Abstract
Early cell biologists perceived centrosomes to be permanent cellular structures. Centrosomes were observed to reproduce once each cycle and to orchestrate assembly of a transient mitotic apparatus that segregated chromosomes and a centrosome to each daughter at the completion of cell division. The molecular picture of centrosomes is now more complete. Centrosomes are composed of a pair of centrioles buried in a complex pericentriolar matrix. The bulk of microtubules in cells lie with one end buried in the pericentriolar matrix and the other extending outward into the cytoplasm. Centrioles recruit and organize pericentriolar material. As a result, centrioles dominate microtubule organization and spindle assembly in cells born with centrosomes. Centriole inheritance is ensured by cell cycle checkpoints that monitor the presence of centrosomes in G1 and spindle bipolarity in mitosis. In spite of these cell cycle controls, assembly of bipolar spindles in acentriolar cell extracts and cell proliferation in acentriolar mutants argue that neither centrioles nor centrosomes are essential structures. This review examines the recent advances in understanding centriole inheritance.
Introduction
Theodor Boveri (1862–1915) was among the first biologists to recognize the importance of centrosomes to inheritance of genetic information.Citation1,Citation2 Boveri and his contemporaries accepted that cells divide by binary fission and that chromosomes provide the genetic basis of inheritance. How the thread like chromosomes in the nucleus of the parental cell are transmitted to the two daughter cells was a mystery. Through careful observations with the crudest of microscopes by today's standards, but the keenest of insights, Boveri defined the centrosome () as a pair of centrioles surrounded by a special material (pericentriolar matrix) that organized the surrounding cytoplasm and formed a “division center” (microtubule aster). Boveri argued that the centrosome was the “reproductive organ” of the cell, orchestrating assembly of a transient mitotic apparatus (spindle) that segregated bipartite chromosomes (sister chromatids) to each daughter cell. Central to his argument, centrosomes are permanent cellular structures that have the capacity to reproduce and divide once per cell cycle. He used simple tricks to generate cells with one or more centrosomes, finding a half-spindle organized by a single centrosome and multipolar spindles organized by a centrosome at each pole. He argued that the number of centrosomes determined the number of spindles poles. The Centrosome Theory formulated by Boveri met resistance; not all cells have centrosomes, but all cells do assemble spindles.
A landmark study provided proof of principle that centrosomes are not essential for spindle assembly.Citation3 Xenopus eggs lack centrioles, but contain materials needed for many rounds of centrosome assembly. When chromatin coated beads are substituted for chromosomes, microtubules emerge from the beads, nucleated by members of a family of plus-end binding proteins that add tubulin at microtubule plus-ends, effectively pushing microtubule minus-ends outward. Microtubules are eventually bundled into spindle poles by microtubule dependent motor proteins. Spindle assembly in acentriolar Xenopus egg extracts suggests that cells have a default pathway to spindle assembly. The significance of these results are supported by studies showing that the components and molecular mechanisms that promote self-assembly of spindles in Xenopus extracts operate in vivo as well.Citation4,Citation5 The existence of a default pathway to spindle assembly begs the question of why centrosomes are conserved structures in virtually all animal cells.
Centrioles accumulate pericentriolar material and as a result, dominate microtubule organization in cells. Centrioles have a highly conserved nine-fold axis of symmetry (), most often formed by triplets of microtubules in a pinwheel. Centrioles are virtually identical to the core of basal bodies that organize the axonemes of cilia and flagella. Defects in basal body function have profound effects on cell signaling during development and human health,Citation6 but space limitations preclude further discussion here on this important topic. This review examines the recent literature on inheritance of centrioles in light of recent debate on the need, or lack thereof, for centrioles in spindle assembly and completion of mitosis.
The Perceived Function of Centrioles in Mitosis
Microtubule organizing centers.
Expectations of centrosome activity and the corresponding role historically ascribed to centrioles are rooted in microtubule dynamics and the perceived role of centrosomes in microtubule nucleation. Microtubules are closed tubes of ∼13 protofilaments of tubulin monomers, α/β-tubulin heterodimers, in head-to-tail polymers held together by noncovalent interactions.Citation7 As a result microtubules constantly turn over in cells, changing both in length and composition. Tubulins are GTP binding proteins and polymerization into growing microtubules requires that both α- and β-tubulins are bound to GTP. Crystallography of α/β-tubulin bound to GTP shows a straight conformation that is thought to promote the longitudinal interactions necessary for polymerization.Citation8 GTP bound to β-tubulin subsequently undergoes hydrolysis, resulting in a more unstable lattice.Citation9 Tubulin self-assembles into microtubules in vitro; the rate at which new microtubules form, designated as nucleation, depends heavily on the concentration of tubulin, GTP and microtubule associated proteins (MAPs) that lower the concentration of tubulin needed for self-assembly of comparable amounts of polymer. Until recently the concentration of tubulin in cells was considered too low to support the level of tubulin polymer found in cells by self-assembly, requiring a mechanism to renew the microtubules lost to depolymerization. Because the bulk of microtubules are organized by centrosomes, microtubule nucleation was inferred to be the essential activity of the pericentriolar matrix.
The pericentriolar matrix.
