Abstract
After some 60 years in research, a few months before my final retirement (there were a few temporary ones), the time has come to reminisce.
After some 60 years in research, a few months before my final retirement (there were a few temporary ones), the time has come to reminisce
My interest in medicine was imprinted early on, when, as a child, I was fascinated by the illustrations in an anatomical atlas, especially of reproductive organs. In my early teens I undertook dissection of chickens, of which my mother was tolerant not only because the residues could subsequently be converted into soup, but because she discerned in this activity the seed to my further development as a surgeon. However, following my encounter with Paul de Kruif’s “Microbe Hunters,” a romantic description of Robert Koch cloning bacteria on slices of boiled potato, Paul Ehrlich discovering the Magic Bullet and Pasteur attenuating rabies virus for vaccination, I became enthralled with research. When my father gave me a Zeiss microscope for my 15th birthday, I discovered the joy of observing the otherwise invisible, and within a few years a room in the attic of our Zürich home was transformed into a rudimentary lab in which, among others, I studied vital staining by acridine orange of the epithelial cells of the newt, at that time still indigenous to Zürich ponds. This resulted in my first publication, in 1953, in the Zeitschrift fuer Zellforschung,Citation1 which at that time, believe it or not, even paid a honorarium for contributed articles.
Concurrently I studied medicine, eventually earned an MD, and even practiced as substitute for a country doctor in Gossau, a village near Zürich. My experiences persuaded me that mankind would be better served if I dedicated myself to research and so I went on to acquire a PhD degree in organic chemistry in 1961, working on curare alkaloids with Paul Karrer (), and eventually became a postdoc with Severo Ochoa in the Department of Biochemistry, New York University, where I worked from 1961 to 1967 on the replication of phage Qß phage, a plus-strand RNA virus (). The major achievement, in collaboration with Piet Borst, my erstwhile postdoc and lifelong friend, was the discovery that the RNA replication intermediate was not a double-stranded RNA as commonly believed, but a single-stranded RNA minus strand. The clinching experiment was the demonstration that highly purified minus strands (difficult to obtain prior to the advent of recombinant DNA technology) were non-infectious but could serve as templates for the cell-free synthesis of infectious plus strands by Qbeta replicase. The resulting paperCitation2 resolved in my favor a long-lasting, painful controversy with Sol Spiegelman, one of the most imaginative (some would say too imaginative) molecular biologists of the sixties, who for many years pushed the erroneous idea of a direct, plus-to-plus strand synthesis by Qbeta replicase, the enzyme he had discovered.
Figure 1. Paul Karrer, Professor of Chemistry at the University of Zürich. He received the Nobel Prize in Chemistry 1937 for his structural elucidation of carotenoids, flavins and vitamins A and B2; he was my thesis advisor 1959–1961 and guided my work on curare alkaloids, his last major interest.
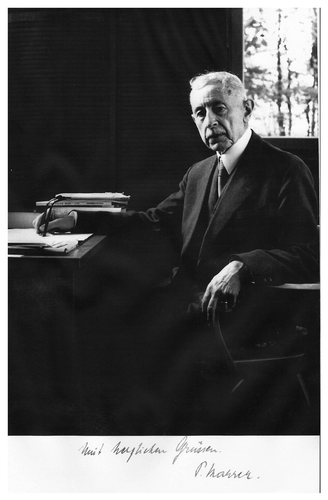
Figure 2. Severo Ochoa. While Professor of Biochemistry at the New York University School of Medicine, he discovered polynucleotide phosphorylase, which enabled the synthesis of polyribonucleotides, and earned him a share of the Nobel Prize in Physiology or Medicine in 1959. His interest in biosynthesis of RNA led to my Qbeta phage project, which I pursued in his Department from 1961–1967. Here, from left to right, Piet Borst (later Director of the Netherlands Cancer Institute), Severo Ochoa, myself, Martin Billeter (later Professor of Molecular Biology at the University of Zürich) and Roy Burdon (to become Chair of Molecular Biology at Strathclyde University). In the foreground the wooden mallet with which frozen Qbeta-infected E.coli paste was reduced to thawable fragments, and on the table the scheme for Qbeta RNA replication that emerged from our work. New York, 1964.
