Abstract
Chronic wasting disease (CWD) is an invariably fatal neurologic disease that naturally infects mule deer, white tailed deer and elk. The understanding of CWD neurodegeneration at a molecular level is very limited. In this study, microarray analysis was performed to determine changes in the gene expression profiles in six different tissues including brain, midbrain, thalamus, spleen, RPLN and tonsil of CWD-infected elk in comparison to non-infected healthy elk, using 24,000 bovine specific oligo probes. In total, 329 genes were found to be differentially expressed (> 2.0-fold) between CWD negative and positive brain tissues, with 132 genes upregulated and 197 genes downregulated. There were 249 DE genes in the spleen (168 up- and 81 downregulated), 30 DE genes in the retropharyngeal lymph node (RPLN) (18 up- and 12 downregulated), and 55 DE genes in the tonsil (21 up- and 34 downregulated). Using Gene Ontology (GO), the DE genes were assigned to functional groups associated with cellular process, biological regulation, metabolic process, and regulation of biological process. For all brain tissues, the highest ranking networks for DE genes identified by Ingenuity Pathway Analysis (IPA) were associated with neurological disease, cell morphology, cellular assembly and organization. Quantitative real-time PCR (qRT-PCR) validated the expression of DE genes primarily involved in different regulatory pathways, including neuronal signaling and synapse function, calcium signaling, apoptosis and cell death and immune cell trafficking and inflammatory response. This is the first study to evaluate altered gene expression in multiple organs including brain from orally infected elk and the results will improve our understanding of CWD neurodegeneration at the molecular level.
Introduction
Chronic wasting disease (CWD) is a transmissible spongiform encephalopathy (TSE) affecting all members of the Cervidae family. Like other prion diseases, CWD is characterized by progressive dementia and/or ataxia.Citation1,Citation2 CWD has a long incubation period usually lasting from two to four years followed by the appearance of clinical symptoms including weight loss and behavioral changes lasting a few weeks to months.Citation3 Despite some similarities, CWD differs from ovine scrapie and bovine spongiform encephalopathy (BSE) in that it occurs in two different non-domestic deer and elk, and epidemiologic observations suggest its horizontal transmission within species.Citation4,Citation5 Although no definitive evidence has been found for higher levels of human TSE disease occurrence in CWD endemic areas where hunting and venison consumption are common,Citation6 research to define the potential of CWD to infect humans is ongoing.Citation7 A recent study has shown that cynomolgus macaques, which are evolutionarily close to humans, when exposed either orally or intracerebrally to CWD, did not develop any symptoms after six years of observation, although the direct relevance to humans is not definitive.Citation8 As the prevalence and distribution of CWD increases, there is also the risk that the disease may be transmitted to other species such as cattle, and this may result in increased risks to human health. Given these uncertainties and increasing CWD prevalence in North America, it is crucial to improve our understanding of the pathogenesis mechanisms of CWD disease.
Pathogenesis studies have revealed that disease associated prion proteins (PrPcwd or PrPd) are present in saliva, blood, urine, and feces.Citation9-Citation11 These prions are especially widespread in the tonsil and gut-associated lymphoid tissues, such as the spleen and lymph nodes, well before neuroinvasion, and they can occur in the absence of spongiform lesions or clinical signs, spreading to the central nervous system and spinal cord with concurrent distribution in peripheral lymphoid tissues from the early incubation phase until death.Citation12-Citation14 While physiological changes associated with TSE disease in the brain are well documented, the underlying molecular events involved in neurodegeneration are poorly defined. A number of studies using microarrays have identified global gene expression changes in TSE diseases such as scrapie in mouse and hamster models with identical genetic backgroundsCitation15-Citation20 and BSE in natural hosts, such as cattle.Citation21-Citation23 These studies have been useful for providing information about the involvement of multiple genes and identifying novel genes related to neurodegenerative pathway and pathogenesis mechanism including altered synapse function, regulation of apoptosis, stress response, and calcium ion homeostasis.Citation14 However, no report has analyzed gene expression changes associated with CWD, and more gene expression profiling studies are needed in TSE-infected animals with different genetic backgrounds.
Based upon established homology between domestic cow (Bos taurus) and white-tailed deer chromosomes,Citation24 a recent study by massively parallel pyrosequencing of a reduced representation library and a random shotgun library from white-tailed deer and using comparative contig overlay method showed a substantial number of high quality contigs (> 92%) were unambiguously aligned to the bovine genome assembly.Citation25 In this study, we used microarray analysis to profile gene expression changes occurring in CWD-infected tissues from orally inoculated elk. Because cervine microarray platform is not available due to insufficient cervid sequence data and genomic information, we used cross-species microarray analysis (i.e., elk samples hybridized to a bovine DNA microarray) to identify differentially expressed (DE) genes in different brain (brain, midbrain and thalamus), spleen, tonsil and RPLN tissues of CWD-diseased elk. The DE genes identified in response to CWD infection are important to understand the molecular mechanisms involved in neuropathology and prion replication.
Results
Differentially expressed genes in brain, spleen, RPLN and tonsil tissues from CWD-infected elk
Global gene expression variations were determined in tissues from the brain, midbrain, thalamus, spleen, RPLN and tonsil from control and CWD-infected elk using a bovine microarray representing 16,846 unique gene loci and 5,943 ESTs from the bovine genome. Microarray analysis identified 329 genes (132 upregulated and 197 downregulated) that were DE at least 2.0-fold in the different brain tissues comparing the CWD-negative and the CWD-positive animals (). Among these, 152, 65 and 112 genes were DE in the brainstem, midbrain and thalamus, respectively. shows there were 249 DE genes (168 upregulated and 81 downregulated) in the spleen, 30 DE genes (18 upregulated and 12 downregulated) in the RPLN, and 55 DE genes (21 upregulated and 34 downregulated) in the tonsil.
Table 1. Gene Ontology (GO) analysis of differentially expressed genes with ≥ 2.0 fold change, associated with CWD disease in brain tissues of elk
Table 2. Gene Ontology (GO) analysis of differentially expressed genes with ≥ 2.0 fold change, associated with CWD disease in spleen (SP), retropharyngeal lymph nodes (RPLN) and tonsil (TO) tissues of elk
Functional classification of DE genes
Overall, 122 DE genes from the brain tissues () and 110 DE genes from the spleen, RPLN and tonsil tissues () were associated with a function based on Gene Ontology (GO) analysis. The results showing categorization of DE genes from the GO analyses (modified Fisher Exact P-value < 0.05) include cellular process, biological regulation, metabolic process, regulation of biological process, multicellular organismal process, and development process ( and Table S1). According to the GO database, many DE genes fall into more than one category; however, for simplistic presentation, these genes have been presented under a single functional heading.