γ-tubulin was among the first pericentriolar proteins identified.Citation10 γ-tubulins show more structural diversity than α- and β-tubulins, but γ-tubulin is also ubiquitously expressed among eukaryotes and essential for viability. A portion of γ-tubulin in extracts of flies and vertebrates sediments in high molecular weight ring-like complexes, designated γTuRCs, with 5–6 other proteins (). The ring-like shape suggested that γTuRCs might provide a template for nucleation of microtubules.Citation10 However, the constituents are not conserved across species () and smaller complexes (γTuSCs) are also found in Drosophilae that do not have a ring-like structure. Crystals of γ-tubulin bound to a non-hydrolysable GTP analog showed a curved conformation that is most similar to α/β-tubulins incorporated into the walls of microtubules and in soluble monomers bound to GDP that cannot polymerize.Citation8 Localization to microtubule minus ends has received considerable attention,Citation11 but γ-tubulin is periodically positioned along the length of microtubules in fission yeastCitation12 and in land plants where it is needed for assembly of branched cortical microtubule arrays.Citation13,Citation14
Initial studies suggested that γ-tubulin is universally essential for microtubule nucleation, but it is now widely acknowledged that alternative pathways exist, in large part because abundant microtubules persist in γ-tubulin deficient mutants and in mutants deficient in other γTuRC components.Citation16–Citation21 Additional proteins implicated in nucleation of microtubules include orthologs of XMAP215 in Xenopus, a conserved MAP that promotes dramatic growth at microtubule plus-ends.Citation22–Citation24 Loss of the ortholog ZYG-9 in Caenorhabditis reduces the number of centrosomal microtubules to a level comparable to loss of α-tubulin and more severely than loss of γ-tubulin.Citation25 Reconstitution of microtubule asters with salt-stripped centrosomes requires XMAP215 in vertebrates.Citation26 XMAP215 bound to beads nucleated microtubule asters in dilute solution of pure tubulin as well as in cell extracts,Citation26 as expected of an authentic microtubule nucleator. In addition to γ-tubulin, glp-1 and ZYG-9, targeted RNAi screens in Caenorhabditis for genes involved in microtubule nucleation and growth identified proteins known to interact with ZYG-9, proteins previously identified in other pathways and novel proteins that have not yet been fully analyzed.Citation25 Similar studies in vertebrate cells and flies have implicated additional proteins in centrosome function. An outstanding question in all cases is whether the perceived effect on microtubule nucleation is a direct or indirect effect on centrosome organization.
Centriole Assembly and Acquisition of Pericentriolar Material
Centriole function and assembly.
Centrioles organize pericentriolar components into a discrete focus. The mechanism is a mystery, but the effect is evident in the mouse blastocyst where centrioles do not appear until the second or third mitosis,Citation27 coinciding with reorganization of pericentriolar components into the characteristic dense spherical mass of canonical centrosomes. At least some pericentriolar proteins are incorporated into centriole barrels; γ-tubulin is essential for centriole assembly as well as assembly of the central pair of microtubules that emerge from the transition zone in basal bodies ().Citation28,Citation29 Accumulation of γ-tubulin at centrioles in Caenorhabditis is mediated by another pericentriolar protein, the spindle defective protein SPD-5.Citation30 RNAi inactivation of either SPD-5 or γ-tubulin reduced centriole assembly to ∼40–50% of the number expected.Citation31 SPD-5 may serve only to recruit γ-tubulin since incorporation into centriole barrel has not been reported. An essential role for γ-tubulin in centriole assembly is significant because loss of γ-tubulin function disrupts centrosome organization across species. Severe γ-tubulin mutants generate malformed spindles, including monopolar structures that follow inward collapse of spindle poles in CaenorhabditisCitation18,Citation19 and fusion of nearby spindles in γ-tubulin deficient Drosophila embryos ().Citation17,Citation32 Thus, γ-tubulin function in microtubule organization and spindle bipolarity reflects, at least in part, its role in centriole assembly and the centriole's role in recruiting and organizing pericentriolar components.
γ-Tubulin was the first of an extended family of tubulin isoforms that proved to be associated with centriole and basal bodies. Almost a decade following discovery of γ-tubulin, genetic and/or genomic approaches identified δ-, ε-, η- and ζ-tubulins.Citation29 In contrast to γ-tubulin, the δ-, ε-, η- and ζ-tubulins have limited distribution among species (). Although little is known about η-, and ζ-tubulin function, basal bodies in Chlamydomonas and Paramecium mutants deficient in δ- and ε-tubulins are shortened and missing the B- and/or C-tubules and ε-tubulin has been implicated in centriole assembly.Citation29 Among other models for function,Citation34 δ- and ε-tubulins may promote stability of centrioles/basal bodies. Stabilizing function is consistent with restored triplet microtubules in δ-tubulin deficient Chlamydomonas mutants that also express an altered form of α-tubulin. This mutant form of α-tubulin stabilizes microtubules and δ- or ε-tubulins may stabilize triplet microtubules in the same way.Citation29 However, the precise role of any member of the extended tubulin family in centriole/basal body assembly is not yet known.
The centriole cycle and the spindle assembly checkpoint.
Centriole assembly is tightly controlled by the cell cycle, limiting the number of centrosomes and thereby promoting assembly of bipolar spindles. Each cell inherits a centriole pair at the completion of mitosis and cytokinesis of its parent (). Centrioles initially show an orthogonal orientation that is lost before assembly of a new centriole is initiated. A nascent centriole or procentriole appears during S-phase near the proximal end of the preexisting or parental centriole.Citation35 Centriole assembly initiates with formation of central tube-like structure,Citation36 followed by the appearance 9 singlet A microtubules in CaenorahditisCitation36 and sequential addition B and C tubules in other species. Procentrioles continue to elongate during G2, but typically do not acquire a significant mass of pericentriolar material or associated microtubules until the next cell cycle. Centrioles remain tethered in most cells until the G2/M transition into mitosis when the connection is severed, allowing centrosomes to separate and form a bipolar spindle.Citation35 If centriole duplication fails, the barren centrioles will separate and organize the poles of a bipolar spindle. A single centriole will be partitioned to each daughter by cytokinesis and these will organize a monopolar spindle in the following cell cycle (). In the absence of bipolar attachment of kinetochores, the spindle assembly checkpoint will arrest the cell cycle. Thus, the spindle assembly checkpoint is perceived as a backup mechanism to block proliferation of cells with defects in centriole duplication.
Genetic analysis of centriole assembly and duplication.