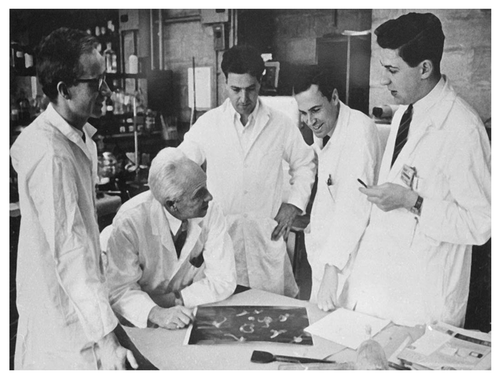
I returned to Zürich in 1967, as Director of the newly founded Institute of Molecular Biology (), and my group, building on my work in New York, undertook to enzymatically synthesize Qbeta RNA in a stepwise fashion. We incubated Qbeta replicase and template minus strand RNA with only three nucleoside triphosphates, so that synthesis stopped at the site where the missing substrate was required, separated the enzyme-template-product from the substrates and performed a second round of synthesis, this time adding the nucleoside triphosphate that had been missing in the first round, along with two others. This procedure, repeated several times, allowed the incorporation of 32P-labeled substrates into short, predetermined RNA segments, and by nearest neighbor analysis Martin Billeter and his colleagues, in 1969, determined the sequence of the first 175 nucleotides of Qbeta RNA,Citation3 for a short while the longest published sequence. Gratifyingly, Fred Sanger, in his Nobel speech, cited our stepwise synthesis as having inspired his plus/minus DNA sequencing strategy.
Figure 3. The Institute of Molecular Biology, 1967. The wooden barrack was erected on the premises of the Veterinary Hospital on the Irchel, Zürich, and housed the newly-founded Institute until 1970, when it moved to the ETH premises on the Hönggerberg. The entire staff, assembled on this cold winter morning, comprised (from left to right) our two technicians, Ueli Sulser and Leposova Andjelkov, Martin Billeter, Rose-Marie von Rotz, who was to remain my indispensable, loyal secretary for the next 30 years, and myself.
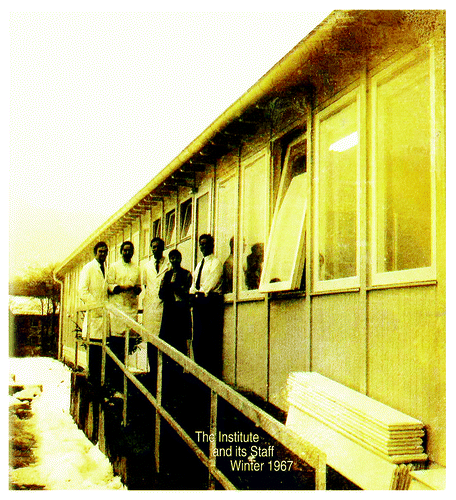
Stepwise synthesis also enabled us to insert nucleotide analogs into precise positions of the Qbeta genome, and in 1974 my postdoc Richard Flavell was the first to generate a site-directed mutation,Citation4 laying the groundwork of what I later termed reverse genetics, i.e., the procedure of modifying genetic material and ascertaining the resulting phenotype, as opposed to classical genetics, where a changed phenotype is identified and the responsible mutation is then tracked down.Citation5
In the course of exploring site-directed mutagenesis, we performed a control experiment which led us to the startling conclusion that a multiply passaged Qbeta phage population was highly heterogeneous with regard to its RNA sequence. We concluded that the population was in a dynamic equilibrium, with viable mutants arising at a high rate (about 10−4 per nucleotide and generation) on the one hand, and being strongly selected against on the other.Citation6 When I presented these results at Manfred Eigen’s annual Klosters meeting in 1977, he excitedly pointed out that this was exactly what he had predicted in his theory of quasispecies. Heterogeneity was subsequently found for many other virus populations and explains how they can rapidly acquire resistance against drugs, namely by selection of an inherently resistant variant initially present at undetectable levels.
