50% seeding dose or SD50, is determined. Back calculations then give the concentration of SD50 per unit volume or weight of original samples. Such analyses have allowed the first in vitro measurements of seed concentrations in the CSF and nasal fluids of prion infected hamsters.Citation6 The detection of substantial levels of seeding activity and infectivity in nasal fluids is consistent with the detection of PrPRes in the olfactory epithelia of hamstersCitation6,Citation16 and humansCitation17 and supports the notion that the nasal mucosa may be a significant source of prion shedding from infected individuals. Furthermore a more recent study found that irritation of the nasal cavity can increase the release of prion seeding activity into nasal fluids by 100–1000-fold.Citation18
Figure 1. End-point dilution quantitation of prion seeding activity by RT-QuIC. Top panel: We show the average relative fluorescence from RT-QuIC reactions seeded by dilutions (8 replicate wells per dilution) of hamster 263K scrapie brain homogenates as a function of time. With more dilute seed samples, the lag phase prior to the rapid fibrilization phase increases and stepwises increases in the fluorescence can be seen as individual reactions become positive. Near the endpoint dilution (in this case the 10−9 dilution) only a subset of the replicate reactions became ThT positive. Bottom panel: Comparison of proportion of positive replicate RT-QuIC reactions (blue) and clinically affected hamsters (black) inoculated with the designated dilutions of 263K scrapie hamster brain homogenate. Normal brain controls are shown in red for the RT-QuIC. From these curves, estimates of the SD50 and LD50 can be made, however, we typically make these estimates using Spearman-Karber analyses of the data.Citation44 In this example (but not all sample types), the dilutions containing an SD50 and an LD50 were similar, indicating similar sensitivities of the RT-QuIC and bioassays for these samples. However, whereas the RT-QuIC assay takes ~2 d, the bioassay take 6–12 mo for determining end-point dilutions. Adapted with permission from Wilham et al.Citation6
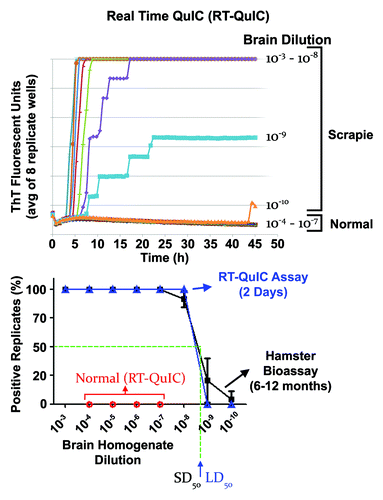
We have also used end-point dilution RT-QuIC to assess the time course of accumulation of prion seeding activity in the CSF of hamsters inoculated with scrapie by either the intracerebral or intratongue routes.Citation19 After intracerebral inoculation, seeding activity appeared in CSF within 1 d, decreased for several days, and then climbed ~100-fold before plateauing at ~30 d, prior to the onset of clinical signs at 60 d. In this case, it seems likely that the initially detected seeds were from the inoculum but that the later surge in seeding activity was due to prions propagating in the host. In contrast, with intratongue inoculations where seeds are not inoculated directly into the CNS, seeding activity was first detected in CSF near the onset of clinical signs of disease at ~85 d, well after seeds had accumulated to much higher levels in the brain tissue. These results call into question the likelihood that RT-QuIC analyses of CSF samples will allow preclinical detection of scrapie infections originating in peripheral, non-CNS sites, at least in this animal model.