Figure 1.Functional classification of the differentially expressed genes in elk brain (brain, midbrain and thalamus), spleen, RPLN and tonsil tissues based on the Gene Ontology (GO) analysis.
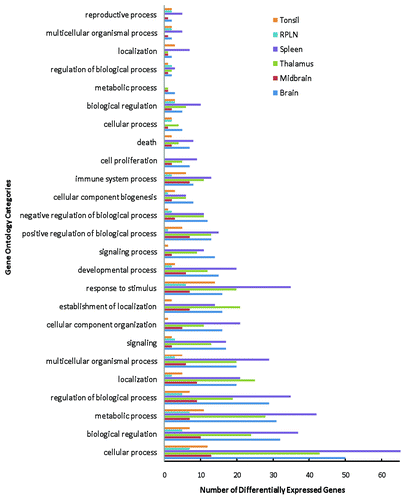
provides a comprehensive list of DE genes for the different brain tissues and the spleen, RPLN and tonsil tissues in CWD-infected elk. While some of these genes have been identified in previous studies of other TSE models (), a number of these genes are being reported for the first time (Table S4). Nonetheless, 86 DE genes identified in this study in response to CWD in elk have been identified in previously published studies of various TSE diseases ().
Table 3. Differentially expressed genes identified by previous studies of prion infected animals
We also used the Ingenuity Pathways Analysis (IPA) to annotate genes according to their functional relationships and to determine potential regulatory networks and pathways. provides the number of DE genes in the different brain tissues and in the spleen, RPLN and tonsil as identified by Genesifter analysis, and the Mapped IDs, network eligible genes and function eligible genes identified by IPA. The representative categories of biological functions/diseases of all of the DE genes in the different tissues using IPA are provided in . While neurological disease, genetic disorder, psychological disorder, skeletal disorders and cancer were the top five categories in both the brain and thalamus under “Diseases and disorders” in brain tissues, endocrine system disorder, metabolic disease, inflammatory response, genetic disease and inflammatory disease were the top functions in midbrain (). Among the DE genes in the brain tissues, cell morphology, cellular assembly and organization, cellular function and maintenance, free radical scavenging, cell signaling and molecular transport were the top molecular and cellular functions; the top physiological system development and function classifications were nervous system, skeletal and muscular system, connective tissue, behavior, tissue development and tissue morphology ().
Table 4. Summary of the number of DE genes which were found to be Network and Function eligible using Ingenuity Pathway Analysis (IPA) in comparison of control vs. infected of different tissues
Table 5. Summary of the highest represented functional categories (top 5) for the DE genes based on IPA analysis in brain, midbrain, thalamus, spleen, RPLN and tonsil tissues
The top functional categories for the DE genes were as follows: cancer, skeletal and muscular disorders, hematological disease, genetic disorder and reproductive system disease for the spleen tissues; genetic disorder, neurological disease, skeletal and muscular disorders, inflammatory response and renal and urological disease functional categories for RPLN tissues; and inflammatory response, reproductive system disease, infection mechanism, hypersensitivity response and connective tissue disorders for tonsil tissues under Diseases and disorders (). Cellular movement, cellular compromise, cellular assembly and organization, cellular function and maintenance, cell cycle, and cell death were the top molecular and cellular functions among DE genes for spleen, RPLN and tonsil tissues; the top physiological system development and function categories were immune cell trafficking and endocrine and hematological system development and function ().
Identification of biologically relevant networks
To investigate the global expression response to CWD and to define the interaction of individual regulated genes in specific pathways, potential networks of interacting DE genes were identified using IPA. All of the potential networks with a score > 9 (a score of 3 or greater is considered significant with p < 0.001) from different tissues have been provided in Additional File 3, Table S2, with information on the network genes, score, the focus genes and top functions associated with the focus genes in each network. For all of the brain tissues, the highest ranked network () identified by IPA was associated with neurological disease, cell morphology, cellular assembly and organization. This network contained genes (Table S2) that are primarily involved in apoptosis and cell death (26S proteasome, CDKN1C, MAPK3, MT1F, POLR2A, PRPH, NR4A2, RDX, SCIN, GNCA, SOD1, TAC1, TAX1BP1, and tubulin), signaling (actin, calmodulin, GNAS, SNAP25, SOD1, TAC1, and TAX1BP1), and cell movement, disassembly and differentiation (CAPZA2, ERMN, GDI, NEFH, and GPNMB). The highest ranking networks were cancer, cell death, and immune cell trafficking for the spleen, and infection mechanism for both the RPLN and tonsil tissues (Table S2). The top five canonical pathways for all of the brain tissues and the spleen, RPLN and tonsil tissues have been provided in Additional File 4, Table S3. Most of these pathways were related to signaling including endothelin-signaling, androgen signaling, synaptic long-term depression and CXCR4 signaling for the brain tissues; B cell development, antigen presentation pathway, disease signaling, and oxidative stress response for midbrain; and Parkinson signaling, OX40 signaling pathway, acute phase response signaling, IL-6 signaling for thalamus tissues. For the spleen, RPLN and tonsil tissues (Table S3), the most prominent pathways included the protein ubiquitination pathway, actin cytoskeleton signaling, aldosterone signaling, and IGF-1 signaling.
Figure 2. The highest scoring gene network based on classification of DE genes from elk brain tissues (brain, midbrain and thalamus) using the Ingenuity Pathway Analysis. The shape of the node indicates the major function of the protein and a line denotes binding of the products of the two genes. The genes in bold have been validated by qRT-PCR.