In 1974 Tada Taniguchi joined my lab as a graduate student and succeeded in generating a complete double-stranded DNA copy of Qbeta RNA, integrated in a plasmid vector which, to our surprise and delight, when transfected into E.coli gave rise to infectious Qbeta phage, the first time such a feat was achieved,Citation7 enabling the application of recombinant DNA technology to RNA viruses.
On my return to Zürich, in 1967, I had also branched out to study Rous sarcoma virus replication. At that time, Temin’s hypothesis of reverse transcription was still held in wide contempt, and I was convinced that a search with appropriate techniques, of which I considered myself master, would yield an RNA minus strand. In the event it did not, and by the time I was ready to concede that there was less than one minus strand per infected cell, the bombshell of the 1970 discovery of reverse transcriptase by Temin and Baltimore burst over our heads. With John Coffin joining us from Temin’s lab, our group made several interesting contributions to the field, including the first demonstration that RSV RNA was synthesized by DNA-dependent RNA polymerase II, the mapping of the RSV genesCitation8 and the demonstration of terminal redundancy of Avian myeloblastosis virus RNACitation9 Nonetheless, the competition in the field had become so strong that I executed a strategic redeployment and turned to the study of eukaryotic transcription and promoter control, one of the challenges being that no eukaryotic genes had yet been cloned.
Richard Flavell and I had, in 1974, devised an imaginative strategy, whereby he (back in Amsterdam) would purify the globin DNA minus strand from EcoRI-cleaved, denatured rabbit chromosomal DNA by hybridizing it to β globin mRNA and recovering the hybrid on an oligo(dT) column, while I would pull out the DNA plus strand using a dC-elongated globin cDNA on an poly(I) Sephadex column (). On a memorable weekend we got together, hybridized our purified products and attempted to clone the dA-dT elongated product in pBR322. Alas, we obtained no clones and eventually found out that our product was highly inhibitory to transfection of E.coli, presumably due to all the junk we had accumulated along the way. With nothing to show for our efforts, we decided to at least have some fun at a Harden Conference in Wye College. I set forth our strategy; and when I got to the point of explaining how I was purifying the globin DNA plus strand, Richard, on cue, jumped up and exclaimed “Hey, I’m purifying the plus strand, you’re supposed to purify the minus strand.” This got a good laugh from the audience, but our bliss was complete when a breathless Nature correspondent approached us and asked; ‘how could you have done such a stupid thing?.” Unfortunately, she failed to get her report published.
Figure 4. Richard the rabbit. The invention of recombinant DNA technology in 1972 was followed by a regulatory tsunami aimed at reining in the perceived grave dangers of cloning DNA from another species. This photograph illustrates the fate suffered by Richard Flavell while attempting to isolate the rabbit globin gene.
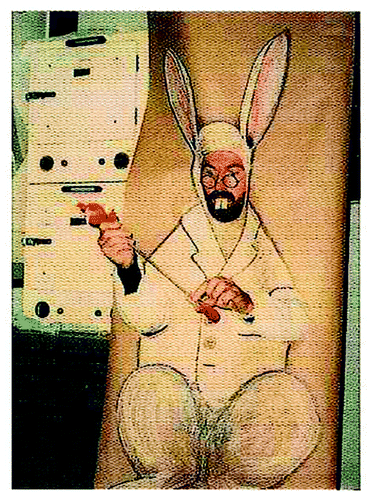
We did however put some of the technology to good use. Peter Curtis used dC-elongated globin cDNA to pull out 10S mature β globin mRNA along with what proved to be a 15S β globin RNA precursor from globin-producing Friend cells. We tried to determine whether the sequences present in the precursor but not in the mRNA were at its 5′ or 3′ end, but did not consider that they might be in the middle and thus missed discovering the large globin intron.