Initiation of centriole assembly requires a member of a conserved family of serine/threonine kinases, Plk-4 (polo kinase 4) in vertebrates and Sak in flies.Citation38 Plk-4 is centrosome associated throughout the cell cycle, but phosphorylation by Cdk2-CyclinA/E near the G1/S phase transition is needed for centriole assembly.Citation38 Overexpression of Plk-4 in cultured human cells generates several procentriole-like structures that encircle the proximal ends of the parental centriole,Citation39,Citation40 indicating that multiple procentrioles can form in association with a preexisting centriole. These results suggest that Plk-4/Sak is an essential positive regulator of centriole assembly.
Genetic studies in Caenorhabditis initiated a period of rapid advance in establishing the pathway of centriole assembly and the players involved. RNAi screens, chemical mutagenesis, and genomic approaches identified the novel zygotic defective ZYG-1 kinase, the spindle assembly proteins SAS-4, SAS-5, SAS-6 and the spindle defective protein SPD-2.Citation41 Localization of SPD-2 is the essential first step and precedes appearance of ZYG-1.Citation42 Caenorhabditis does not encode a Plk-4 homolog and it is not clear whether ZYG-1 provides the same or a different function. ZYG-1 activity is necessary for subsequent recruitment of SAS-5 and SAS-6 that are mutually dependent for localization to centrioles and necessary for subsequent recruitment of SAS-4. Formation of the central tube in forming centrioles requires SAS-5 and SAS-6 while assembly of nine singlet microtubules on the periphery of the central tube requires SAS-4.Citation36 Homologs of SAS-5 in other species have not been reported, but homologs of SAS-6 and SAS-4 humans and flies are also needed for centriole assembly. Controlled expression experiments in human cells indicate that turnover of HsSas-6 protein by the proteasome is needed to limit assembly in S-phase to a single centriole.Citation43
Putative homologs of SPD-2 in Caenorhabditis include DSpd-2 in DrosophilaCitation44,Citation45 and Cep192Citation46 in humans. Although localization indicates that each is a component of the pericentriolar matrix and centrioles, homology to SPD-2 is weak and limited to short segments of these long proteins that are dominated by coiled coil structure. The mutant phenotypes differ from SDP-2 and each other; RNAi inactivation of DSpd-2 in cultured cells showed mild effects on centrosome organization,Citation44 but centriole duplication was not affected in somatic cells of DSpd-2 mutants.Citation45 However, pericentriolar components fail to accumulate normally at the sperm centriole following fertilization of DSpd-2 deficient eggs.Citation45 RNAi inactivation of Cep192 did not result in loss of centrioles tagged with centrin:GFP, but both spindle and centrosome organization was severely disrupted.Citation46 These findings highlight the difficulty in establishing whether centrosomal proteins are true orthologs or proteins with similar, but distinct functions. The challenge is acerbated by use of RNAi methods and transposon insertion mutants that produce incomplete inactivation. Additional proteins have been implicated in centriole assembly, such as Cep135 in vertebratesCitation40 and asterless in DrosophilaCitation47 that may not have orthologs in Caenorhabditis or humans.
Mortality of centrioles and basal bodies.
A widely held perception is that centrioles are effectively immortal; once assembled, centrioles are inherited in successive cell cycles as preformed protein structures. This perception is well founded in that centrioles are known to be highly stable to conditions that disassemble other microtubule arrays,Citation48,Citation49 but direct analysis of centriole longevity is limited. Two lines of evidence suggest that centriole structure in Caenorhabditis is dynamic, at least to a limited extent. One set of studies first showed that reduced expression of SAS-4, SAS-5 or SAS-6 generated shortened centrioles and reduced the corresponding size of the pericentriolar mass and microtubule aster,Citation41 as if the aster of microtubules formed by centrosomes reflects centriole length and presumably stability. This is consistent with reduced levels of SAS-4:GFP in preassembled centrioles by reduced expression of SAS-5 or SAS-6 by RNAi, suggesting centriole assembly is reversible. The second set of studies used marked mating experiments in which sperm of wild-type males fertilized eggs expressing SAS-6:GFP. The results of this experiment showed that SAS-6 becomes associated with both newly formed centrioles as well as the preassembled parental centrioles. Taken together, these studies suggest that centrioles can disassemble, albeit perhaps more likely in centrioles with singlet microtubules or lacking δ-, ε-, η- and/or ζ-tubulins. In this context it is noteworthy that the absence of basal bodies in sensory cilia in CaenorhabditisCitation50 could reflect disassembly of a preexisting basal body. Further, monastral bipolar spindles in which only one pole shows evidence of a centrosomeCitation44,Citation51,Citation52 could reflect loss of centrosome organization that is secondary to transient disassembly of centrioles.
Centriole Inheritance by Mitosis
Centrioles and cell cycle progression.
Centrioles assemble near the proximal end of a preexisting centriole that was built in an earlier cell cycle and inherited by mitosis and cell division. The concept of a preformed microtubule template or a centriole-localized genome that directs centriole assembly has been discarded for lack of experimental support.Citation53,Citation54 Yet, the nearly universal pattern of assembly suggests that the parental centriole contributes in some way to assembly of the new centriole. One of the first studies to address this question in cultured cells used microsurgical techniques to generate cytoplasts that contained centrioles, but lacked a nucleus and karyoplasts that contained a nucleus, but lacked centrioles (). The karyoplast remained viable, but new centrioles did not form,Citation55 suggesting a preexisting centriole might be needed for new centriole assembly. Subsequent studies followed cell fragments with continuous microscopic observation,Citation56,Citation57 producing three important findings. First, acentriolar karyoplasts entered and completed mitosis, supporting in vitro studies showing centrosomes are not essential for assembly of bipolar spindles.Citation58 Second, acentriolar cells successfully completed cytokinesis less often than cells with centrioles, suggesting a role for centrioles in the fidelity of cytokinesis. Third, acentriolar cells generated by cytokinesis arrested prior to entry into S phase, as if cells cannot exit G1 without a centriole in much the same way that cells cannot exit mitosis without a bipolar spindle. A centriole checkpoint offered an attractive method to block proliferation of acentriolar cells,Citation59 but an outstanding question was whether removal of centrioles elicited a stress driven cell cycle arrest in response to loss of cell cycle regulators associated with centrosomeCitation60,Citation61 or simply to stress induced by the mechanics of centrosome removal.