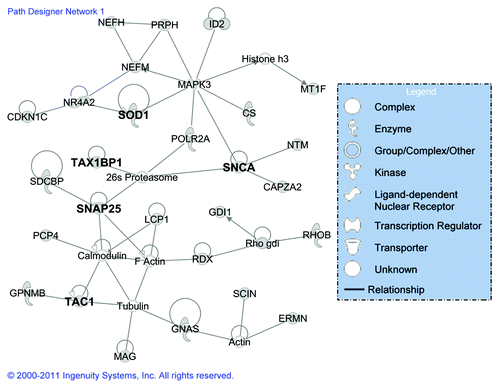
Quantitative real-time PCR validation of DE genes
Quantitative RT-PCR analyses were performed to validate the differential expression of several genes from different pathways including the following: neuronal signaling and synapse function in neurological disease [α-Synuclein (SNCA), Neuropeptide Y (NPY), Cocaine and amphetamine responsive transcript (CART), Synaptosomal associated protein 25 kDa (SNAP25) and Tachykinin 1 (TAC1)]; calcium ion regulation [Parvalbumin B (PVALB), Visinin-like 1 (VSNL1), Angiotensin II receptor (AGTRL1)]; apoptosis and cell death [Tax 1 binding protein 1 (Tax1BP1) and Superoxide dismutase (SOD1)]; and immune cell trafficking and inflammatory response (Chemokine 20 (CCL20), Serum amyloid A-like (SAA)].
All five genes, i.e., SNCA, NPY, CART, SNAP25 and TAC1, from the neuronal signaling and synapse function in neurological disease category showed reduced expression in both of the positive animals as compared with the control animals (), validating our microarray data. The extent of differential expression varied in the brain, midbrain and thalamus tissues from the two animals; however, the trend remained similar in both animals. Both of the genes associated with apoptosis and cell death, Tax1BP1 and SOD1, and three of the genes involved in calcium ion regulation, PVALB, VSNL1 and AGTRL1, showed decreased expression in all brain tissues from both animals (). A higher CCL20 expression was observed in the elk-infected spleen, RPLN and tonsil tissues (). We observed some inconsistency in SAA expression, which was increased in spleen, RPLN and tonsil tissues from one of the infected elk and only in RPLN in tissue from the other animal. A reduced expression was observed for AGTRL1 in the spleen, RPLN and tonsil tissues ().
Figure 3. Quantitative real-time PCR analysis of DE genes in elk brain tissues (A) and elk spleen, RPLN and tonsil tissues (B) in two different animals. DE genes associated with neuronal signaling include: α-Synuclein A (SNCA); Neuropeptide Y (NPY), Cocaine amphetamine responsive transcript (CART); Synaptosomal-associated protein 25KD (SNAP25), Tachykinin (TAC1); calcium ion regulation: Parvalbumin (PVALB), Visinin-like (VNSL1) and Angotensin II receptor (AGTRL); apoptosis: Human T-cell leukemia virus type I (Tax1BP1) and Superoxide dismutase (SOD1); immune and inflammatory response: Chemokine 20 (CCL20) and Serum amyloid A-like (SAA). Gene expression was compared between control and PRPCWD infected elk.
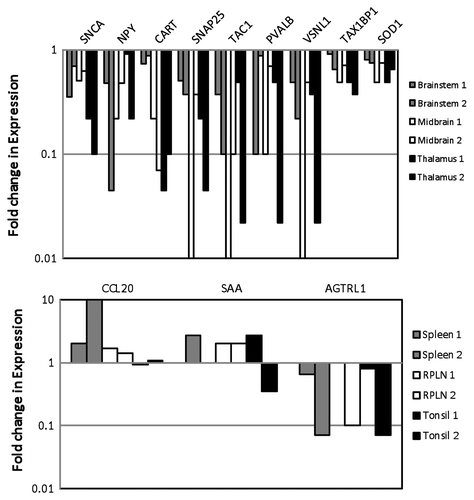
Discussion
Due to the increasing prevalence of CWD and the potential risk of its transmission to domestic livestock or humans, it is important to gain an understanding of CWD neurodegeneration at the molecular level. This study is the first comprehensive analysis of DE genes and pathways associated with CWD in elk including qRT-PCR validation of the differential expression of a set of key genes from different pathways from multiple organs of CWD infected elk. Eighty-six DE genes identified in this study in response to CWD in elk have also been identified in studies of other TSE diseases, suggesting the involvement of similar mechanisms, while 92 genes identified only in elk suggesting a host species specific mechanism may also be part of the pathogenesis mechanism.
Neuronal signaling and synapse function in neurological disease in elk brain tissues
Synapse loss has been identified as an early and critical pathophysiological event in neurodegenerative diseases.Citation26 The altered levels of a number of other compounds and neuropeptides that are important for normal neuronal function have also been noted for prion diseases. All DE genes related to the nervous system function and synaptic transmission identified in this study (11 DE genes listed in ) showed a reduced expression in infected animals as compared with control animals. Previous studies have shown that decreased CART and SNAP25 expressionCitation27,Citation20 and increased neuropeptide Y (NPY) and α-synuclein gene expression, are associated with prion infection in mice.Citation28,Citation29 The presynaptic protein α-synuclein has been reported to be involved in Creutzfield-Jacob disease (CJD) and in scrapie-infected hamsters through its role in prion protein accumulation and neurodegeneration in prion diseases.Citation29 Abnormalities in synaptic plasticity are believed to overlap with the deposition of PrPSc, which is one of the earliest features of prion disease.Citation30 Additionally, prion responsive genes in mice have been found to be associated with a number of signaling pathways particularly related to synaptic long-term potentiation and calcium signaling.Citation20 NPY is a neurotransmitter that is known to be associated with a number of physiological processes in the brain and has been shown to be overexpressed in a number of TSE experimental models, including mouse, and in Alzheimer disease.Citation28,Citation31,Citation32 Another neurotransmitter, CART, which is a peptide that has been implicated in a variety of brain functions including the protection offered by estradiol against ischemic brain injury in stroke and other neurodegenerative diseases,Citation33 showed differential expression between infected and control brain samples, and its reduced expression was validated by qRT-PCR. Sorensen et al.Citation20 detected a lower expression of CART in mice with prion infection in microarray studies, which is consistent with our studies.
Table 6. Oligonucleotides and probes used for qRT-PCR analysis of the DE genes
SNAP25 is a protein that is involved in neurotransmitter vesicle trafficking,Citation34 and a reduction in SNAP25 and a number of other crucial synaptic proteins have been observed in sporadic CJD,Citation26 and a lower expression of SNAP25 was found in all elk tissues. The reduced expression of TAC1, another neurotransmitter, was also observed in all brain tissues of CWD-infected animals. However, TAC1 differential expression has not been reported in previous mouse studies.Citation20,Citation35,Citation16,Citation19 All of the DE genes associated with neuronal signaling may play a role in the loss of function of neurons, and disruption of this system may be important for CWD-induced neurodegeneration in elk, leading to the irreversible pathogenesis.