In 1977, at a Gordon Conference, I learned a lot about interferon from Peter Lengyel, in particular that induction of interferon (IFN) mRNA synthesis was very rapid, within hours of exposure to virus. I thought that studying the interferon gene promoter would be of great interest, but at that time not only was eukaryotic gene cloning in its infancy, but interferon itself had not been purified, antibodies to it were not available, and it could only be quantified by a laborious bioassay. Luckily, Paula Pitha had two years earlier shown that poly(A) RNA from virus-induced cells, when injected into Xenopus oocytes caused synthesis and secretion of biologically active interferon, and this experimental tool suggested to me a novel cloning strategy for IFN cDNA. We would prepare a bank of 5000 individual E.coli clones containing cDNA plasmids from interferon-producing cells, pool them in groups of 500 clones, fix the plasmid DNA from the pools to membranes, hybridize mRNA from interferon-producing cells to them, elute the RNA, inject it into Xenopus oocytes and assay for secreted interferon. If a pool gave a positive result, the constituent clones would be assembled in smaller groups, and so on, until a positive clone was identified.
At this point I would like to digress and survey the biotech landscape in 1977. The first biotech company aiming at the production of human proteins in bacteria was Genentech, founded by Swanson and Boyer in 1976. By 1977, Genentech expressed somatostatin in E.coli and the meagerly funded company gained an investment of $400'000 from INCO, the International Nickel Company. Genentech’s early success whetted INCO's appetite for biotech ventures and in Spring 1977 two INCO emissaries persuaded me to co-found a biotech company, later to be called Biogen, along with Wally Gilbert, Phil Sharp, Ken Murray and a number of other European molecular biologists and some venture capitalists.
Because Biogen could not afford to set up its own laboratory, I and other scientific board members undertook projects, fully funded by Biogen, in our own labs. My project proposal was for the cloning and expression of interferon cDNA. While I was quite confident that we would succeed in the cloning, there was no way of knowing at the time if biologically active eukaryotic protein could be produced in E.coli. It was only a year later that Genentech reported expression of human growth hormone in E.coli.
The reorientation of the IFN project toward a commercial objective entailed a switch from mouse to human IFN and my collaboration with Peter Lengyel was postponed in favor of a collaboration with Kari Cantell in Helsinki, who was producing crude IFN preparations from batches of virus-infected leukocytes pooled from 90'000 blood donors. Tada Taniguchi, and sometimes I, extracted poly(A) RNA from his “used” leukocytes and cloned the cDNA in E.coli. In 1978 Tada accepted a position at the Cancer Institute in Tokyo with the directive to clone interferon! We agreed that we in Zürich would work on “leukocyte interferon” while Tada would undertake the cloning of “fibroblast interferon” in Tokyo.
In the meantime developments within Biogen had become critical, the company was running out of money and was technically bankrupt. In the nick of time Moshe Alafi, a venture capitalist and co-founder of Biogen, persuaded Schering Plough to buy 16% of Biogen for 8 million dollars, giving the fledgling company a total value of 50 million dollars! Schering thereby acquired the rights to three projects, among which was IFN-α, which I was engaged in.