De novo assembly of centrioles in acentriolar cultured cells.
The existence of a centriole checkpoint was examined in transformed Hela cellsCitation62 that show suppressed p53 and Rb function and compromised surveillance of the G1/S checkpoint as a result. Centrioles tagged with centrin:GFP at the poles of metaphase spindles were ablated with a laser and acentriolar cells were generated by cytokinesis as in previous experiments. Acentriolar Hela cells not only progressed through the cell cycle at the same pace as sibling control cells with centrosomes, but also formed centrioles de novo (). Surprisingly, subsequent studies in normal non-transformed cells also showed de novo assembly of centrioles.Citation63 Cell cycle arrest in acentriolar cells generated in earlier worksCitation55–Citation57 was shown to be a stress response elicited by removal of centrioles by laser ablation and microsurgery that was not evident until the G1/S transition in the following cell cycle. An implication of these findings is that a preformed template is not needed to form a new centriole or to progress through the G1/S checkpoint.
Centrioles assembled de novo were comparable to centrioles formed adjacent to a parental centriole in two important ways (). First, de novo assembled centrioles showed normal structure at the ultra-structural level. This is in contrast to centrioles assembled de novo in cells delayed in S phase, which included a mixture of normal and malformed centrioles.Citation64,Citation65 Malformed centrioles assembled de novo in S phase arrested cells may, directly or indirectly, reflect delay in S phase or the methods used to elicit S phase delay. Second, de novo assembled centrioles behaved like those in non-transformed cells (); pro/centrioles showed random excursions throughout the cell during the first cell cycle, showing minimal pericentriolar material and virtually no microtubules that could impede movement. Similar excursions of the newly formed centrioles are evident in some cell types where the parental and newly formed centrioles disjoin rather than remaining associated as a centriole pair.Citation66 In both cases, wandering centrioles eventually coalesced and formed stationary groups that were surrounded by a mass pericentriolar material and a radial array of microtubules.
Centriole induced suppression of de novo assembly of new centrioles.
Following de novo assembly of centrioles during the first S-phase in acentriolar cells (, S1), new centrioles did not appear in the cytoplasm during the subsequent S-phase (, S2) and then new centrioles only appeared adjacent to preassembled parental centrioles,Citation63 as if the presence of a centriole somehow suppressed the de novo assembly pathway. Subsequent studies have addressed this question in Drosophila, but the results raise new questions. Inactivation of Sak kinase, like Plk-4 in humans, reduced centriole number,Citation67 consistent with a role in centriole assembly. Sak was overexpressed in an attempt to drive centriole assembly, using ectopic promoters to drive expression of GFP tagged proteins.Citation68 Overexpression of Sak in larval brains generated extra foci of pericentriolar components as assayed by immunofluorescence analysis. However, these foci did not generate multipolar spindles as typical of cells with extra centrosomes. The presence of centrioles or centriole precursors was not tested by ultra-structural analysis and the possibility that the foci represent aggregates of pericentriolar material without assembly of centrioles cannot be ruled out. These results alone are consistent with the view that de novo assembly of new centrioles is suppressed in cells containing centrioles.
Different results were obtained in acentriolar Drosophila eggs,Citation68,Citation69 where centrioles are inactivated and/or discarded during oogenesis.Citation70 Although sufficient materials to assemble thousands of centrioles are provided to the eggs during oogenesis, centrioles do not form de novo. Rather, sperm at fertilization provides a founder centriole and centrioles duplicate once each cycle as in other cell types. High levels of Sak/Plk-4 as well as DSas-6 and DSas-4 over expression generated many masses in unfertilized eggs that were immunostained with antibodies against centrosomal proteins and many were associated with microtubules, consistent with de novo centriole assembly and centrosome organization. However, these foci did not appear to duplicate and increase in number during continuous live imaging.Citation68 The relationship between these structures and similar microtubule asters that transiently form during oocyte activationCitation17 is not known. Ultra-structural analysis was not performed on masses induced by overexpression of Sak or DSas-4, but transmission electron microscopy of masses generated by DSas-6 overexpression showed aberrant tube-like structures, but not recognizable centriole barrels.Citation69 Taken together, these studies indicate that overexpression of Sak, DSas-4 and DSas-6 does not result in de novo assembly of normal centrioles, but may induce organization of centriole precursors and pericentriolar masses capable of organizing microtubules. Further advance may be afforded by development of an in vitro system for centriole assembly that allows controlled addition of one or multiple components.
Proliferation of acentriolar cells and the spindle assembly checkpoint.
Mutations in the DSas-4 gene block centriole duplication in Drosophila; ultra-structural analysis indicated the absence of identifiable centrioles in somatic cells of DSas-4 mutants.Citation71 Spindle assembly was slowed, but mitosis continued without significant cell cycle delay. Development was remarkably normal, save for aberrant cilia and flagella that require a centriole/basal body for assembly. DSas-4 mutant animals are uncoordinated due to defects in sensory neurons and die shortly after birth. A plausible explanation offered for cell proliferation in DSas-4 mutants is that wild-type protein contributed by heterozygous females to unfertilized eggs sustains embryogenesis, but is gradually lost in sequential mitotic divisions during larval development. Gradual dilution of DSas-4 may allow mutant cells to gradually adjust to changes in centriole length and/or relocation of pericentriolar material. Similar explanations may apply to centrosominCitation72 and asterless,Citation47,Citation73 mutants in Drosophila. These results seem to confirm studies in vitro indicating that neither centrioles nor centrosomes are essential for spindle assembly, mitosis or cell cycle progression.Citation58
The availability of acentriolar DSas-4 mutants allows tests of the role of centrioles and centrosomes in Drosophila development. The position of centrosomes in cells determines spindle positioning and the plane of cell division. Stem cells frequently utilize asymmetric positioning of spindles to allow self-renewal of one daughter as a stem cell and one daughter to differentiate. Partitioning of centrosomes during division of Drosophila germline stem cells was proposed to help keep one daughter as a stem cell and move the other toward differentiation.Citation70,Citation74 The role of centriole duplication and centrosomes in oogenesis and embryogenesis in Drosophila was examined in pupal ovaries of homozygous DSas-4 mutant females as well as in germline clones.Citation75 The lack of centrioles and organized centrosomes had no effect on asymmetric division of germline stem cells or larval neuralblasts.Citation75 These and other results in this work indicated that asymmetric inheritance of the parental centriole or asymmetric behavior of centrosomes is not essential features of stem cell divisions in Drosophila as previously suggested (refs. Citation76 and Citation77).
