Abstract
A dysfunction in copper homeostasis seems to occur in Alzheimer’s disease (AD). We recently demonstrated that an excess of non-ceruloplasmin-copper (i.e. ‘free’ copper) correlates with the main functional and anatomical deficits as well as the cerebrospinal markers of the disease, thus suggesting that copper contributes to AD neurodegeneration. Aim of this study was to investigate the profile of serum ceruloplasmin isoforms immunoreactive protein in relation to copper dysfunction in AD. Twenty-five AD patients and 25 controls were included in the study. All subjects underwent individual measurements of serum ceruloplasmin and copper concentrations, and the amount of ‘free’ copper was computed for each copper and ceruloplasmin pair. Serum samples were also pooled and analyzed by two dimensional polyacrylamide gel electrophoresis (2-D PAGE) and western blot analysis. The mean concentration of ’free’ copper resulted higher in AD patients than in controls. Ceruloplasmin 2-D PAGE western blot analysis of pooled sera showed in the AD samples low-molecular-weight spots in the <50 kDa range that were not detected in controls’ pooled sera (p < 0.029). Our data indicate a ceruloplasmin fragmentation in the serum of AD patients, possibly related to ‘free’ copper deregulation in this disease.
Introduction
Alzheimer's Disease (AD) is a heterogeneous and progressive neurodegenerative disorder representing the most common cause of dementia in the elderly. Recently, the ability of certain proteins involved in AD neurodegeneration—as well as in other neurodegenerative diseases such as the Prion diseases—to reversibly bind some metals with high capacity and sufficient stability constant to affect the metal's homeostasis has become the focus of increasing research. It reasonable to think that an abnormal homeostasis of these metals in the brain—in particular copper in both AD and Prion diseases—may contribute to set up chemical conditions in which toxicity is promoted. For example, there is compelling evidence in AD of anomalous interactions of the protein beta amyloid (Aβ) with copper, causing oxidative stress. High concentrations of copper are found within senile plaques and neurofibrillary tangles of AD brainsCitation1,Citation2 of which Aβ is the main constituent, and the amyloid precursor protein (APP) is known to be a crucial regulator of neuronal copper homeostasis.Citation3 These phenomena could be due simply to aging or to variations in levels of circulating blood copper.Citation4
Copper is a cofactor of several intracellular enzymes, including cytochrome oxidase, copper-zinc superoxide dismutase and lysyl oxidase. About 95% of copper contained in human serum travels bound to ceruloplasmin, making this protein a central actor of copper transport and metabolism. The remaining amount—generally referred to as ‘free’ copper—binds to albumin, amino acids (e.g., histidine), and low-molecular-weight complexes (0.5–5% normal value < 1.6 µmol/L),Citation5,Citation6 which can easily cross the brain blood barrier (BBB).Citation6
The distribution of copper in AD is actually very complex. There is new evidence suggesting that brain copper may redistribute outside the neuronal cell, leaving it relatively deficient.Citation7 Although past studies in man have found no differences between AD and controls in serum copper levels,Citation8–Citation11 more sophisticated measurements have recently shown both an increase,Citation12–Citation15 and a decrease of these levels,Citation16,Citation17 thus opening a debate on a toxic or protective role of copper in AD. Results from our laboratory, though, suggest that it is the ceruloplasmin-copper relationship, rather than the level of absolute (i.e., bound and not bound to ceruloplasmin) serum copper, that may be the key issue in interpreting in vivo copper findings in AD.Citation18 This notion is not in contrast with the previously cited AD studies. In fact, they considered absolute serum copper levels,Citation8–Citation11,Citation16–Citation17 and their controversy does not dispute the ceruloplasmin-copper relationship and the evidence of ‘free’ copper, whose levels can be easily computed from the absolute and bound forms of copper on the basis of the fact that ceruloplasmin contains 0.3% of copper.Citation5 In fact, even those authors reporting decreased levels in AD of absolute serum copper confirm a copper/ceruloplasmin dyshomeostasis.Citation10,Citation11,Citation17,Citation18 Snaedal et al., (1998)Citation11 found similar levels of absolute serum