In 1979 Shige Nagata (), who succeeded Tada in the interferon project, began to pick up groups of bacterial clones which were scoring positive by the hybridization/translation assay and by the end of the year he isolated several individual positive clones, which, on a memorable Christmas day, proved to contain interferon activity. Within days we characterized the antiviral activity immunologically as being human leukocyte interferon, prepared a restriction map of the cDNA, filed a patent application on January 8, 1980, announced our achievement at a memorable press conference on January 16 () and followed up with a paper in NatureCitation10 describing the first production of biologically active human interferon in E.coli. A few months after our success Genentech and Hoffmann LaRoche, in a collaborative effort (which may well have made use of a widely circulated preprint containing the restriction map) also cloned and expressed IFN α; eventually, Schering Plough and Biogen shared the IFN α market. It is interesting to note that the huge interest in interferon had originally been raised by reports that it was effective in cancer treatment. Once interferon was available in sufficient amounts to carry out clinical trials on a larger scale these hopes were largely dashed; however, a rare blood disorder, hairy cell leukemia, could be successfully treated with interferon, which in 1986 led to FDA approval. After interferon was approved, it was tested for other uses and eventually pegylated IFNα, in combination with ribavirin, became the accepted treatment of hepatitis C and resulted in a billion dollar market.
Figure 5. Shige Nagata in action. Shige’s untiring efforts led to the first isolation of human α interferon cDNA, the cognate chromosomal gene family and the expression of biologically active interferon in E.coli. Here he posed for a photo that appeared in Life magazine. Note the state-of-the-art glass micropipette and the mouth-pipetting technique which earned us a tongue-in-cheek reproach from Phil Leder.
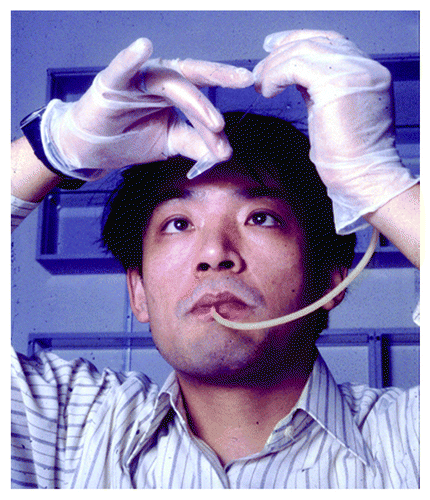
Figure 6. Cloning gold rush. Biogen called a press conference to announce that we had cloned and expressed human α interferon. Rather than garnering applause, I was criticized by both the press and my colleagues for participating in a commercial endeavor while employed by the University. A few years later many of my critical colleagues were following my example.
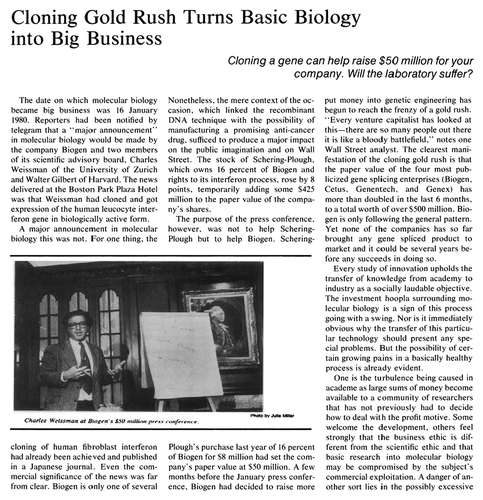
Once again, competition by superb scientists, including Tom Maniatis, Tada Taniguchi and Sydney Pestka, to mention just a few, persuaded me to search for greener pastures. In 1982, during the International Biochemistry Meeting in Perth, I dropped in on a talk by Stan Prusiner on scrapie, in which he reported the isolation of PrPSc (at that time simply called PrP, because he did not yet know that there were two forms of the protein), from scrapie-infected hamster brain. Because he had not found this protein in uninfected brain, he suggested that it was the infectious agent, and that there might be an as yet unknown mechanism which would allow this protein to be copied. I approached him after the talk and told him I couldn’t believe that a protein could be made without being encoded by a mRNA and that I was convinced that if one searched for it, it would be found. Stan was very open-minded about it and we started a collaboration, along with Lee Hood, who had sequenced the N-terminus of PrP.