Perspectives
As genetic, molecular and cell biological studies continue to provide new insights into centriole inheritance, new questions emerge. If centrosomes are not essential for microtubule nucleation or spindle assembly, why are centrosomes and centrioles present in virtually all animal cells? If spindles can form without any centrioles or centrosomes, why does the presence of one centrosome generate monopolar spindles? Although canonical centrioles are not essential, do apparently acentriolar cells have unrecognizable centriole substructures that provide the function of centrioles without the structure? Does the involvement of pericentriolar proteins in centriole assembly indicate that centrioles reflect self-assembly of pericentriolar matrix? If so, what underlies recruitment and/or self-assembly of pericentriolar components? Further work may show whether function in assembly of cilia and flagella offers sufficient explanation for conservation or whether centrioles in animal cells reflect structures and functions that are not yet appreciated.
Figures and Tables
Figure 1 Centrosome structure. Pericentriolar material surrounds a pair of barrels-shaped centrioles. Minus ends of astral microtubules lie in pericentriolar matrix and plus ends extend outward. The designated plus-end of microtubules is more dynamic, frequently growing or shrinking. The opposing minus end is also dynamic although tubulin is lost, but not added in vivo.
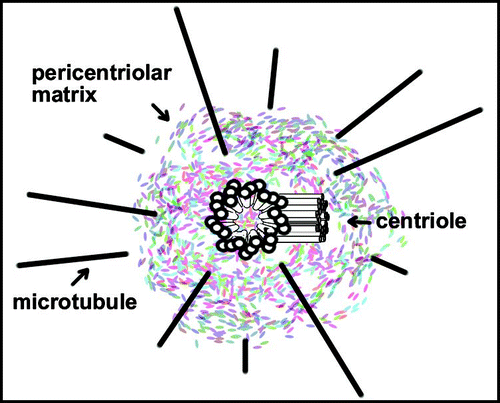
Figure 2 Spindle fusions in γ-tubulin mutants. Spindles in embryos from wild-type (wt) and γ-Tub37C (γ-mat) mutants as indicated. Embryos were stained with antibodies against α-tubulin to visualize microtubules and a fluorescent chromatin dye to visualize chromosomes. Arrows indicate some sites of apparent spindle fusion. Magnification identical in all panels.
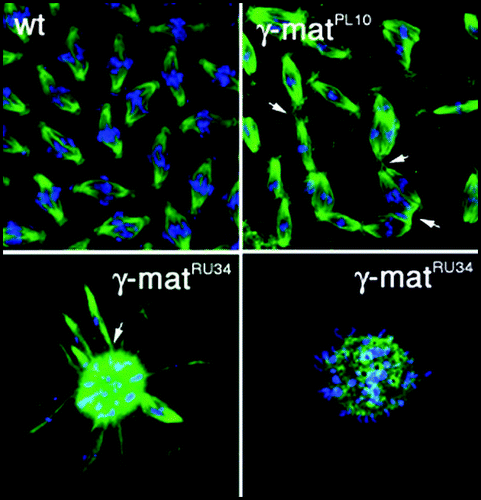
Figure 3 Centriole and basal body structure. Amorphous material is the first indication of a forming centriole or basal body. (A–C) The A-, B- and C-tubules appear sequentially. (D) Appendages form at the distal end of the parental centriole. (E–G) The transition zone marks the end of C-tubules in basal bodies. B- and C-tubules extend into axonemes. (H) The central pair of microtubules emerges in the transition zone, but they are not present in cilia. (E–H) The plasma membrane surrounding basal body/axonemes is depicted as a circle. Modified from tomography of centriole/basal body structure in Chlamydomonas.Citation33
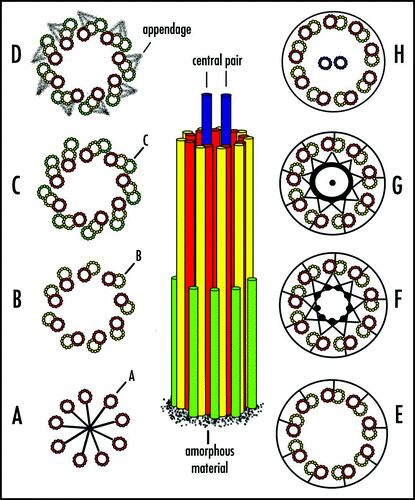
Figure 4 The centriole cycle and the spindle assembly checkpoint. (A) Normal centriole cycle. (B1) Failure in centriole duplication, but centrosomes with a single centriole assemble a bipolar spindle. (B2) A single centrosome is partitioned to daughter cells that generate a monopolar spindle. Centriole pairs are connected by fibrillar material, designated by black dots. Parental centriole shows distal appendages (blue lines) that may be vestiges of centriole assembly since they are not essential for mitosis or viability.Citation37 Pericentriolar matrix and microtubule asters are not depicted for simplicity.
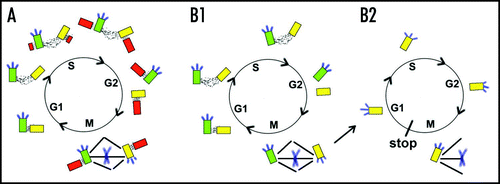
Figure 5 Centriole checkpoint at the G1/S transition. Acentriolar cells were generated by microsurgery with the sharpened tip of glass pipetteCitation56 as shown here or by laser ablation.Citation57 Karyoplasts complete mitosis and often cytokinesis, arresting before passing the G1/S phase transition. Mitosis in control cells is depicted at the top. For simplicity, asters of microtubules in interphase cells are not shown.