Apoptosis and cell death in elk brain tissues
There has been strong evidence, based on several studies, that the apoptotic death of neurons is an underlying cause of the spongiform degeneration of the central nervous system in prion diseases.Citation26 However, whether this programmed cell death is occurring as an effect of PrPsc accumulation, a loss of functional PrPc or due to effects of the immune response remains unclear. Identification of six DE genes associated with apoptosis in CWD-infected elk, and further validation of a reduced expression of Tax1BP1 and SOD1 indicates the role of these genes in CWD disease in elk. The Tax1BP1 protein has the ability to deregulate the expression of a vast array of cellular genes,Citation36 and its DE was not reported in previous TSE studies. Among the pathological mechanisms of prion disease, neuroprotective and anti-apoptotic functions of PrPc are the most significant due to the anti-oxidant activity of PrPc37. Several experiments have shown the synergistic activity of PrPc and the SOD1 enzyme, and both are dependent on copper uptake and are involved in the protection against oxidative stress.Citation38 Other studies have reported a significant upregulation of the SOD1 gene in the brain stem of H-type BSE.Citation39
Calcium signaling in examined elk tissues
The altered regulation of calcium ion levels is proposed to be important in TSE-induced cell death.Citation40 The altered expression of calcium binding, transport and homeostasis genes has been observed in previous prion disease studiesCitation17,Citation20,Citation41,Citation42 and other neurodegenerative disorders, such as Down syndrome and Parkinson disease.Citation43,Citation44 Recent research has also demonstrated that neurodegeneration induced by a PrPsc-like prion protein peptide is the result of ER stress and calcium ion release, which results in cytosolic Ca2+ elevation, cytochrome c release, and apoptosis.Citation40 In this study, four DE genes were identified and two genes, Parvalbumin (PVALB) and Visinin-like 1 (VSNL1), which are related to modulation of the calcium ions levels, were further validated. PVALB is a calcium binding albumin protein present mainly in GABAergic interneurons in the nervous system that have been shown to influence the survival or death of neurons under pathological conditions.Citation45 VSNL1 is a neuronal Ca2+ sensor protein that modulates Ca2+-dependent cell signaling; however, its function in other tissues remains largely unknown.Citation46 The reduced expression of VSNL1 in CWD-infected elk provides support to a previous study that showed VSNL1 downregulation in brain tissues of scrapie-infected mice.Citation19 Research has shown the involvement of calcium binding proteins in neurologic disordersCitation35 through a possible calcium trafficking role of functional PrPc40; thus, calcium deregulation may be important in the events leading to the characteristic pathology observed in TSE diseases.
Immune and inflammatory response in elk spleen and RPLN tissues
A distinct immune/inflammatory response has been identified in previous TSE disease microarray studies.Citation15,Citation16,Citation18 It has been suggested that these results are due to microglial and astrocyte activation in response to neurodegeneration; however, some researchers believe that this activation is a key element in neurodegeneration.Citation26 Activation of these resident immune cells can trigger the release of proinflammatory cytokines, reactive oxygen species, proteases and complement proteins, all of which may be cytotoxic and lead to neurodegeneration.Citation44 Many studies have mentioned the involvement of the lymphoreticular cells in PrPSc accumulation, replication and transport from the peripheral lymphoid organs to the central nervous system,Citation48 and the detection of anti-PrP antibody at the terminal stage of mouse scrapie.Citation49 Investigating local immunity may be important to understand prion disease progression in the body. In addition, the contribution of inflammation to the progression of prion disease is poorly understood.
Serum amyloid (SAA) proteins are a family of apolipoproteins with biological functions that are not fully defined, although recent reports indicate that SAA induces proinflammatory cytokine expression.Citation50 SAA is mainly secreted by hepatocytes, and its concentration increases in the blood up to 1,000 times during an inflammatory response.Citation51 SAA expression was increased in all tissues from one infected elk and in the RPLN in the other animal. SAA has been associated with the top network of cancer and cell death in spleen tissues and with increased CCL20 expression, indicating its involvement in immune cell trafficking in spleen tissues (Table S1). A higher CCL20 expression was observed in elk-infected spleen tissues. The chemotactic actions of chemokines are known to be critical for the recruitment and activation of leukocytes, and they are important for the innate immune responders, such as neutrophils, dendritic cells and natural killer (NK) cells, as well as those cells involved in the adaptive immune response.Citation52 NK cells are capable of inducing enzyme-mediated apoptosis and may contribute to immune system-mediated apoptosis of CWD-infected neurons.
The Angiotensin-converting enzyme (ACE) cleaves angiotensin I to angiotensin II.Citation53 Variations in the ACE gene have been associated with Alzheimer disease,Citation54,Citation55 suggesting that ACE and ACE receptors may play a role in, or be affected by, neurodegenerative disease. Angiotensin II was downregulated in infected spleen, tonsil and RPLN tissues. Some of these genes could be considered presumptive biomarkers to distinguish CWD-infected elk from healthy animals; however, this needs to be assessed in further studies with more individuals.
Conclusions
CWD infects elk, white-tailed deer, and mule deer, and with no treatment or early diagnostic tests available, the disease is invariably fatal. Gene profiling studies data in naturally oral infected cervid TSE are also very limited. Thus, gaining a better understanding about the molecular mechanisms involved in CWD infection will potentially shed light on the many unknowns that are hampering the effective control and management of this disease. This is the first study to perform a high-throughput gene expression analysis to identify changes in the brain, spleen, RPLN and tonsil tissues of CWD disease-infected elk, which may prove useful in testing the accessible lymphatic tissues, such as the tonsils and RPLN, in preclinical animals.