eQuIC: capturing and detecting prion seeds from dilute and/or inhibitor-laden samples
Although RT-QuIC is suitable for detecting prion seeding activity in a variety of specimens, it can be inhibited by components of some types of samples such as whole blood, blood plasma or blood-contaminated tissues. To circumvent this problem and to pursue a blood test for prions, we have incorporated an immunoprecipitation step onto the front end of the RT-QuIC reaction (). We also improved the sensitivity with the addition of a rPrP substrate replacement step during the RT-QuIC reaction as has been shown to be helpful in PMCA reactions.Citation10 The combination of these two assay modifications is what we call eQuIC.Citation8 For this purpose we employed the monoclonal IgM antibody 15B3 which is selective for PrPSc and other abnormal PrP oligomers over monomeric PrPC.Citation20,Citation21 The antibody is coupled to anti-IgM coated magnetic beads and incubated with the test sample. This method has been developed using primarily ~0.5 ml of either spiked or naturally infected blood plasma.Citation8 The beads are then washed, treated, and added directly to reaction wells for RT-QuIC. After 24h, 90% of the liquid is removed, leaving most of the beads and nascent seeded amyloid in the well, and fresh rPrP substrate is added to continue the reaction.
Figure 2. eQuIC diagram and data. In eQuIC, prion seeds are immunoprecipitated with 15B3-coated beads and the beads are added to the reaction wells to initiate the reaction. After 24 h, the substrate is replaced and prion-seeded reactions usually turn ThT-positive within several hours (if not earlier).
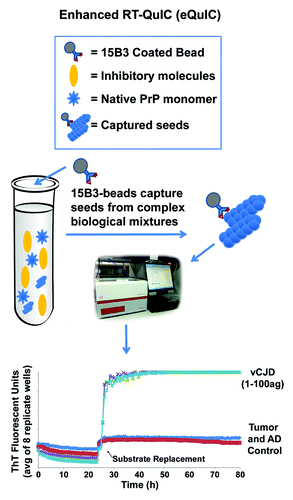
The resulting eQuIC assay has proven to be several orders of magnitude more sensitive than RT-QuIC alone. For instance, up to 1014-fold dilutions of vCJD brain homogenates in human plasma, containing less than ~1 ag of PrPRes have been detected.Citation8 This is ~10,000-fold more sensitive than previously reported assays for dilutions of vCJD brain homogenateCitation22 and is similar to the sensitivity limit for hamster PrPRes using serial PMCA.Citation2 Sensitivities in the 1–100 ag range have also been achieved in eQuIC assays of sheep, hamster and murine scrapie PrPRes (C.D. Orrù, A.Hughson and S. Vascellari, unpublished data). An attogram is equivalent to the mass of ~20 PrP molecules, which is near the number of molecules estimated to comprise the most infectious (per unit protein) scrapie particle.Citation23 However, we note that estimates of PrPRes content in TSE brain homogenates by immunoblotting are rough and dependent on proteinase K digestion conditions. Furthermore, it is possible that the majority of seeding activity is often associated with forms of PrPSc that are more proteinase K-sensitive than those typically detected as PrPRes. These factors make it difficult to use presently available data to determine the minimum number of seed particles or molecules of PrPSc required to initiate positive RT-QuIC or serial PMCA reactions.
eQuIC can readily detect prion seeds naturally present in the blood of scrapie-infected hamstersCitation8 and sheep (unpublished data) as indicated by the discrimination of plasma samples from infected and uninfected controls. Comparisons of eQuIC reactions using beads lacking the 15B3 antibody showed that the antibody improved the sensitivity of the assay by several orders of magnitude.Citation8 Collectively, the blood plasma data provide evidence that eQuIC can serve as the basis for a blood test for prion infections. Other in vitro assays have also detected prions endogenous to the blood of infected individuals.Citation10,Citation11,Citation22,Citation24 Further testing will be required to determine if approaches similar to eQuIC can be applied successfully to the detection of minute concentrations of prions in other types of samples, including those containing substances that are otherwise incompatible with prion-seeded amplification reactions such as RT-QuIC and PMCA.