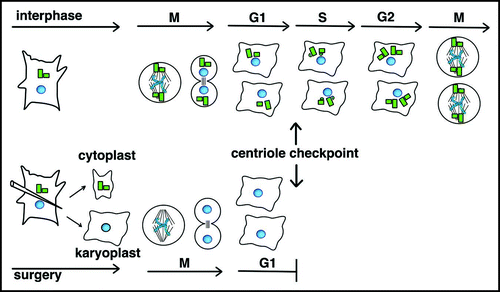
Figure 6 De novo assembly of centrioles. Following laser ablation of centrioles and completion of mitosis (M0), centrin-GFP in presumptive procentrioles (small dots) form in the first S-phase (S1). Pro/centrioles coalesce in G12 when centrioles acquire a robust pericentriolar mass and an aster of microtubules. Note that immature de novo assembled centrioles are depicted at the poles of a bipolar spindle at M1 for simplicity, but multipolar spindles were also formed. Because immature centrioles are not associated with microtubules, positioning of immature de novo assembled centrioles at spindle poles in M1 may reflect a microtubule-independent mechanism. Control cells retaining centrioles undergo normal cell cycle progression, but only G11-M1 is depicted here.
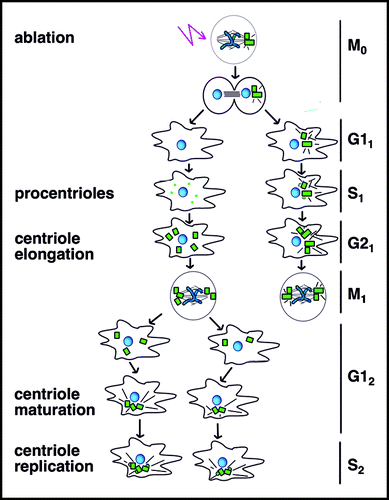
Table 1 γ-tubulin containing complexesTable Footnotea
Table 2 The extended tubulin superfamilyTable Footnotea
Acknowledgements
I am grateful to the editor and reviewers for critical reading of the manuscript and helpful suggestions. I want to thank S. Nickerson and the Department of Animal and Dairy Science at the University of Georgia for defraying charges for color figures.
Note
This manuscript has been previously published: Centriole Wilson P. Chernoff Y. Inheritance. Protein-Based Inheritance 2007; Austin and New York Landes Bioscience and Kluwer Academic Press 119 - 133
References
- Moritz KB, Sauer HW. Boveri's contributions to developmental biology-a challenge for today. Int J Dev Biol 1996; 40:27 - 47
- Sathananthan AH, Ratnasooriya WD, de Silva A, Randeniya P. Rediscovering Boveri's centrosome in Ascaris (1888): Its impact on human fertility and development. Reprod Biomed Online 2006; 12:254 - 270
- Gadde S, Heald R. Mechanisms and molecules of the mitotic spindle. Curr Biol 2004; 14:797 - 805
- Hannak E, Heald R. Xorbit/CLASP links dynamic microtubules to chromosomes in the Xenopus meiotic spindle. J Cell Biol 2006; 172:19 - 25
- Maiato H, Khodjakov A, Rieder CL. Drosophila CLASP is required for the incorporation of microtubule subunits into fluxing kinetochore fibres. Nat Cell Biol 2005; 7:42 - 47
- Zariwala MA, Knowles MR, Omran H. Genetic defects in ciliary structure and function. Annu Rev Physiol 2007; 69:423 - 450
- Desai A, Mitchison TJ. Microtubule polymerization dynamics. Annu Rev Cell Dev Biol 1997; 13:83 - 117
- Nogales E, Wang HW. Structural mechanisms underlying nucleotide-dependent self-assembly of tubulin and its relatives. Curr Opin Struct Biol 2006; 16:221 - 229
- Nogales E, Wang HW. Structural intermediates in microtubule assembly and disassembly: How and why?. Curr Opin Cell Biol 2006; 18:179 - 184
- Wiese C, Zheng Y. Microtubule nucleation: {gamma}-tubulin and beyond. J Cell Sci 2006; 119:4143 - 4153
- Moritz M, Agard DA. Gamma-tubulin complexes and microtubule nucleation. Curr Opin Struct Biol 2001; 11:174 - 181
- Janson ME, Setty TG, Paoletti A, Tran PT. Efficient formation of bipolar microtubule bundles requires microtubule-bound gamma-tubulin complexes. J Cell Biol 2005; 169:297 - 308
- Shimamura M, Brown RC, Lemmon BE, Akashi T, Mizuno K, Nishihara N, Tomizawa K, Yoshimoto K, Deguchi H, Hosoya H, Horio T, Mineyuki Y. Gamma-tubulin in basal land plants: Characterization, localization, and implication in the evolution of acentriolar microtubule organizing centers. Plant Cell 2004; 16:45 - 59
- Hashimoto T, Kato T. Cortical control of plant microtubules. Curr Opin Plant Biol 2006; 9:5 - 11
- Wilson PG, Zheng Y, Oakley CE, Oakley BR, Borisy GG, Fuller MT. Differential expression of two gamma-tubulin isoforms during gametogenesis and development in Drosophila. Dev Biol 1997; 184:207 - 221
- Martin MA, Osmani SA, Oakley BR. The role of gamma-tubulin in mitotic spindle formation and cell cycle progression in Aspergillus nidulans. J Cell Sci 1997; 110:623 - 633
- Wilson PG, Borisy GG. Maternally expressed gamma Tub37CD in Drosophila is differentially required for female meiosis and embryonic mitosis. Dev Biol 1998; 199:273 - 290
- Strome S, Powers J, Dunn M, Reese K, Malone CJ, White J, Seydoux G, Saxton W. Spindle dynamics and the role of gamma-tubulin in early Caenorhabditis elegans embryos. Mol Biol Cell 2001; 12:1751 - 1764
- Hannak E, Oegema K, Kirkham M, Gonczy P, Habermann B, Hyman AA. The kinetically dominant assembly pathway for centrosomal asters in Caenorhabditis elegans is gamma-tubulin dependent. J Cell Biol 2002; 157:591 - 602
- Muller H, Fogeron ML, Lehmann V, Lehrach H, Lange BM. A centrosome-independent role for gamma-TuRC proteins in the spindle assembly checkpoint. Science 2006; 314:654 - 657
- Verollet C, Colombie N, Daubon T, Bourbon HM, Wright M, Raynaud-Messina B. Drosophila melanogaster gamma-TuRC is dispensable for targeting gamma-tubulin to the centrosome and microtubule nucleation. J Cell Biol 2006; 172:517 - 528
- Kerssemakers JW, Laura Munteanu E, Laan L, Noetzel TL, Janson ME, Dogterom M. Assembly dynamics of microtubules at molecular resolution. Nature 2006; 442:709 - 712