copper in AD and controls, but significantly lower concentrations of ceruloplasmin, which corresponds to a higher concentration of ‘free’ copper in AD than in controls. Kessler and colleagues (2006)Citation17 reported data coherent with a 2.5 fold increase of ‘free’ copper in AD with respect to normal values (<1.6 µmol/L).Citation5 Sedighi et al. (2006)Citation10 reported a 40% increase of ‘free’ copper in AD compared to controls, even though they found similar levels of copper and ceruloplasmin. Our observations were also recently confirmed by two other groups (Althaus, personal communication).Citation19 In particular, hard data from Althaus' study indicated that ‘free’ copper levels in AD sera nearly doubled the ones in normal sera (AD 2.27 +/− 0.16 µmol/L; normal 1.56 +/− 0.29 µmol/L). Moreover, one must remember that ‘free’ copper represents the most important diagnostic marker for Wilson's disease—the paradigmatic disease of copper toxicosis and accumulation.Citation5,Citation6 In particular, our recent biochemical studies demonstrated that a discrete concentration increase of copper occurs in AD which exceeds the amount of ceruloplasmin,Citation15 and that a considerable amount of inactive ceruloplasmin can be detected in the cerebrospinal fluid of AD patients.Citation20
Since ceruloplasmin is the main copper transporter in general circulation, we aimed in the present work at studying the qualitative changes occurring in the ceruloplasmin present in the serum of AD patients, explored by means of a two dimensional polyacrylamide gel electrophoresis (2-D PAGE) approach, to detect possible protein modifications that can be considered explanatory of ‘free’ copper deregulation.
Results
‘Free’ copper concentration is higher in the serum of AD patients than controls.
shows the levels of serum copper biological variables expressed as mean and standard deviation (SD) of individual concentrations contributing to patient and control pools. Serum absolute (i.e., total of bound and not bound to ceruloplasmin) copper was higher in the AD group (p < 0.001), while differences between AD and controls in concentrations of ceruloplasmin and bound copper were not evident (p = 0.369). The computed concentration of ‘free’ copper resulted instead higher in AD patients than in controls (t test, p = 0.001).
Ceruloplasmin 2D map in serum of AD patients and controls.
To reveal qualitative isoform differences in serum ceruloplasmin between AD and controls, we carried out 2-D PAGE experiments by pooling sera weighted for the same amount of ceruloplasmin (28 mg/dL) per sample. No gross changes were detected for the 135 and 115 kDa molecular weight forms ( and ). The immunoblotting 2-D maps show various spots in the pH 4.5–pH 5.7 range, resolving the 135 and the 115 kDa band (), usually detected on ceruloplasmin SDS-PAGE. The main difference between AD patients' and controls ceruloplasmin was the presence of lower molecular weight spots (<50 kDa) in patients' serum, not detected in controls' ( and ). Analysis of the separated 135 kDa and 115 kDa spots, identified on a master map created by using the PDQuest analysis software, showed no qualitative or quantitative differences, suggesting that the spectrum of ceruloplasmin isoforms was substantially superimposable between AD and control samples (). By 2-D PAGE analysis, it was possible to identify eight spots at 135 kDa and five spots at 115 kDa. Quantitative analysis of optical density of the separated spots showed a relative different density >50% in five identified spots, labelled 1 to 5 in . The ceruloplasmin spots 1, 2 and 5 were decreased, while spots 3 and 4 were increased in AD of at least 50%. However, the variability experienced during different trials allowed us to rule out a significant relevance of these differences.
We carried out a densitometric analysis of the spots detected at the three molecular weight bands of ceruloplasmin corresponding to 135 kDa, 115 kDa and <50 kDa forms (). The sum of the percentages of the single “band” optical density at 135 kDa, 115 kDa, 50 kDa represents 100% of the ceruloplasmin immunoreactivity of a sample, which corresponds to 28 mg/dL of ceruloplasmin (see Methods). The analysis allowed calculation of the contribution of each “band” to ceruloplasmin immunoreactivity in the AD and control pools. In particular, the low molecular weight fragments (<50 kDa) in AD appear to represent about 26% of total ceruloplasmin immunoreactivity, while they represent around 1% in controls (Mann Whitney test, p < 0.029, ).