I entrusted Bruno Oesch, a young post doc, with preparing and screening a cDNA bank of some 150’000 clones from scrapie-infected hamster brain with a set of degenerate primers prepared by Lee, which covered the N-terminus of PrP, and Bruno soon found a positive clone which contained the complete coding sequence of mature PrP. Using the cDNA as probe, we were astonished to find that not only scrapie-infected but also normal brain DNA contained a PrP gene. Even more surprisingly, PrP mRNA was present in uninfected brain tissue at the same level as infected brain, despite the fact that Stan had found PrP only in infected brain. The enigma was soon clarified by Stan’s group: PrP from infected brain was largely proteinase-K-resistant while PrP from uninfected brain (PrPC) was completely sensitive to the proteinase; because the first step in Stan’s purification procedure was based on digestion with proteinase K, his attempts to purify PrP from uninfected brain were unsuccessful. Using a newly acquired anti-PrP antibody, Stan found that both uninfected and infected brain contained PrP, but while the sample from infected brain was largely resistant to proteinase K, the PrP signal from uninfected brain was wiped out. The resulting seminal Cell paperCitation11 reported that, following scrapie infection, PrP was converted into a partially proteinase K-resistant form, designated PrPSc, which might be the infectious agent. Very soon thereafter, in record time, a brilliant diploma student, Konrad Basler (who years later succeeded me as chairman of the Institute), cloned and sequenced the chromosomal PrP gene.
Stan’s hypothesis, that the scrapie agent was a protein devoid of a nucleic acid genome, had from the outset, when he published his seminal Science paper in 1982, been greeted with scorn and antagonism. Much of the outrage was elicited by his naming the scrapie agent “prion,” for “proteinaceous infectious particles” on the basis of what was considered very preliminary evidence. Years later John Hardy congratulated Stan on inventing the term prion because in it Prusiner’s name would resonate for all times. He added that should he ever discover a particle causing Alzheimer disease he would call it a hardon….
At the time, the prevailing view was the scrapie agent contained a nucleic acid and was a virus, albeit an unusual one in view of its long incubation time and its resistance to sterilization, and was for many years termed “slow virus.” Stan’s lab provided several potent genetic arguments that indeed PrPSc was the major if not only component of the infectious agent, including the demonstration that the species barrier for prions could be overcome by introducing the PrP gene of the prion donor into the recipient and that familial Creutzfeldt-Jakob disease was linked to a mutation in the PrP gene.
In the late eighties it occurred to me that if we could eliminate PrP from the mouse it should become resistant to scrapie and incapable of replicating the agent. One of my very talented colleagues, Michel Aguet, using the techniques developed by Capecchi, Evans and Smithies, generated homozygous PrP knockout mice and we were astonished to find that they developed normally and were healthy. This was one of the first instances where the knockout of a mammalian gene had no readily discernable consequences and it earned the mouse a cover portrait in Nature.Citation12 We inoculated the knockout mice with massive doses of prions; months passed excruciatingly slowly, but gratifyingly the mice remained healthy and failed to propagate prion infectivity, showing that PrP is essential for generating the infectious agent and providing impressive support for the “protein only” hypothesis. The PrP knockout mouse was the first instance of an animal genetically engineered to be resistant to an infectious disease. We published these results in Cell in 1993,Citation13 and considering that the work had been conceived and performed in its entirety in Zürich I did not include Stan among the authors. This decision regrettably led to the end of our long-standing friendship.
While I lost -and missed - the association with Stan, I gained a collaboration with an enthusiastic young neuropathologist, Adriano Aguzzi. We enjoyed many years of frictionless and rewarding collaborations, and when, in 1999, I retired from the University of Zürich () to pursue a post-professorial Fellowship in John Collinge’s lab in London, Adriano developed a meteoric career which rapidly propelled him to the Directorship of the Neuropathology Institute and led to his anointment as the Swiss “Mr. Prion.”
Figure 7. Grillfest. The happy look on my face testifies to the satisfaction of my life-long addiction to the famed Swiss “Kalbsbratwurst,” here at the annual “Grillfest” on the ETH campus on the Hönggerberg., summer 1998.