- Brittle AL, Ohkura H. Mini spindles the XMAP215 homologue, suppresses pausing of interphase microtubules in Drosophila. Embo J 2005; 24:1387 - 1396
- Al-Bassam J, van Breugel M, Harrison SC, Hyman A. Stu2p binds tubulin and undergoes an open-to-closed conformational change. J Cell Biol 2006; 172:1009 - 1022
- Srayko M, Kaya A, Stamford J, Hyman AA. Identification and characterization of factors required for microtubule growth and nucleation in the early C. elegans embryo. Dev Cell 2005; 9:223 - 236
- Popov AV, Severin F, Karsenti E. XMAP215 is required for the microtubule-nucleating activity of centrosomes. Curr Biol 2002; 12:1326 - 1330
- Calarco-Gillam PD, Siebert MC, Hubble R, Mitchison T, Kirschner M. Centrosome development in early mouse embryos as defined by an autoantibody against pericentriolar material. Cell 1983; 35:621 - 629
- Fuller SD, Gowen BE, Reinsch S, Sawyer A, Buendia B, Wepf R, Karsenti E. The core of the mammalian centriole contains gamma-tubulin. Curr Biol 1995; 5:1384 - 1393
- Dutcher SK. Long-lost relatives reappear: Identification of new members of the tubulin superfamily. Curr Opin Microbiol 2003; 6:634 - 640
- Hamill DR, Severson AF, Carter JC, Bowerman B. Centrosome maturation and mitotic spindle assembly in C. elegans require SPD-5, a protein with multiple coiled-coil domains. Dev Cell 2002; 3:673 - 684
- Dammermann A, Muller-Reichert T, Pelletier L, Habermann B, Desai A, Oegema K. Centriole assembly requires both centriolar and pericentriolar material proteins. Dev Cell 2004; 7:815 - 829
- Stumpff J, Kellogg DR, Krohne KA, Su TT. Drosophila Wee1 interacts with members of the gammaTURC and is required for proper mitotic-spindle morphogenesis and positioning. Curr Biol 2005; 15:1525 - 1534
- O'Toole ET, Giddings TH, McIntosh JR, Dutcher SK. Three-dimensional organization of basal bodies from wild-type and delta-tubulin deletion strains of Chlamydomonas reinhardtii. Mol Biol Cell 2003; 14:2999 - 3012
- Chang P, Giddings TH Jr, Winey M, Stearns T. Epsilon-tubulin is required for centriole duplication and microtubule organization. Nat Cell Biol 2003; 5:71 - 76
- Quarmby LM, Mahjoub MR. Caught Nek-ing: Cilia and centrioles. J Cell Sci 2005; 118:5161 - 5169
- Pelletier L, O'Toole E, Schwager A, Hyman AA, Muller-Reichert T. Centriole assembly in Caenorhabditis elegans. Nature 2006; 444:619 - 623
- Ishikawa H, Kubo A, Tsukita S, Tsukita S. Odf2-deficient mother centrioles lack distal/subdistal appendages and the ability to generate primary cilia. Nat Cell Biol 2005; 7:517 - 524
- Swallow CJ, Ko MA, Siddiqui NU, Hudson JW, Dennis JW. Sak/Plk4 and mitotic fidelity. Oncogene 2005; 24:306 - 312
- Habedanck R, Stierhof YD, Wilkinson CJ, Nigg EA. The Polo kinase Plk4 functions in centriole duplication. Nat Cell Biol 2005; 7:1140 - 1146
- Kleylein-Sohn J, Westendorf J, Le Clech M, Habedanck R, Stierhof YD, Nigg EA. Plk4-induced centriole biogenesis in human cells. Dev Cell 2007; 13:190 - 202
- Leidel S, Gonczy P. Centrosome duplication and nematodes: Recent insights from an old relationship. Dev Cell 2005; 9:317 - 325
- Delattre M, Canard C, Gonczy P. Sequential protein recruitment in C. elegans centriole formation. Curr Biol 2006; 16:1844 - 1849
- Strnad P, Leidel S, Vinogradova T, Euteneuer U, Khodjakov A, Gonczy P. Regulated HsSAS-6 levels ensure formation of a single procentriole per centriole during the centrosome duplication cycle. Dev Cell 2007; 13:203 - 213
- Goshima G, Wollman R, Goodwin SS, Zhang N, Scholey JM, Vale RD, Stuurman N. Genes required for mitotic spindle assembly in Drosophila S2 cells. Science 2007; 316:417 - 421
- Dix CI, Raff JW. Drosophila Spd-2 recruits PCM to the sperm centriole, but is dispensable for centriole duplication. Curr Biol 2007; 17:1759 - 1764
- Gomez-Ferreria MA, Rath U, Buster DW, Chanda SK, Caldwell JS, Rines DR, Sharp DJ. Human Cep192 is required for mitotic centrosome and spindle assembly. Curr Biol 2007;
- Varmark H, Llamazares S, Rebollo E, Lange B, Reina J, Schwarz H, Gonzalez C. Asterless is a centriolar protein required for centrosome function and embryo development in Drosophila. Curr Biol 2007; 17:1735 - 1745
- Bobinnec Y, Khodjakov A, Mir LM, Rieder CL, Edde B, Bornens M. Centriole disassembly in vivo and its effect on centrosome structure and function in vertebrate cells. J Cell Biol 1998; 143:1575 - 1589
- Rosenbaum J. Cytoskeleton: Functions for tubulin modifications at last. Curr Biol 2000; 10:801 - 803
- Wright KA. Peripheral sensilla of some lower invertebrates: The platyhelminthes and nematoda. Microsc Res Tech 1992; 22:285 - 297
- Wilson PG, Fuller MT, Borisy GG. Monastral bipolar spindles: Implications for dynamic centrosome organization. J Cell Sci 1997; 110:451 - 464
- Kwok BH, Yang JG, Kapoor TM. The rate of bipolar spindle assembly depends on the microtubule-gliding velocity of the mitotic kinesin Eg5. Curr Biol 2004; 14:1783 - 1788
- Fulton C. Origin and Continuity of Cell Organelles 1971; New York Springer-Verlag, New York: Inc.