Some of the DE genes identified have been previously reported in mice, cattle and humans suggesting that some common mechanisms associated with prion pathogenesis may exist among different host species. The differential expression of the Tax1BP1 gene, which is known to regulate the expression of a vast array of cellular genes, was not reported in previous TSE studies and needs to be confirmed in further studies with a larger number of animals. DE genes associated with major regulatory and signaling networks, including neuronal signaling, synapse function in neurological disease, calcium signaling, apoptosis and cell death, and immune cell trafficking and inflammatory response, from both microarray and qRT-PCR analyses provide further support for the utility of a bovine DNA microarray for gene expression profiling of elk samples. We are aware of the limitations of the study due to the lack of several biological replicates. However, high dispersion in the results is expected even when the biological replicates are individuals affected by evolution and natural selection depending on the gender, breed or environmental factors.Citation56 These results may also provide direction for other research focused on diagnosis, treatment and prevention of TSE diseases.
Methods
Animals and sampling
Rocky mountain elk (Cervus elaphus nelsoni) were selected from two farms in central Alberta, Canada. All animal screening, selection, inoculation and experiments were approved by the Canadian Food Inspection Agency (CFIA) and the Lethbridge Laboratory Animal Care Committee and followed the guidelines of the Canadian Council for Animal Care (CCAC). Two animals were selected for the negative inoculation control group, and two animals were selected for the CWD-infected group. All animals in the experiment were females, and they were inoculated at approximately 4 mo of age. The animals were kept in a biosafety level 3 facility for the remaining duration of the experiment. After a week of adjustment to the new environment, the animals were orally inoculated with 10 mL of a 10% brain homogenate (1 g tissue equivalent). The two control animals were fed normal elk brain tissue homogenate, while the CWD-infected group was fed brain tissue homogenate from confirmed CWD-positive elk.
Tissue collection, confirmation of PrPCWD infection and RNA extraction
Once clinical signs had reached a predetermined level, the animals were euthanized (overdose of pentobarbital injected intravenously in the jugular vein), and an extensive post mortem examination was performed. Animals no. 8 and 28 were euthanized 738 d post-inoculation. Control animals no. 6 and 31 were euthanized 752 d post-inoculation. Weights of the animals at euthanasia were as follows: no. 8, 450 lbs; no. 28, 485 lbs; no. 6, 610 lbs; and no. 31, 530 lbs.
All of the tissues (i.e., the brain stem, midbrain, thalamus, RPLN, tonsil and spleen) were collected as quickly as possible and placed in RNAlater (Ambion) to preserve the integrity of the RNA samples. After 24 h at 4°C, the RNAlater was removed from the samples, and the preserved tissues were stored at -80°C until further processing. Tissues from each of the animals were tested during routine surveillance by Prionics Check Priostrip and Prionics Check Western (Prionics AG), and the CWD status was confirmed by histology and immunohistochemistry.
Total RNA extraction from the infected and control tissues were performed using the Trizol/Chloroform protocol found in the Qiagen RNeasy extraction kit (Qiagen). The RNA quality and quantity were measured using a Nanodrop 1000 Spectrophotometer (Thermo Fisher Scientific), and the samples were stored at -80°C.
Microarray hybridization and data analysis
Global gene expression variations were determined by microarray analysis of two groups of control and CWD-infected animals (control animal no. 6 vs. infected animal no. 8 and control animal no. 31 vs. infected animal no. 28). Duplicates of 24,000 bovine oligonucleotide probes (www.Bovineoligo.org) representing 16,846 different genes, with 5,943 ESTs from the bovine genome, were spotted onto ultragap slides (Corning) using Q-array2 (Genetix). One microgram of total RNA was reversed-transcribed, amino-allyl coupled (Applied Biosystemsand labeled with Cy3 or Cy5 fluorescent dyes (GE Healthcare) according to the protocol of the manufacturer. For each tissue, four slides were used including dye swaps and two technical replicates. Thus, each gene was represented eight times in the statistical analysis. Hybridizations were performed in a hybridization chamber (Genetix) at 42°C overnight. Hybridized slides were washed with low stringency buffer [2x standard sodium citrate (SSC), 0.5% sodium dodecyl sulfate (SDS)], high stringency buffer (0.5x SSC, 0.2% SDS) and 0.05x SSC. The hybridized slides were scanned at 5 micron resolution and their signal intensities were detected by Q-Scan (Genetix).
Data analysis was performed using GeneSifter (VizX Labs). The background-corrected signal intensity for each spot was normalized by the locally weighted scatter plot smoothing (LOWESS) method and log base 2 transformed. Differences in the gene expression levels between control and CWD-infected tissues were analyzed using the t-test statistic method. The criteria for significant DE genes were a 2-fold or greater change in expression level with a p < 0.01, which was adjusted by the Bonferroni correction method.Citation57 The 95% quality filter was used to eliminate data from probes in any group that had an intensity variation between the probes greater than 5%.
Functional analysis of the DE genes
The characterization of the DE genes was performed by GeneSifter to annotate the GO of each gene into their respective biological and cellular processes and molecular function categories. The DE genes were also analyzed for their functions, pathways, and networks using IPA (Ingenuity Systems, http://www.ingenuity.com/products/IPA/Free-Trial-Software.html). Each gene identifier was mapped to its corresponding gene, called Focus genes, in the Ingenuity Pathways database, which was overlaid into a global molecular network. Networks of these Focus genes were then algorithmically generated to reveal their connectivity, and functional analysis of these networks identified their association with biological function and/or disease.
Quantitative real-time PCR analysis of DE genes
For technical validation of the microarray analysis, 12 DE genes were further tested using qRT-PCR. provides information on the targeted genes and the primer and probe sequences for each targeted gene. We tested four genes for endogenous controls, GPADH, 18S, Cyc and RPL. According to the Bestkeeper software,Citation58 RPL showed most stable expression and was selected as the endogenous control for subsequent analysis. RNA abundance was measured using the TaqMan Universal PCR Master Mix with gene specific MGB probes labeled with FAM and VIC fluorescent dyes (Applied Biosystems). Due to the limited amount of RNA, the samples were amplified using the reverse-transcribed synthesis amino-allyl kit (Applied Biosystems). The expression levels detected between total RNA and aRNA samples have been shown to be consistent.Citation59 Each reaction was performed in triplicate with 500 ng of aRNA using the StepOnePlus Real time PCR System (Applied Biosystems). The positive control (bovine cDNA) and the negative control (no template) control were always included on each plate. The thermal cycling conditions were as follows: 95°C for 20 sec followed by 40 cycles of 95°C for 1 sec and 60°C for 20 sec. The delta CT value for each gene was calculated by subtracting the reference gene CT from the targeted gene CT. Gene expression was compared between two control and two PrPCWD infected elk in three brain (brain, midbrain and thalamus) and spleen, RPLN, and tonsil tissues. The changes in gene expression between the control and infected animals were quantitatively measured relative to the RNA from the control sample. Relative quantification values were determined using 2-ΔΔCT method and were expressed as fold change in the infected vs. control animals.
