Abstract
Our laboratory recently reported a novel prion [SWI+], in the budding yeast Saccharomyces cerevisiae. [SWI+] is the prion form of Swi1, a component of the SWI/SNF chromatin-remodeling complex. Cells harboring [SWI+] exhibit a partial loss-of-function phenotype for SWI/SNF, which can be easily assayed by poor growth on some non-fermentable carbon sources such as raffinose. Swi1 is unique among yeast prion proteins for its nuclear localization and the fact that it comprises part of a large, multi-subunit protein complex. The discovery of [SWI+] demonstrates for the first time a link between prion function and chromatin remodeling, implying a possible role for prions in gene regulation. We believe that the unique features of this novel yeast prion will provide new insight into prion biology.
The Swi1 Prion Domain
The well-characterized yeast prion proteins, Sup35, Ure2 and Rnq1, which are the determinants of [PSI+], [URE3] and [PIN+] (also known as [RNQ+]), respectively, all contain a domain characterized by an unusually high content (∼45%) of glutamine (Q) and asparagine (N) residues, in contrast to the normal yeast protein Q + N content of about 10% ().Citation2 These Q/N-rich domains are essential for de novo formation and propagation of their corresponding prions and are thus referred to as prion-forming domains (PrDs).Citation3 The N-terminal region (residues 1–524) of Swi1 is also Q/N-rich (). Similar to the PrDs of Sup35 and Ure2, the N-terminal region is dispensable for Swi1 function.Citation1 Although it remains to be tested, this suggests that the Swi1 PrD is likely contained within this 524-residue region. Intriguingly, this region contains a larger percentage of N residues than Q residues. Moreover, the N residues are concentrated within the first 300 amino acid residues, and the Q residues are primarily located within the adjacent region of ∼200 amino acids. This predominance of N over Q and the distribution pattern of Q and N are reminiscent of the Ure2 PrD ().
The unusual amino acid composition of yeast prion domains is not limited to a high Q + N content. For instance, all four prion domains have similar percentages of the hydroxy group-containing residues, including serine (S), threonine (T) and tyrosine (Y). The PrDs of Ure2 and Rnq1, and the first 524 residues of Swi1 (Swi11–524) in particular contain a noticeably large number of S residues ( and ). The prion domain of Sup35 is not enriched in S; however, it contains a larger percentage of Y. These hydroxy group-containing residues might play an important role in prionogenesis since, like Q and N, they are non-charged polar residues and good hydrogen bonders. In this regard, a recent report showed that insertion of tyrosine (Y) residues into polyQ peptides increases the fragmentation frequency and heritability of their polymers.Citation4 Another report defines glycine (G), S, Y, N and Q as “poor protectors,” which are more likely to be present in highly vulnerable, or aggregation-prone, proteins.Citation5 According to this study, these five amino acids do not “protect” the hydrogen backbone of the polypeptide chain, leading to increased vulnerability, or propensity to misfold and aggregate. Although the composition of the putative Swi1 prion domain overall correlates well with the PrDs of Sup35, Ure2 and Rnq1, Swi11–524 seems to have more in common with the Ure2 PrD than those of Sup35 and Rnq1. For example, Swi11–524 and Ure21–89 do not contain oligopeptide repeats (). Moreover, Ure21–89 and Swi11–524 also contain some bulky hydrophobic residues, such as isoleucine (I) and valine (V), which are completely lacking in the PrDs of Sup35 and Rnq1. Although the significance of these observations remains to be seen, these features suggest that Swi11–524 is more reminiscent of Ure2 PrD than those of Sup35 and Rnq1. We believe a more detailed investigation of the Swi1 PrD will yield further insight into sequence requirements for prionogenesis.
The Impact of [SWI+] on SWI/SNF Function
It has been proposed that yeast prions, as non-Mendelian genetic elements, provide some feature that is adaptive for the organism.Citation6 This scenario is easily plausible: first, like nucleic acid-based mutation, prion formation can cause heritable phenotypic changes, some of which may be advantageous; second, such a change can be reversible since prion elements can be formed and lost without altering the underlying nucleic acids; third, since prions are infectious proteins, prion-conferred phenotypic traits are dominant and thus can provide a “quick fix” for organisms to cope a sudden undesirable environmental changes; fourth, both yeast and mammalian prion proteins are capable of adopting multiple stable conformations, (“strains” or variants in the case of yeast) and thus provide a multi-level epigenetic regulation. Lastly, when a prion protein enters an aggregated prion conformation, it may sequester other important cellular factors, resulting in a complex phenotype of partial loss-of-function of multiple genes. Taken together, the stable yet reversible prion inheritance offers a potential for regulatory plasticity that cannot be achieved by nucleic acid mutation.