Conclusions
Recent advances in assays based on prion-seeded rPrP amyloid formation have considerably improved their sensitivity and practicality. Initial evaluations of CSF-based RT-QuIC for sCJD diagnosis have indicated at least comparable sensitivity (85–90%) and substantially better specificity (100%) than tests for other markers.Citation12,Citation14 Given that the eQuIC is several orders of magnitude more sensitive than the RT-QuIC, it will be important to test if eQuIC might push the diagnostic sensitivity for sCJD closer to 100%. Another major goal at this point is to determine whether eQuIC can also detect prion seeds naturally occurring in the blood of humans infected with vCJD. If so, such testing of blood could help prevent further transfusion-based transmissions of vCJD. As demonstrated by the analyses of CSF and nasal fluids,Citation6,Citation16,Citation18 the highly sensitive, quantitative, relatively rapid and high-throughput attributes of end-point dilution RT-QuIC should facilitate measurements of prion seeds in a wide variety of samples suspected of being contaminated with prions. In principle, an end-point dilution quantitation should also be possible with the more sensitive eQuIC assay, but additional work will be necessary to establish its reliability.
Ultimately, it will be important to have multiple ultrasensitive tests available for prion detection and TSE diagnostics. Given the dire implications of a diagnosis of TSE infection, the accuracy of such tests and the ability to confirm positive reactions with both repeats of the same test and the applications of other tests will be of paramount importance. The apparent rarity of actual TSE infections in most human and animal populations also makes it likely that even tests with very low false positive rates will yield more false positives than real positives in any surveillance of general, rather than clinically targeted, populations. Hopefully, the eventual availability of both primary and confirmatory tests will reduce false positive rates to manageable levels.
Acknowledgments
This work was supported by the Intramural Research Program of the NIAID, NIH. S. V. was partially supported by the Master and Back Program of the Regione Sardegna (Italy).
References
- Caughey B, Baron GS, Chesebro B, Jeffrey M. Getting a grip on prions: oligomers, amyloids, and pathological membrane interactions. Annu Rev Biochem 2009; 78:177 - 204; http://dx.doi.org/10.1146/annurev.biochem.78.082907.145410; PMID: 19231987
- Saá P, Castilla J, Soto C. Ultra-efficient replication of infectious prions by automated protein misfolding cyclic amplification. J Biol Chem 2006; 281:35245 - 52; http://dx.doi.org/10.1074/jbc.M603964200; PMID: 16982620
- Chen B, Morales R, Barria MA, Soto C. Estimating prion concentration in fluids and tissues by quantitative PMCA. Nat Methods 2010; 7:519 - 20; http://dx.doi.org/10.1038/nmeth.1465; PMID: 20512142
- Atarashi R, Moore RA, Sim VL, Hughson AG, Dorward DW, Onwubiko HA, et al. Ultrasensitive detection of scrapie prion protein using seeded conversion of recombinant prion protein. Nat Methods 2007; 4:645 - 50; http://dx.doi.org/10.1038/nmeth1066; PMID: 17643109