Discussion
The main result of our study is the identification of <50 kDa fragments of ceruloplasmin proteolysis in the serum of AD patients with higher-than-normal ‘free’ copper levels. Ceruloplasmin is routinely measured in clinical chemistry as an immunoreactive protein, comprising both the active holo-form and the inactive and copper-free apo-form of the protein. The spots at high molecular weight (135–115 kDa) of the fully denatured protein that we show in this study may represent a pool of the two forms, as we have recently shown in a dedicated study, revealing a conspicuous amount of apo-ceruloplasmin in the CSF of AD patients.Citation20 The evidence of low-molecular-weight fragments of ceruloplasmin in AD samples suggests that at least a portion of this protein is present in its apoform in general circulation, and indicates that an impairment in the incorporation of copper into the protein may occur in AD during ceruloplasmin biosynthesis. In fact, the six integral copper atoms are incorporated into ceruloplasmin during its biosynthesis through the copper-transporting ATPase ATP7B in the secretory compartment of the liver.Citation21 In this regard, Wilson's disease offers a good example of the effects of the lack or hypofunction of copper incorporation into ceruloplasmin. In this disease, the absence or impairment of ATP7B prevents copper translocation to the secretory pathway, resulting in the secretion of apo-ceruloplasmin which, being unstable, is rapidly degraded in the blood.Citation21,Citation22 Consequently, the idea of an impairment also in AD of copper incorporation into ceruloplasmin simply comes from the notion that the ceruloplasmin apo-protein is rapidly degraded in plasma secondary to a defect in copper incorporation.Citation21–Citation23 In other words, the identification of <50 kDa ceruloplasmin fragments in AD general circulation appears as the sign of an impairment of copper transfer into the secretory pathway of hepatocytes that could, at least in part, account for the rise of the ‘free’ copper portion found in AD. Ceruloplasmin fragmentation, together with the ‘free’ copper rise in AD, could be the cause or the result of a disturbed hepatocytes function, possibly resulting in liver hypometabolism. The latter concept is supported by the results of a previous clinical study of ours which demonstrated that AD patients with no evidence of additional pathological conditions—including liver diseases—had higher ‘free’ copper, longer prothrombin time and lower albumin levels than controls matched for age, sex and risk factors for cardiovascular diseases and medication intake.Citation24 In our opinion, the hypothesis that copper in AD might be related to liver hypofunction linked to APP metabolism at hepatocyte level is strongly supported by the evidence that in the APP knock-out mouse model a massive copper increase (80%) in the liver was found to be caused by APP ablation.Citation25 More precisely, it could be speculated that, in this mouse model, a perturbation of copper efflux linked to APP at the liver level disrupts normal copper transport producing a reduction of the liver's efficiency to excrete copper through the bile, and explaining the elevated (40%) copper level found in the brain.Citation25 A hint towards this interpretation comes from our estimation—via a clearance measure—that about 3% of serum ‘free’ copper crosses the BBB in living patients and possibly interact with Aβ.Citation15 The latter finding was recently confirmed by other authors, who demonstrated an interaction between copper and Aβ in the CSF,Citation26 probably resulting in catalytic generation of peroxides (H2O2) and Aβ aggregation,Citation15,Citation26 ultimately leading to neurodegeneration.Citation27
Alternative hypotheses could be put forward to explain ceruloplasmin fragmentation in AD. For example, it could be caused by oxidative insults as a result of incorrect or excessive copper loading into the protein, or by an upregulation of redox active enzymes causing a general increase of oxidative stress, or a downregulation in enzymes that normally regulate oxidative stress. Both forms of enzyme would be susceptible to changes in copper homeostasis, as it has been reported to occur in AD. Ceruloplasmin fragmentation could also have an extra-hepatic origin, possibly accounting for the inactive apo-form detected in the CSF fluid, which could be collected in general circulation for excretion. However, the latter seems improbable due to the different range of ceruloplasmin concentration in CSF and serum. Further studies are needed to explore these alternative hypotheses.
Ceruloplasmin fragmentation in AD serum is the main change in the ceruloplasmin structure that we detected in this study. In fact, our data show that the ceruloplasmin isoform profile is not qualitatively different in AD patients than in healthy controls in terms of molecular weight and protein isoelectric pH. Actually, the profile of ceruloplasmin isoforms at 115 and 135 kDa in serum reported in the present study is consistent with previous evidence in normal aging,Citation28 and the isoforms of the 135 and 115 kDa bands were qualitatively and quantitatively similar in AD and controls.