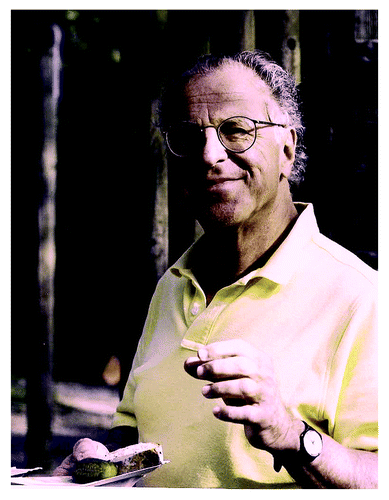
In London I took advantage of John’s generous hospitality and an MRC grant to build up a small group. I had come to the conclusion that the classical bioassay for prions, which consumed vast numbers of mice and took more than half a year to execute, was not an appropriate tool for an aging scientist. With Peter Kloehn I developed a cell-based assay for prions, the “standard scrapie cell assay,” which took only 12 days to complete, was far more accurate than the bioassay, allowed partial robotization and a throughput of hundreds of samples per week.Citation14
In the Fall of 2003 I was surprised by a phone call from Richard Lerner, then President of the Scripps Research Institute in LaJolla, inquiring whether I would be interested in heading a new Scripps institute to be established in Florida. By December I agreed to accept the position offered, which by then had shrunk to Chairmanship of a Department but provided very generous support. For some time I commuted between Florida and London, winding up the work in John’s Prion Unit and building up a group in the temporary lab space made available by Florida Atlantic University in Boca Raton. An enterprising postdoc, Sukhi Mahal, who had worked with me in London, accompanied me to Florida and developed the cell panel assay, which allowed the characterization of prion strains by their ability to infect four cell lines, as assessed by the standard scrapie cell assay. This enabled us to discover that prions were subject to mutation and selection in cultured cells, and resulted in what will have been my last Science paper.Citation15
As my eightieth birthday approached, I decided to retire while my departure still elicits expressions of regret rather than relief. I have noted that it is more rewarding to train students than to publish papers, because the half-life of a paper is three years, while that of a scientist is 30 years. Happily, I leave behind more than 100 former students, many of whom surpassed me in their accomplishments and some of whom may perpetuate the scientific tradition I inherited from my own teachers ().
Figure 8. Genealogy in science. Successful scientists are frequently the scientific offspring of other successful scientists, however, if there is a genetic component to Nobel predisposition (crown-like symbol), it is likely recessive. Only a few of my hundred-odd scientific offspring are listed (in alphabetical order), several of whom have outclassed me.
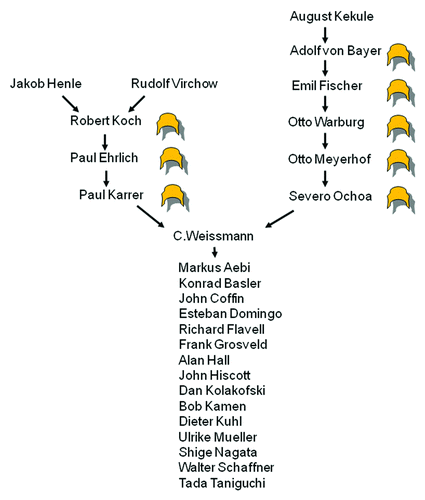
Acknowledgments
I thank the Jane Coffin Child Fund, the Swiss National Fund, the Department of Education of the Kanton of Zürich, the Medical Research Council, The Scripps Research Institute, the National Institutes of Health and the Alafi Family Foundation for generous support over the decades. I thank Donny Strosberg for critical reading of the manuscript.