- Atarashi R, Wilham JM, Christensen L, Hughson AG, Moore RA, Johnson LM, et al. Simplified ultrasensitive prion detection by recombinant PrP conversion with shaking. Nat Methods 2008; 5:211 - 2; http://dx.doi.org/10.1038/nmeth0308-211; PMID: 18309304
- Wilham JM, Orrú CD, Bessen RA, Atarashi R, Sano K, Race B, et al. Rapid end-point quantitation of prion seeding activity with sensitivity comparable to bioassays. PLoS Pathog 2010; 6:e1001217; http://dx.doi.org/10.1371/journal.ppat.1001217; PMID: 21152012
- Orrú CD, Wilham JM, Hughson AG, Raymond LD, McNally KL, Bossers A, et al. Human variant Creutzfeldt-Jakob disease and sheep scrapie PrP(res) detection using seeded conversion of recombinant prion protein. Protein Eng Des Sel 2009; 22:515 - 21; http://dx.doi.org/10.1093/protein/gzp031; PMID: 19570812
- Orrú CD, Wilham JM, Raymond LD, Kuhn F, Schroeder B, Raeber AJ, et al. Prion disease blood test using immunoprecipitation and improved quaking-induced conversion. MBio 2011; 2:e00078 - 11; http://dx.doi.org/10.1128/mBio.00078-11; PMID: 21558432
- Colby DW, Zhang Q, Wang S, Groth D, Legname G, Riesner D, et al. Prion detection by an amyloid seeding assay. Proc Natl Acad Sci U S A 2007; 104:20914 - 9; http://dx.doi.org/10.1073/pnas.0710152105; PMID: 18096717
- Rubenstein R, Chang B, Gray P, Piltch M, Bulgin MS, Sorensen-Melson S, et al. A novel method for preclinical detection of PrPSc in blood. J Gen Virol 2010; 91:1883 - 92; http://dx.doi.org/10.1099/vir.0.020164-0; PMID: 20357038
- Trieschmann L, Navarrete Santos A, Kaschig K, Torkler S, Maas E, Schätzl H, et al. Ultra-sensitive detection of prion protein fibrils by flow cytometry in blood from cattle affected with bovine spongiform encephalopathy. BMC Biotechnol 2005; 5:26; http://dx.doi.org/10.1186/1472-6750-5-26; PMID: 16202155
- Atarashi R, Satoh K, Sano K, Fuse T, Yamaguchi N, Ishibashi D, et al. Ultrasensitive human prion detection in cerebrospinal fluid by real-time quaking-induced conversion. Nat Med 2011; 17:175 - 8; http://dx.doi.org/10.1038/nm.2294; PMID: 21278748
- Orrú CD, Caughey B. Prion seeded conversion and amplification assays. Top Curr Chem 2011; 305:121 - 33; http://dx.doi.org/10.1007/128_2011_184; PMID: 21678135
- Atarashi R, Sano K, Satoh K, Nishida N. Real-time quaking-induced conversion: A highly sensitive assay for prion detection. Prion 2011; 5:150 - 3; http://dx.doi.org/10.4161/pri.5.3.16893; PMID: 21778820
- Peden AH, McGuire LI, Appleford NE, Mallinson G, Wilham JM, Orru CD, et al. Sensitive and specific detection of sporadic Creutzfeldt-Jakob disease brain prion protein using real-time quaking induced conversion. J Gen Virol 2012; 93:438 - 49; http://dx.doi.org/10.1099/vir.0.033365-0; PMID: 22031526
- Bessen RA, Shearin H, Martinka S, Boharski R, Lowe D, Wilham JM, et al. Prion shedding from olfactory neurons into nasal secretions. PLoS Pathog 2010; 6:e1000837; http://dx.doi.org/10.1371/journal.ppat.1000837; PMID: 20419120
- Zanusso G, Ferrari S, Cardone F, Zampieri P, Gelati M, Fiorini M, et al. Detection of pathologic prion protein in the olfactory epithelium in sporadic Creutzfeldt-Jakob disease. N Engl J Med 2003; 348:711 - 9; http://dx.doi.org/10.1056/NEJMoa022043; PMID: 12594315
- Bessen RA, Wilham JM, Lowe D, Watschke CP, Shearin H, Martinka S, et al. Accelerated shedding of prions following damage to the olfactory epithelium. J Virol 2012; 86:1777 - 88; http://dx.doi.org/10.1128/JVI.06626-11; PMID: 22130543