Our explorative study has a number of limitations which include: the need for AD selection with possible sampling bias (mild cases may be underrepresented); the exclusion of other dementias and neurodegenerative diseases, not permitting to conclusively rule out similar abnormalities in conditions other than AD; the choice to carry out a qualitative evaluation of the ceruloplasmin isoform profile on a pool of sera rather than performing measurements in individual sera. All these considerations certainly warrant the extension of the investigation to other patient populations and to in vitro models, aiming at elucidating differences in metal binding properties and stability of the ceruloplasmin isoforms.
Materials and Methods
The study was approved by the local IRB and all participants or legal guardians signed an informed consent.
Samples of fresh serum were obtained from 25 individuals (ages 65–81 years, mean = 75 years) with a diagnosis of probable AD according to NINCDS-ADRDA criteriaCitation29 and a Mini-Mental State Examination (MMSE)Citation30 score of 25 or less [(19 (5), mean (SD)] and 25 age- and sex-matched individuals (ages 64–89 years, mean = 76.1 years), cognitively normal and with a mean MMSE score of 28 (1).
Exclusion criteria for both patients and controls were conditions affecting copper metabolism and biological variables of oxidative stress (e.g., diabetes mellitus, inflammatory diseases, Hodgkin's disease, recent history of heart or respiratory failure, chronic liver or renal failure, malignant tumors, alcohol abuse). Serum samples from both AD patients and controls were pooled. Ceruloplasmin content in both pools was made to 28 mg/dL and each serum sample contributed equally to ceruloplasmin content. Serum pools were precipitated in 8:1 v/v 100% methanol. The samples were kept at −20°C for 2 h and then centrifuged at 13,000 rpm for 30 min at 4°C. The supernatant was discarded and the pellet re-suspended in 450 µl of a solution for isoelectric focusing (IEF) (2D PAGE sample buffer), containing 8 M urea, 2% CHAPS, 0.3% DTT, 0.5% Ampholine pH 3.5–10 and traces of bromophenol blue.
Copper and ceruloplasmin content measurements.
Serum copper concentration was measured both following the method of Abe et al., (1989)Citation31 (Randox Laboratories, Crumlin, UK), and utilizing an A Analyst 300 Perkin Elmer atomic absorption spectrophotometer, equipped with a graphite furnace with platform HGA 800. Ceruloplasmin was analyzed by immunoturbidimetry assay using a rabbit anti-human ceruloplasmin antibody in phosphate buffer (Roche, Diagnostic, Germany) automated on a Hitachi 917 analyser (Roche Diagnostics). For each serum copper and ceruloplasmin pair we computed the amount of copper bound to ceruloplasmin (CB) and the amount of copper not bound to ceruloplasmin (‘free’ copper) as follows: CB = n * ceruloplasmin (mg/L); n = 0.0472 (µmol/mg); ‘free’ copper = absolute serum copper - CB [Appendix 1 Calculation of ‘free copper’ concentration].Citation5 This calculation expresses ‘free’ copper in µmol/L and corresponds to that expressed in mg/dL: CB = ceruloplasmin (mg/dL) * 0.03; ‘free’ copper (mg/dL) = absolute serum copper - CB.Citation5 For homogeneity with previous publications we preferred to express ‘free’ copper in µmol/L units.
Electrophoresis and immunoblotting.
2-D PAGE were performed on a Protean IEF Cell (Bio-Rad Laboratories, CA, USA) for about 25 KVh after the dry 17 cm pH 4.5–5.7 and the 7 cm pH 3–10 IPG strips (Bio-Rad Laboratories, CA, USA) were re-hydrated with the sample solution; the strips were then equilibrated and run on a second dimension 10% or 7–20% gradient polyacrylamide gel. Proteins were transferred to nitrocellulose membranes (Bio-Rad Laboratories, CA, USA) at 4°C (60 V, 2 h), blocked in TBS containing 0.2% Tween-20 and 10% non-fat dry milk (1 h at room temperature) and incubated with a 1:10,000 goat anti-human ceruloplasmin antibody solution (1 h at RT) (Sigma-Aldrich Corporation, St. Louis, MI, USA). Films were developed by incubating membranes with 1:80,000 HRP-conjugated anti-goat secondary antibody solution and using an enhanced luminol reagent (Perkin Elmer Life Sciences, Boston, USA). Kodak films were used. Four gel replicates of each pooled sample per experiment were performed and developed on the same film to avoid procedural bias in the optical density reading (intra-run variation assay). Then, three sets of experiments were performed to assess the inter-run variation. Analysis of 2D PAGE films was performed by using PDQuest 2-D analysis software (Bio-Rad Laboratories, CA, USA), creating a master map from match data sets of four replicates and comparing optical density of AD pool vs. control pool.