Abbreviations: | ||
CWD | = | chronic wasting disease |
DE | = | differentially expressed |
PrP | = | prion protein |
TSE | = | transmissible spongiform encephalopathy |
Additional material
Download Zip (312.8 KB)Acknowledgments
Urmila Basu performed IPA analysis and wrote the manuscript; Luciane M. Almeida worked on microarray and qRT-PCR experiments and contributed to manuscript writing; Sandor Dudas was involved in RNA extraction, preliminary microarray experiments and contributed to manuscript writing. Catherine E. Graham and Stefanie Czub performed animal study and pathology study; Stephen S. Moore contributed to the discussion and manuscript writing; Le Luo Guan was involved in experimental design, data analysis and manuscript writing. This research was supported by PrionNet Canada, Alberta Prion Institute and Alberta Bovine Genomic Program.
Disclosure of Potential Conflicts of Interest
No potential conflicts of interest were disclosed.
References
- Prusiner SB. Prions. Proc Natl Acad Sci U S A 1998; 95:13363 - 83; http://dx.doi.org/10.1073/pnas.95.23.13363; PMID: 9811807
- Prusiner SB. Prion Biology and Diseases. 2004, 2nd edition, Cold Spring Harbor Laboratory Press. ISBN: 0879696931.
- Salman MD. Chronic wasting disease in deer and elk: scientific facts and findings. J Vet Med Sci 2003; 65:761 - 8; http://dx.doi.org/10.1292/jvms.65.761; PMID: 12939501
- Williams ES. The transmissible spongiform encephalopathies: disease risks for North America. Vet Clin North Am Food Anim Pract 2002; 18:461 - 73; http://dx.doi.org/10.1016/S0749-0720(02)00032-4; PMID: 12442578
- Williams ES. Chronic wasting disease. Vet Pathol 2005; 42:530 - 49; http://dx.doi.org/10.1354/vp.42-5-530; PMID: 16145200
- Anderson CA, Bosque P, Filley CM, Arciniegas DB, Kleinschmidt-Demasters BK, Pape WJ, et al. Colorado surveillance program for chronic wasting disease transmission to humans: lessons from 2 highly suspicious but negative cases. Arch Neurol 2007; 64:439 - 41; http://dx.doi.org/10.1001/archneur.64.3.439; PMID: 17353391
- Belay ED, Maddox RA, Williams ES, Miller MW, Gambetti P, Schonberger LB. Chronic wasting disease and potential transmission to humans. Emerg Infect Dis 2004; 10:977 - 84; http://dx.doi.org/10.3201/eid1006.031082; PMID: 15207045
- Race B, Meade-White KD, Miller MW, Barbian KD, Rubenstein R, LaFauci G, et al. Susceptibilities of nonhuman primates to chronic wasting disease. Emerg Infect Dis 2009; 15:1366 - 76; http://dx.doi.org/10.3201/eid1509.090253; PMID: 19788803
- Haley NJ, Seelig DM, Zabel MD, Telling GC, Hoover EA. Detection of CWD prions in urine and saliva of deer by transgenic mouse bioassay. PLoS One 2009; 4:e4848; http://dx.doi.org/10.1371/journal.pone.0004848; PMID: 19293928
- Tamgüney G, Giles K, Bouzamondo-Bernstein E, Bosque PJ, Miller MW, Safar J, et al. Transmission of elk and deer prions to transgenic mice. J Virol 2006; 80:9104 - 14; http://dx.doi.org/10.1128/JVI.00098-06; PMID: 16940522
- Tamgüney G, Miller MW, Wolfe LL, Sirochman TM, Glidden DV, Palmer C, et al. Asymptomatic deer excrete infectious prions in faeces. Nature 2009; 461:529 - 32; http://dx.doi.org/10.1038/nature08289; PMID: 19741608
- Gilch S, Chitoor N, Taguchi Y, Stuart M, Jewell JE, Schätzl HM. Chronic wasting disease. Top Curr Chem 2011; 305:51 - 77; http://dx.doi.org/10.1007/128_2011_159; PMID: 21598099
- Spraker TR, O’Rourke KI, Balachandran A, Zink RR, Cummings BA, Miller MW, et al. Validation of monoclonal antibody F99/97.6.1 for immunohistochemical staining of brain and tonsil in mule deer (Odocoileus hemionus) with chronic wasting disease. J Vet Diagn Invest 2002; 14:3 - 7; http://dx.doi.org/10.1177/104063870201400102; PMID: 12680636
- Huzarewich RLCH, Siemens CG, Booth SA. Application of “omics” to prion biomarker discovery. J Biomed Biotechnol 2010; 2010:613504; http://dx.doi.org/10.1155/2010/613504; PMID: 20224650
- Booth S, Bowman C, Baumgartner R, Sorensen G, Robertson C, Coulthart M, et al. Identification of central nervous system genes involved in the host response to the scrapie agent during preclinical and clinical infection. J Gen Virol 2004; 85:3459 - 71; http://dx.doi.org/10.1099/vir.0.80110-0; PMID: 15483264
- Xiang W, Windl O, Wünsch G, Dugas M, Kohlmann A, Dierkes N, et al. Identification of differentially expressed genes in scrapie-infected mouse brains by using global gene expression technology. J Virol 2004; 78:11051 - 60; http://dx.doi.org/10.1128/JVI.78.20.11051-11060.2004; PMID: 15452225
- Greenwood AD, Horsch M, Stengel A, Vorberg I, Lutzny G, Maas E, et al. Cell line dependent RNA expression profiles of prion-infected mouse neuronal cells. J Mol Biol 2005; 349:487 - 500; http://dx.doi.org/10.1016/j.jmb.2005.03.076; PMID: 15896347
- Riemer C, Neidhold S, Burwinkel M, Schwarz A, Schultz J, Krätzschmar J, et al. Gene expression profiling of scrapie-infected brain tissue. Biochem Biophys Res Commun 2004; 323:556 - 64; http://dx.doi.org/10.1016/j.bbrc.2004.08.124; PMID: 15369787