- Orrù CD, Hughson AG, Race B, Raymond GJ, Caughey B. Time course of prion seeding activity in cerebrospinal fluid of scrapie-infected hamsters after intratongue and intracerebral inoculations. J Clin Microbiol 2012; http://dx.doi.org/10.1128/JCM.06099-11; PMID: 22238438
- Korth C, Stierli B, Streit P, Moser M, Schaller O, Fischer R, et al. Prion (PrPSc)-specific epitope defined by a monoclonal antibody. Nature 1997; 390:74 - 7; http://dx.doi.org/10.1038/36337; PMID: 9363892
- Biasini E, Tapella L, Mantovani S, Stravalaci M, Gobbi M, Harris DA, et al. Immunopurification of pathological prion protein aggregates. PLoS One 2009; 4:e7816; http://dx.doi.org/10.1371/journal.pone.0007816; PMID: 19915706
- Edgeworth JA, Farmer M, Sicilia A, Tavares P, Beck J, Campbell T, et al. Detection of prion infection in variant Creutzfeldt-Jakob disease: a blood-based assay. Lancet 2011; 377:487 - 93; http://dx.doi.org/10.1016/S0140-6736(10)62308-2; PMID: 21295339
- Silveira JR, Raymond GJ, Hughson AG, Race RE, Sim VL, Hayes SF, et al. The most infectious prion protein particles. Nature 2005; 437:257 - 61; http://dx.doi.org/10.1038/nature03989; PMID: 16148934
- Saá P, Castilla J, Soto C. Presymptomatic detection of prions in blood. Science 2006; 313:92 - 4; http://dx.doi.org/10.1126/science.1129051; PMID: 16825570
- Terry LA, Howells L, Hawthorn J, Edwards JC, Moore SJ, Bellworthy SJ, et al. Detection of PrPsc in blood from sheep infected with the scrapie and bovine spongiform encephalopathy agents. J Virol 2009; 83:12552 - 8; http://dx.doi.org/10.1128/JVI.00311-09; PMID: 19740979
- Thorne L, Terry LA. In vitro amplification of PrPSc derived from the brain and blood of sheep infected with scrapie. J Gen Virol 2008; 89:3177 - 84; http://dx.doi.org/10.1099/vir.0.2008/004226-0; PMID: 19008409
- Everest SJ, Ramsay AM, Chaplin MJ, Everitt S, Stack MJ, Neale MH, et al. Detection and localisation of PrP(Sc) in the liver of sheep infected with scrapie and bovine spongiform encephalopathy. PLoS One 2011; 6:e19737; http://dx.doi.org/10.1371/journal.pone.0019737; PMID: 21589864
- Gough KC, Baker CA, Rees HC, Terry LA, Spiropoulos J, Thorne L, et al. The oral secretion of infectious scrapie prions occurs in preclinical sheep with a range of PRNP genotypes. J Virol 2012; 86:566 - 71; http://dx.doi.org/10.1128/JVI.05579-11; PMID: 22013047
- Maddison BC, Rees HC, Baker CA, Taema M, Bellworthy SJ, Thorne L, et al. Prions are secreted into the oral cavity in sheep with preclinical scrapie. J Infect Dis 2010; 201:1672 - 6; http://dx.doi.org/10.1086/652457; PMID: 20402590
- Maddison BC, Baker CA, Rees HC, Terry LA, Thorne L, Bellworthy SJ, et al. Prions are secreted in milk from clinically normal scrapie-exposed sheep. J Virol 2009; 83:8293 - 6; http://dx.doi.org/10.1128/JVI.00051-09; PMID: 19494004
- Haley NJ, Mathiason CK, Zabel MD, Telling GC, Hoover EA. Detection of sub-clinical CWD infection in conventional test-negative deer long after oral exposure to urine and feces from CWD+ deer. PLoS One 2009; 4:e7990; http://dx.doi.org/10.1371/journal.pone.0007990; PMID: 19956732