In preliminary experiments we performed immunoprecipitation of ceruloplasmin from AD serum, using the rabbit antibody from Roche utilized in the immunoturbidimetry assay. In particular, following manufacture procedure of the Roche protein-G agarose kit, we immunoprecipitated ceruloplasmin from 1 ml of the AD serum pool, using 0.5 ml of antibody from Roche, and 1.5 ml of the kit buffer. The immunoprecipitate was then run together with a sample of serum in a SDS-PAGE 10% separating gel, transferred in nitrocellulose, and incubated with 1:10,000 Sigma anti-CP goat antibody solution (1 h at RT). Films were developed by incubating membranes with a 1:80,000 HRP-conjugated anti-goat secondary antibody solution and using an enhanced luminol reagent (Perkin Elmer Life Sciences, Boston, USA). Kodak films were used. The Roche goat anti-ceruloplasmin recognized the low-molecular-weight bands both from the total serum and from the immunoprecipitate (data not shown). Moreover, we used another antibody—from Alpha Diagnostic Intl., Inc.,—developed in rabbit, and the pattern of the ceruloplasmin band was the same of the goat anti-ceruloplasmin. We performed the study with the Sigma antibody since it was less expensive.
Abbreviations
AD | = | Alzheimer's disease |
APP | = | amyloid precursor protein |
MMSE | = | mini-mental state examination |
BBB | = | brain blood barrier |
IEF | = | isoelectric focusing |
SDS-PAGE | = | SDS-polyacrylamide separating gels |
2-D PAGE | = | two dimensional polyacrylamide gel electrophoresis |
CSF | = | cerebrospinal fluid |
Figures and Tables
Figure 1 2D quantitative analysis of serum ceruloplasmin isoforms at 135 and 115 kDa. A master map was created utilizing PDQuest software from a triplicate of AD sample and a quadruplicate of control sample. The optical density analysis of the spots did not show statistically significant differences between AD and control samples.
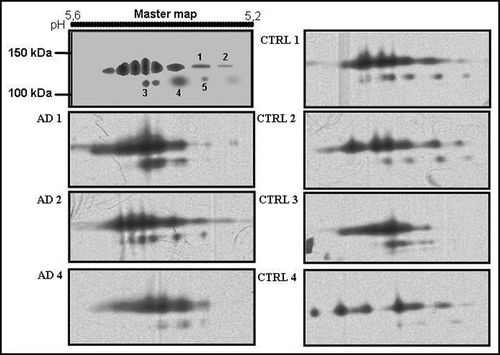
Figure 2 2D analysis of serum ceruloplasmin in AD (A) and controls (B). The pH 5–5.7 range is shown. Note the protein pattern similarity at about 135 and 115 kDa. Low-molecular-weight (<50 kDa) positive spots are present only in AD serum (A). 450 µl of a 1.5 mg/mL protein solution were loaded per gel. Ceruloplasmin content in both pools was brought 28 mg/dL and each serum sample contributed equally to the ceruloplasmin content.
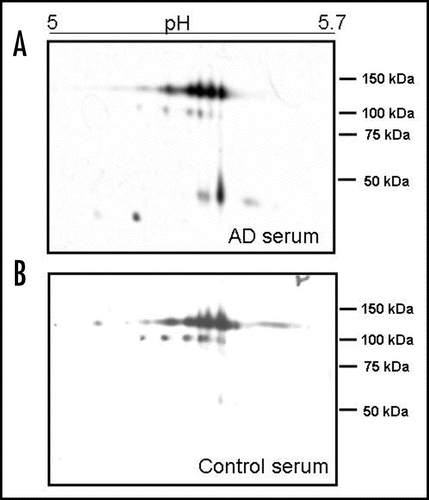
Figure 3 Box plot of the densitometric analysis (optical density percentage: % OD) of the ceruloplasmin spots immunoreactivity in controls and AD serum pools. The % ODs of the single spots obtained after the analysis of 2D PAGE films were analysed as a single “band” on the basis of their molecular weight corresponding to the 135 kDa, 115 kDa and <50 kDa forms. The sum of the 135 kDa % OD, 115 kDa % OD, 50 kDa % OD represents 100% of the ceruloplasmin immunoreactivity of a sample, which corresponds to 28 mg/dL of protein (see Methods). Black line displays the median and the box 90% of value distribution of four Western blots replicates performed in three sets of experiments. *p < 0.05.