References
- Weissmann C. [Vital staining of amphibian larvae with acridine orange]. Z Zellforsch Mikrosk Anat 1953; 38:374 - 408; http://dx.doi.org/10.1007/BF00339824; PMID: 13091052
- Feix G, Pollet R, Weissmann C. Replication of viral RNA, XVI. Enzymatic synthesis of infectious virual RNA with noninfectious Q-beta minus strands as template. Proc Natl Acad Sci U S A 1968; 59:145 - 52; http://dx.doi.org/10.1073/pnas.59.1.145; PMID: 5242118
- Billeter MA, Dahlberg JE, Goodman HM, Hindley J, Weissmann C. Sequence of the first 175 nucleotides from the 5′ terminus of Qbeta RNA synthesized in vitro. Nature 1969; 224:1083 - 6; http://dx.doi.org/10.1038/2241083a0; PMID: 5353716
- Flavell RA, Sabo DL, Bandle EF, Weissmann C. Site-directed mutagenesis: generation of an extracistronic mutation in bacteriophage Q beta RNA. J Mol Biol 1974; 89:255 - 72; http://dx.doi.org/10.1016/0022-2836(74)90517-8; PMID: 4444051
- Weissmann C. Reversed genetics. A new approach to the elucidation of structure-function relationships. Trends Biochem Sci 1978; 3:111
- Domingo E, Sabo D, Taniguchi T, Weissmann C. Nucleotide sequence heterogeneity of an RNA phage population. Cell 1978; 13:735 - 44; http://dx.doi.org/10.1016/0092-8674(78)90223-4; PMID: 657273
- Taniguchi T, Palmieri M, Weissmann C. QB DNA-containing hybrid plasmids giving rise to QB phage formation in the bacterial host. Nature 1978; 274:223 - 8; http://dx.doi.org/10.1038/274223a0; PMID: 355887
- Coffin JM, Billeter MA. A physical map of the Rous sarcoma virus genome. J Mol Biol 1976; 100:293 - 318; http://dx.doi.org/10.1016/S0022-2836(76)80065-4; PMID: 176365
- Stoll E, Billeter MA, Palmenberg A, Weissmann C. Avian myeloblastosis virus RNA is terminally redundant: implications for the mechanism of retrovirus replication. Cell 1977; 12:57 - 72; http://dx.doi.org/10.1016/0092-8674(77)90185-4; PMID: 198142
- Nagata S, Taira H, Hall A, Johnsrud L, Streuli M, Ecsödi J, et al. Synthesis in E. coli of a polypeptide with human leukocyte interferon activity. Nature 1980; 284:316 - 20; http://dx.doi.org/10.1038/284316a0; PMID: 6987533
- Oesch B, Westaway D, Wälchli M, McKinley MP, Kent SB, Aebersold R, et al. A cellular gene encodes scrapie PrP 27-30 protein. Cell 1985; 40:735 - 46; http://dx.doi.org/10.1016/0092-8674(85)90333-2; PMID: 2859120
- Büeler H, Aguzzi A, Sailer A, Greiner RA, Autenried P, Aguet M, et al. Mice devoid of PrP are resistant to scrapie. Cell 1993; 73:1339 - 47; http://dx.doi.org/10.1016/0092-8674(93)90360-3; PMID: 8100741
- Büeler H, Fischer M, Lang Y, Bluethmann H, Lipp H-P, DeArmond SJ, et al. Normal development and behaviour of mice lacking the neuronal cell-surface PrP protein. Nature 1992; 356:577 - 82; http://dx.doi.org/10.1038/356577a0; PMID: 1373228
- Klöhn P-C, Stoltze L, Flechsig E, Enari M, Weissmann C. A quantitative, highly sensitive cell-based infectivity assay for mouse scrapie prions. Proc Natl Acad Sci U S A 2003; 100:11666 - 71; http://dx.doi.org/10.1073/pnas.1834432100; PMID: 14504404
- Li J, Browning S, Mahal SP, Oelschlegel AM, Weissmann C. Darwinian evolution of prions in cell culture. Science 2010; 327:869 - 72; http://dx.doi.org/10.1126/science.1183218; PMID: 20044542