- Marshall WF, Rosenbaum JL. Are there nucleic acids in the centrosome?. Curr Top Dev Biol 2000; 49:187 - 205
- Maniotis A, Schliwa M. Microsurgical removal of centrosomes blocks cell reproduction and centriole generation in BSC-1 cells. Cell 1991; 67:495 - 504
- Hinchcliffe EH, Miller FJ, Cham M, Khodjakov A, Sluder G. Requirement of a centrosomal activity for cell cycle progression through G1 into S phase. Science 2001; 291:1547 - 1550
- Khodjakov A, Rieder CL. Centrosomes enhance the fidelity of cytokinesis in vertebrates and are required for cell cycle progression. J Cell Biol 2001; 153:237 - 242
- Wadsworth P, Khodjakov A. E pluribus unum: Towards a universal mechanism for spindle assembly. Trends Cell Biol 2004; 14:413 - 419
- Murray AW. Cell cycle: Centrioles at the checkpoint. Science 2001; 291:1499 - 1502
- Srsen V, Merdes A. The centrosome and cell proliferation. Cell Div 2006; 1:26
- Loffler H, Lukas J, Bartek J, Kramer A. Structure meets function-centrosomes, genome maintenance and the DNA damage response. Exp Cell Res 2006; 312:2633 - 2640
- La Terra S, English CN, Hergert P, McEwen BF, Sluder G, Khodjakov A. The de novo centriole assembly pathway in HeLa cells: Cell cycle progression and centriole assembly/maturation. J Cell Biol 2005; 168:713 - 722
- Uetake Y, Loncarek J, Nordberg JJ, English CN, La Terra S, Khodjakov A, Sluder G. Cell cycle progression and de novo centriole assembly after centrosomal removal in untransformed human cells. J Cell Biol 2007; 176:173 - 182
- Marshall WF, Vucica Y, Rosenbaum JL. Kinetics and regulation of de novo centriole assembly: Implications for the mechanism of centriole duplication. Curr Biol 2001; 11:308 - 317
- Khodjakov A, Rieder CL, Sluder G, Cassels G, Sibon O, Wang CL. De novo formation of centrosomes in vertebrate cells arrested during S phase. J Cell Biol 2002; 1171 - 1181
- Piehl M, Tulu US, Wadsworth P, Cassimeris L. Centrosome maturation. Measurement of microtubule nucleation throughout the cell cycle by using GFP-tagged EB1. Proc Natl Acad Sci USA 2004; 101:1584 - 1588
- Bettencourt-Dias M, Rodrigues-Martins A, Carpenter L, Riparbelli M, Lehmann L, Gatt MK, Carmo N, Balloux F, Callaini G, Glover DM. SAK/PLK4 is required for centriole duplication and flagella development. Curr Biol 2005; 15:2199 - 2207
- Peel N, Stevens NR, Basto R, Raff JW. Overexpressing centriole-replication proteins in vivo induces centriole overduplication and de novo formation. Curr Biol 2007; 17:834 - 843
- Rodrigues-Martins A, Bettencourt-Dias M, Riparbelli M, Ferreira C, Ferreira I, Callaini G, Glover DM. DSAS-6 organizes a tube-like centriole precursor, and its absence suggests modularity in centriole assembly. Curr Biol 2007; 17:1465 - 1472
- Fuller MT, Spradling AC. Male and female Drosophila germline stem cells: Two versions of immortality. Science 2007; 316:402 - 404
- Basto R, Lau J, Vinogradova T, Gardiol A, Woods CG, Khodjakov A, Raff JW. Flies without centrioles. Cell 2006; 125:1375 - 1386
- Megraw TL, Kao LR, Kaufman TC. Zygotic development without functional mitotic centrosomes. Curr Biol 2001; 11:116 - 120
- Giansanti MG, Gatti M, Bonaccorsi S. The role of centrosomes and astral microtubules during asymmetric division of Drosophila neuroblasts. Development 2001; 128:1137 - 1145
- Spradling AC, Zheng Y. Developmental biology: The mother of all stem cells?. Science 2007; 315:469 - 470
- Stevens NR, Raposo AA, Basto R, St Johnston D, Raff JW. From stem cell to embryo without centrioles. Curr Biol 2007; 17:1498 - 1503
- Yamashita YM, Jones DL, Fuller MT. Orientation of asymmetric stem cell division by the APC tumor suppressor and centrosome. Science 2003; 301:1547 - 1550
- Yamashita YM, Mahowald AP, Perlin JR, Fuller MT. Asymmetric inheritance of mother versus daughter centrosome in stem cell division. Science 2007; 315:518 - 521