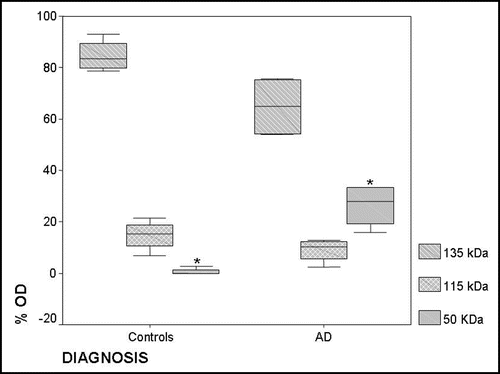
Table 1 Levels of serum copper and ceruloplasmin in controls and AD patients
Acknowledgements
The study was partially supported by grants to R.S. of the Italian Department of Health “Treatment of Alzheimer's Disease with Ammonium Tetrathiomolybdate” RF 2006, EudraCT Protocol No.: 2007-005003-18 and by a grant from the AFaR Foundation.
References
- Lovell MA, Robertson JD, Teesdale WJ, Campbell JL, Markesbery WR. Copper, iron and zinc in Alzheimer's disease senile plaques. J Neurol Sci 1998; 158:47 - 52
- Sayre LM, Perry G, Harris PL, Liu Y, Schubert KA, Smith MA. In situ catalysis by neurofibrillary tangles and senile plaques in Alzheimer's disease: a central role for bound transition metals. J Neurochem 2000; 74:270 - 279
- Barnham KJ, McKinstry WJ, Multhaup G, Galatis D, Morton CJ, Curtain CC, et al. Structure of the Alzheimer's disease amyloid precursor protein copper binding domain. A regulator of neuronal copper homeostasis. J Biol Chem 2003; 278:17401 - 17407
- Bush AI. Metal complexing agents as therapies for Alzheimer's disease. Neurobiol Aging 2002; 23:1031 - 1038
- Walshe JM. Clinical Investigations standing Committee of the association of Clinical Biochemists. Wilson's disease: the importance of measuring serum caeruloplasmin non-immunologically. Ann Clin Biochem 2003; 40:115 - 121
- Hoogenraad TU. Monography: Wilson's disease. Major Problems in Neurology 1996; 30 London W.B. Saunders
- Religa D, Strozyk D, Cherny RA, Volitakis I, Haroutunian V, Winblad B, et al. Elevated cortical zinc in Alzheimer disease. Neurology 2006; 67:69 - 75
- Molina JA, Jiménez-Jiménez FJ, Aguilar MV, Meseguer I, Mateos-Vega CJ, González-Muñoz MJ, et al. Cerebrospinal fluid levels of transition metals in patients with Alzheimer's disease. J Neural Transm 1998; 105:479 - 488
- Ozcankaya R, Delibas N. Malondialdehyde, superoxide dismutase, melatonin, iron, copper and zinc blood concentrations in patients with Alzheimer Disease: cross-sectional study. Croat Med J 2002; 43:28 - 32
- Sedighi B, Shafa MA, Shariati M. A study of serum copper and ceruloplasmin in Alzheimer's disease in Kerman, Iran. Neurology Asia 2006; 11:107 - 109
- Snaedal J, Kristinsson J, Gunnarsdóttir S, Olafsdóttir Baldvinsson M, Jóhannesson T. Copper, ceruloplasmin and superoxide dismutase in patients with Alzheimer's disease: a case-control study. Dement Geriatr Cogn Disord 1998; 9:239 - 242
- González C, Martín T, Cacho J, Breñas MT, Arroyo T, García-Berrocal B, et al. Serum zinc, copper, insulin and lipids in Alzheimer's disease epsilon 4 apolipoprotein E allele carriers. Eur J Clin Invest 1999; 29:637 - 642
- Squitti R, Lupoi D, Pasqualetti P, Dal Forno G, Vernieri F, Chiovenda P, et al. Elevation of serum copper levels in Alzheimer's disease. Neurol 2002; 59:1153 - 1161
- Smorgon C, Mari E, Atti AR, Dalla Nora E, Zamboni PF, Calzoni F, et al. Trace elements and cognitive impairment: an elderly cohort study. Arch Gerontol Geriatr Suppl 2004; 204:393 - 402
- Squitti R, Barbati G, Rossi L, Ventriglia M, Dal Forno G, Cesaretti S, et al. Excess of nonceruloplasmin serum copper in AD correlates with MMSE, CSF β-amyloid and h-tau. Neurology 2006; 67:76 - 82