- Skinner PJ, Abbassi H, Chesebro B, Race RE, Reilly C, Haase AT. Gene expression alterations in brains of mice infected with three strains of scrapie. BMC Genomics 2006; 7:114 - 26; http://dx.doi.org/10.1186/1471-2164-7-114; PMID: 16700923
- Sorensen G, Medina S, Parchaliuk D, Phillipson C, Robertson C, Booth SA. Comprehensive transcriptional profiling of prion infection in mouse models reveals networks of responsive genes. BMC Genomics 2008; 9:114 - 28; http://dx.doi.org/10.1186/1471-2164-9-114; PMID: 18315872
- Khaniya B, Almeida L, Basu U, Taniguchi M, Williams JL, Barreda DR, et al. Microarray analysis of differentially expressed genes from Peyer’s patches of cattle orally challenged with bovine spongiform encephalopathy. J Toxicol Environ Health A 2009; 72:1008 - 13; http://dx.doi.org/10.1080/15287390903084199; PMID: 19697233
- Almeida LM, Basu U, Khaniya B, Taniguchi M, Williams JL, Moore SS, et al. Gene expression in the medulla following oral infection of cattle with bovine spongiform encephalopathy. J Toxicol Environ Health A 2011; 74:110 - 26; http://dx.doi.org/10.1080/15287394.2011.529061; PMID: 21218340
- Basu U, Almeida LM, Olson NE, Meng Y, Williams JL, Moore SS, et al. Transcriptome analysis of the medulla tissue from cattle in response to bovine spongiform encephalopathy using digital gene expression tag profiling. J Toxicol Environ Health A 2011; 74:127 - 37; http://dx.doi.org/10.1080/15287394.2011.529062; PMID: 21218341
- Gallagher DS Jr., Derr JN, Womack JE. Chromosome conservation among the advanced pecorans and determination of the primitive bovid karyotype. J Hered 1994; 85:204 - 10; PMID: 8014460
- Seabury CM, Bhattarai EK, Taylor JF, Viswanathan GG, Cooper SM, Davis DS. Dowd SE, Lockwood ML, Seabury PM. Genome-wide polymorphism and comparative analyses in the white-tailed eeer (Odocoileus virginianus): A model for conservation genomics. PLoS ONE 2011; 6:e15811; http://dx.doi.org/10.1371/journal.pone.0015811; PMID: 21283515
- Crozet C, Beranger F, Lehmann S. Cellular pathogenesis in prion diseases. Vet Res 2008; 39:44; http://dx.doi.org/10.1051/vetres:2008021; PMID: 18413130
- Sisó S, Puig B, Varea R, Vidal E, Acín C, Prinz M, et al. Abnormal synaptic protein expression and cell death in murine scrapie. Acta Neuropathol 2002; 103:615 - 26; http://dx.doi.org/10.1007/s00401-001-0512-6; PMID: 12012094
- Diez M, Groth D, DeArmond SJ, Prusiner SB, Hökfelt T. Changes in neuropeptide expression in mice infected with prions. Neurobiol Aging 2007; 28:748 - 65; http://dx.doi.org/10.1016/j.neurobiolaging.2006.02.017; PMID: 16621165
- Haïk S, Privat N, Adjou KT, Sazdovitch V, Dormont D, Duyckaerts C, et al. Alpha-synuclein-immunoreactive deposits in human and animal prion diseases. Acta Neuropathol 2002; 103:516 - 20; http://dx.doi.org/10.1007/s00401-001-0499-z; PMID: 11935269
- Johnston AR, Fraser JR, Jeffrey M, MacLeod N. Synaptic plasticity in the CA1 area of the hippocampus of scrapie-infected mice. Neurobiol Dis 1998; 5:188 - 95; http://dx.doi.org/10.1006/nbdi.1998.0194; PMID: 9848090
- Diez M, DeArmond SJ, Groth D, Prusiner SB, Hökfelt T. Decreased MK-801 binding in discrete hippocampal regions of prion-infected mice. Neurobiol Dis 2001; 8:692 - 9; http://dx.doi.org/10.1006/nbdi.2001.0404; PMID: 11493033
- Diez M, Danner S, Frey P, Sommer B, Staufenbiel M, Wiederhold KH, et al. Neuropeptide alterations in the hippocampal formation and cortex of transgenic mice overexpressing beta-amyloid precursor protein (APP) with the Swedish double mutation (APP23). Neurobiol Dis 2003; 14:579 - 94; http://dx.doi.org/10.1016/j.nbd.2003.08.003; PMID: 14678773
- Xu Y, Zhang W, Klaus J, Young J, Koerner I, Sheldahl LC, et al. Role of cocaine- and amphetamine-regulated transcript in estradiol-mediated neuroprotection. Proc Natl Acad Sci U S A 2006; 103:14489 - 94; http://dx.doi.org/10.1073/pnas.0602932103; PMID: 16971488
- Söllner T, Whiteheart SW, Brunner M, Erdjument-Bromage H, Geromanos S, Tempst P, et al. SNAP receptors implicated in vesicle targeting and fusion. Nature 1993; 362:318 - 24; http://dx.doi.org/10.1038/362318a0; PMID: 8455717
- Sawiris GP, Becker KG, Elliott EJ, Moulden R, Rohwer RG. Molecular analysis of bovine spongiform encephalopathy infection by cDNA arrays. J Gen Virol 2007; 88:1356 - 62; http://dx.doi.org/10.1099/vir.0.82387-0; PMID: 17374782
- Vajente N, Trevisan R, Saggioro D. HTLV-1 Tax protein cooperates with Ras in protecting cells from apoptosis. Apoptosis 2009; 14:153 - 63; http://dx.doi.org/10.1007/s10495-008-0289-3; PMID: 19089619
- Vana K, Zuber CH, Nikles D, Weiss S. Novel aspects of prions, their receptor molecules, and innovative approaches for TSE therapy. Cell Mol Neurobiol 2007; 27:107 - 28; http://dx.doi.org/10.1007/s10571-006-9121-1; PMID: 17151946
- Brown DR, Besinger A. Prion protein expression and superoxide dismutase activity. Biochem J 1998; 334:423 - 9; PMID: 9716501