- Haley NJ, Mathiason CK, Carver S, Zabel M, Telling GC, Hoover EA. Detection of chronic wasting disease prions in salivary, urinary, and intestinal tissues of deer: potential mechanisms of prion shedding and transmission. J Virol 2011; 85:6309 - 18; http://dx.doi.org/10.1128/JVI.00425-11; PMID: 21525361
- Kurt TD, Perrott MR, Wilusz CJ, Wilusz J, Supattapone S, Telling GC, et al. Efficient in vitro amplification of chronic wasting disease PrPRES. J Virol 2007; 81:9605 - 8; http://dx.doi.org/10.1128/JVI.00635-07; PMID: 17553879
- Mathiason CK, Hayes-Klug J, Hays SA, Powers J, Osborn DA, Dahmes SJ, et al. B cells and platelets harbor prion infectivity in the blood of deer infected with chronic wasting disease. J Virol 2010; 84:5097 - 107; http://dx.doi.org/10.1128/JVI.02169-09; PMID: 20219916
- Jones M, Peden AH, Prowse CV, Gröner A, Manson JC, Turner ML, et al. In vitro amplification and detection of variant Creutzfeldt-Jakob disease PrPSc. J Pathol 2007; 213:21 - 6; http://dx.doi.org/10.1002/path.2204; PMID: 17614097
- Jones M, Peden AH, Yull H, Wight D, Bishop MT, Prowse CV, et al. Human platelets as a substrate source for the in vitro amplification of the abnormal prion protein (PrP) associated with variant Creutzfeldt-Jakob disease. Transfusion 2009; 49:376 - 84; http://dx.doi.org/10.1111/j.1537-2995.2008.01954.x; PMID: 18980616
- Tattum MH, Jones S, Pal S, Collinge J, Jackson GS. Discrimination between prion-infected and normal blood samples by protein misfolding cyclic amplification. Transfusion 2010; 50:996 - 1002; http://dx.doi.org/10.1111/j.1537-2995.2010.02595.x; PMID: 20180925
- Murayama Y, Yoshioka M, Masujin K, Okada H, Iwamaru Y, Imamura M, et al. Sulfated dextrans enhance in vitro amplification of bovine spongiform encephalopathy PrP(Sc) and enable ultrasensitive detection of bovine PrP(Sc). PLoS One 2010; 5: e13152; http://dx.doi.org/10.1371/journal.pone.0013152; PMID: 20957174
- Fujihara A, Atarashi R, Fuse T, Ubagai K, Nakagaki T, Yamaguchi N, et al. Hyperefficient PrP Sc amplification of mouse-adapted BSE and scrapie strain by protein misfolding cyclic amplification technique. FEBS J 2009; 276:2841 - 8; http://dx.doi.org/10.1111/j.1742-4658.2009.07007.x; PMID: 19459939
- Gonzalez-Montalban N, Makarava N, Ostapchenko VG, Savtchenk R, Alexeeva I, Rohwer RG, et al. Highly efficient protein misfolding cyclic amplification. PLoS Pathog 2011; 7:e1001277; http://dx.doi.org/10.1371/journal.ppat.1001277; PMID: 21347353
- Rubenstein R, Chang B, Gray P, Piltch M, Bulgin MS, Sorensen-Melson S, et al. Prion disease detection, PMCA kinetics, and IgG in urine from sheep naturally/experimentally infected with scrapie and deer with preclinical/clinical chronic wasting disease. J Virol 2011; 85:9031 - 8; http://dx.doi.org/10.1128/JVI.05111-11; PMID: 21715495
- Chang B, Gray P, Piltch M, Bulgin MS, Sorensen-Melson S, Miller MW, et al. Surround optical fiber immunoassay (SOFIA): an ultra-sensitive assay for prion protein detection. J Virol Methods 2009; 159:15 - 22; http://dx.doi.org/10.1016/j.jviromet.2009.02.019; PMID: 19442839
- Colby DW, Wain R, Baskakov IV, Legname G, Palmer CG, Nguyen HO, et al. Protease-sensitive synthetic prions. PLoS Pathog 2010; 6:e1000736; http://dx.doi.org/10.1371/journal.ppat.1000736; PMID: 20107515
- Dougherty RM. Animal virus titration techniques. In: Harris RJC, editor. Techniques in experimental virology.New York: Academic Press, Inc.; 1964. p. 183-6.