- Pajonk FG, Kessler H, Supprian T, Hamzei P, Bach D, Schweickhardt J, et al. Cognitive decline correlates with low plasma concentrations of copper in patients with mild to moderate Alzheimer's disease. J Alzheimer's Dis 2005; 8:23 - 27
- Kessler H, Pajonk FG, Meisser P, Schneider-Axmann T, Hoffmann KH, Supprian T, et al. Cerebrospinal fluid diagnostic markers correlate with lower plasma copper and ceruloplasmin in patients with Alzheimer's disease. Neural Transm 2006; 113:1763 - 1769
- Squitti R, Pasqualetti P, Dal Forno G, Moffa F, Cassetta E, Lupoi D, et al. Excess of serum copper not related to ceruloplasmin in Alzheimer disease. Neurol 2005; 64:1040 - 1046
- Hoogenraad TU. Frijns CJM, Kappelle LJ, Klijn CJM. Measuring hypercupremia in blood of patients with Alzheimer's disease is logical, but the utility of measuring free-copper has to be proven. Wokke JHJ Neurologie 2007; Utrechts Tijdschrift voor 111 - 112
- Capo CR, Arciello M, Squitti R, Cassetta E, Rossini PM, Calabrese L, et al. Features of ceruloplasmin in the cerebrospinal fluid of Alzheimer's disease patients. Biometals 2008; 21:367 - 372
- Terada K, Schilsky ML, Miura N, Sugiyama T. ATP7B (WND) protein. Int J Biochem Cell Biol 1998; 30:1063 - 1067
- Shim H, Harris ZL. Genetic defects in copper metabolism. J Nutr 2003; 133:1527 - 1531
- Bielli P, Calabrese L. Structure to function relationships in ceruloplasmin: a ‘moonlighting’ protein. Cell Mol Life Sci 2002; 59:1413 - 1427
- Squitti R, Ventriglia M, Barbati G, Cassetta E, Ferreri F, Dal Forno G, et al. ‘Free’ copper in serum of Alzheimer's disease patients correlates with markers of liver function. J Neural Transm 2007; 114:1589 - 1594
- White AR, Reyes R, Mercer JF, Camakaris J, Zheng H, Bush AI, et al. Copper levels are increased in the cerebral cortex and liver of APP and APLP2 knockout mice. Brain Res 1999; 842:439 - 444
- Strozyk D, Launer LJ, Adlard PA, Cherny RA, Tsatsanis A, Volitakis I, et al. Zinc and copper modulate Alzheimer Abeta levels in human cerebrospinal fluid. Neurobiol Aging 2007; [Epub ahaed of print]
- Opazo C, Huang X, Cherny RA, Moir RD, Roher AE, White AR, et al. Metalloenzyme-like activity of Alzheimer's disease beta-amyloid. Cu-dependent catalytic conversion of dopamine, cholesterol and biological reducing agents to neurotoxic H(2)O(2). J Biol Chem 2002; 277:40302 - 40308
- Musci G, Bonaccorsi di Patti MC, Fagiolo U, Calabrese L. Age-related changes in human ceruloplasmin. Evidence for oxidative modifications. J Biol Chem 1993; 268:13388 - 13395
- McKhann G, Drachman D, Folstein M, Katzman R, Price D, Stadlan EM. Clinical diagnosis of Alzheimer's disease: report of the NINCDS-ADRDA Work Group under the auspices of Department of Health and Human Services Task Force on Alzheimer's Disease. Neurol 1984; 34:939 - 944
- Folstein MF, Folstein SE, McHugh RR. ”Mini-mental state“. A practical method for grading the cognitive state of patients for the clinician. J Psychiatr Res 1975; 12:189 - 198
- Abe A, Yamashita S, Noma A. Sensitive direct colorimetric assay for copper in serum. Clin Chem 1989; 35:552 - 554