- Larska M, Polak MP, Zmudzinski JF, Torres JM. Comparison of mRNA expression levels of selected genes in the brain stem of cattle naturally infected with classical and atypical BSE. Brain Res 2010; 1351:13 - 22; http://dx.doi.org/10.1016/j.brainres.2010.07.035; PMID: 20654596
- Ferreiro E, Oliveira CR, Pereira CM. The release of calcium from the endoplasmic reticulum induced by amyloid-beta and prion peptides activates the mitochondrial apoptotic pathway. Neurobiol Dis 2008; 30:331 - 42; http://dx.doi.org/10.1016/j.nbd.2008.02.003; PMID: 18420416
- Colling SB, Collinge J, Jefferys JG. Hippocampal slices from prion protein null mice: disrupted Ca(2+)-activated K+ currents. Neurosci Lett 1996; 209:49 - 52; http://dx.doi.org/10.1016/0304-3940(96)12596-9; PMID: 8734907
- Fuhrmann M, Bittner T, Mitteregger G, Haider N, Moosmang S, Kretzschmar H, et al. Loss of the cellular prion protein affects the Ca2+ homeostasis in hippocampal CA1 neurons. J Neurochem 2006; 98:1876 - 85; http://dx.doi.org/10.1111/j.1471-4159.2006.04011.x; PMID: 16945105
- Griffin WS, Sheng JG, McKenzie JE, Royston MC, Gentleman SM, Brumback RA, et al. Life-long overexpression of S100beta in Down’s syndrome: implications for Alzheimer pathogenesis. Neurobiol Aging 1998; 19:401 - 5; http://dx.doi.org/10.1016/S0197-4580(98)00074-8; PMID: 9880042
- Muramatsu Y, Kurosaki R, Watanabe H, Michimata M, Matsubara M, Imai Y, et al. Cerebral alterations in a MPTP-mouse model of Parkinson’s disease--an immunocytochemical study. J Neural Transm 2003; 110:1129 - 44; http://dx.doi.org/10.1007/s00702-003-0021-y; PMID: 14523625
- Voigtländer T, Unterberger U, Guentchev M, Schwaller B, Celio MR, Meyer M, et al. The role of parvalbumin and calbindin D28k in experimental scrapie. Neuropathol Appl Neurobiol 2008; 34:435 - 45; http://dx.doi.org/10.1111/j.1365-2990.2007.00902.x; PMID: 18005331
- Dai FF, Zhang Y, Kang Y, Wang Q, Gaisano HY, Braunewell KH, et al. The neuronal Ca+2 sensor protein-1 is expressed in pancreatic islet and regulates insulin secretion. J Biol Chem 2006; 281:21942 - 53; http://dx.doi.org/10.1074/jbc.M512924200; PMID: 16731532
- Chiarini A, Dal Pra I, Whitfield JF, Armato U. The killing of neurons by beta-amyloid peptides, prions, and pro-inflammatory cytokines. Ital J Anat Embryol 2006; 111:221 - 46; PMID: 17385278
- Montrasio F, Frigg R, Glatzel M, Klein MA, Mackay F, Aguzzi A, et al. Impaired prion replication in spleens of mice lacking functional follicular dendritic cells. Science 2000; 288:1257 - 9; http://dx.doi.org/10.1126/science.288.5469.1257; PMID: 10818004
- Sassa Y, Kataoka N, Inoshima Y, Ishiguro N. Anti-PrP antibodies detected at terminal stage of prion-affected mouse. Cell Immunol 2010; 263:212 - 8; http://dx.doi.org/10.1016/j.cellimm.2010.03.018; PMID: 20417929
- Cheng N, He R, Tian J, Ye PP, Ye RD. Cutting edge: TLR2 is a functional receptor for acute-phase serum amyloid A. J Immunol 2008; 181:22 - 6; PMID: 18566366
- Badolato R, Wang JM, Murphy WJ, Lloyd AR, Michiel DF, Bausserman LL, et al. Serum amyloid A is a chemoattractant: induction of migration, adhesion, and tissue infiltration of monocytes and polymorphonuclear leukocytes. J Exp Med 1994; 180:203 - 9; http://dx.doi.org/10.1084/jem.180.1.203; PMID: 7516407
- Esche C, Stellato C, Beck LA. Chemokines: key players in innate and adaptive immunity. J Invest Dermatol 2005; 125:615 - 28; http://dx.doi.org/10.1111/j.0022-202X.2005.23841.x; PMID: 16185259
- Coates D. The angiotensin converting enzyme (ACE). Int J Biochem Cell Biol 2003; 35:769 - 73; http://dx.doi.org/10.1016/S1357-2725(02)00309-6; PMID: 12676162
- Katzov H, Chalmers K, Palmgren J, Andreasen N, Johansson B, Cairns NJ, et al. Genetic variants of ABCA1 modify Alzheimer disease risk and quantitative traits related to beta-amyloid metabolism. Hum Mutat 2004; 23:358 - 67; http://dx.doi.org/10.1002/humu.20012; PMID: 15024730
- Lehmann DJ, Cortina-Borja M, Warden DR, Smith AD, Sleegers K, Prince JA, et al. Large meta-analysis establishes the ACE insertion-deletion polymorphism as a marker of Alzheimer’s disease. Am J Epidemiol 2005; 162:305 - 17; http://dx.doi.org/10.1093/aje/kwi202; PMID: 16033878
- Whitehead A, Crawford DL. Neutral and adaptive variation in gene expression. Proc Natl Acad Sci U S A 2006; 103:5425 - 30; http://dx.doi.org/10.1073/pnas.0507648103; PMID: 16567645
- Bland JM, Altman DG. Multiple significance tests: the Bonferroni method. BMJ 1995; 310:170; http://dx.doi.org/10.1136/bmj.310.6973.170; PMID: 7833759
- Pfaffl MW, Tichopad A, Prgomet C, Neuvians TP. Determination of stable housekeeping genes, differentially regulated target genes and sample integrity: BestKeeper--Excel-based tool using pair-wise correlations. Biotechnol Lett 2004; 26:509 - 15; http://dx.doi.org/10.1023/B:BILE.0000019559.84305.47; PMID: 15127793
- Taniguchi M, Guan LL, Zhang B, Dodson MV, Okine E, Moore SS. Adipogenesis of bovine perimuscular preadipocytes. Biochem Biophys Res Commun 2008; 366:54 - 9; http://dx.doi.org/10.1016/j.bbrc.2007.11.110; PMID: 18060854