In support of this hypothesis, [PSI+] was shown to be advantageous under some experimental conditions.Citation7,Citation8 The finding that [RNQ+] exists in some clinical isolates and wild strains of S. cerevisiae seems to be in agreement with the idea that prions might have some adaptive feature that is beneficial to the yeast cells.Citation9,Citation10 Intriguingly, [PSI+] and [URE3] have not yet been found to exist in wild S. cerevisiae strains. This absence has been used as a strong argument that these two prions are diseases instead of being beneficial.Citation9,Citation11 In contrast, the biological function of the [Het-s] prion in heterokaryon incompatibility of the filamentous fungus,Citation12 Podospora anserina, is well recognized.Citation13–Citation15 Given the potential for a biological role for yeast prions, what are the implications of [SWI+] for the function of the SWI/SNF complex? Can the prion-mediated conformational change of Swi1 play a regulatory role in transcription? We have shown that cells bearing [SWI+] exhibit a partial loss-of-function phenotype of SWI/SNF, indicating that the [SWI+] prion conformation greatly reduces the ability of Swi1 to act as a chromatin-remodeling factor. Although [SWI+] cells exhibit disadvantageous phenotypes such as poor growth and of the inability to use alternative carbon sources, the idea that [SWI+] might be beneficial to the cells under some undefined environmental conditions remains appealing. While SWI/SNF primarily functions as a transcriptional activator, it has also been shown to represses transcription of several genes.Citation16 As the SWI/SNF complex is a global regulator in gene expression, a decrease in SWI/SNF function could contribute to an increase or decrease in transcription of a subset of genes comprising up to 6% of the genome. In S. cerevisiae, there is a second SWI/SNF-like complex called RSC (remodels the structure of chromatin). RSC is composed of subunits homologous to those of SWI/SNF, and in addition shares several subunits with SWI/SNF. Specifically, Arp7, Arp9 and Rtt102 have been shown to interact with both SWI/SNF and RSC.Citation17,Citation18 It is therefore possible that [SWI+] affects not only SWI/SNF function, but also RSC function.
Although [SWI+] variants, or “strains”, have not yet been identified, the possible existence of [SWI+] variants would confer an even finer level of control of transcriptional regulation, based on the relative strength or weakness of the variant. Upon extreme environmental changes, the formation of [SWI+] could not only turn “on” or “off” but also fine-tune the expression of SWI/SNF target genes, resulting in an altered transcriptional landscape that might aid the cells in coping with harmful environmental changes. This prion-mediated phenotypic plasticity could be an important tool for cells to survive temporary environmental stresses. Although it is an attractive hypothesis that [SWI+] formation might be beneficial to the cells, experimental proofs are needed to validate this prediction.
Unique Aspects of [SWI+]
A particularly interesting feature of Swi1 that sets it apart from other known yeast prions is that it is a nuclear protein. Sup35, Rnq1 and Ure2 are all cytoplasmic proteins, and are also very abundant in a normal cell (). In contrast, Swi1 is typically expressed at only around 250 copies per cell (). These unique features could help researchers understand how prions form de novo. It has been demonstrated that overexpression of a prion protein increases the rate of misfolding and thus results in a higher frequency of prion conversion.Citation19–Citation21 One would think that [SWI+] would therefore arise less frequently than other prion elements because of its low abundance. In fact, inheritable Swi1 aggregates can be formed without Swi1 overproduction.Citation1 This suggests that Swi1 may be more aggregation-prone than other prion proteins.
Another unique feature of Swi1 is that its human homologue is important in disease. The human homologue of SWI/SNF is the BAF complex, which contains one of two Snf2 homologues, named BRM (Brahma) and BRG1 (Brahma-related gene).Citation22–Citation24 The BAF proteins (BRG1-associated factors) are homologous to subunits of the yeast complex.Citation25 BAF250 (also known as ARID1A) is homologous to Swi1, and is responsible for directing the complex to target promoters. The genomic region encoding BAF250 is one of the most frequently deleted regions across all cancer typesCitation26 and knockdown of BAF250 results in a failure of cell cycle arrest.Citation27 Interestingly, BAF250 has a Q- and N-rich N-terminus that is reminiscent of yeast prion domains. BRM also contains regions that are noticeably Q-rich. Although it remains to be tested whether these components of human SWI/SNF-like complexes are able to adopt prion-like conformations, the discovery of [SWI+] indicates possible implications for human health and disease.
One interesting finding from our recent studies is that the presence of [PSI+] enhances the transmissibility of [SWI+]. When [SWI+] is cytoduced into [swi−] cells, the Raf− phenotype is acquired at a rate of about 49%. When [SWI+] is cytoduced from a [SWI+][PSI+] cell, however, the rate of transmission increases to about 89%.Citation1 In cells containing both [PSI+] and [SWI+], curing of [PSI+] by Hsp104 overexpression also resulted in a high rate of [SWI+] loss. Can these phenomena be explained by cross-seeding? What is the nature of the relationship between [SWI+] and [PSI+]? Further studies are required to investigate the interaction between [SWI+], [PSI+] and other prions.
How Common is the Prion Phenomenon?
An outstanding question in prion biology that remains to be answered is: how widespread is the prion phenomenon? Michelitsch and Weissman identified about 100 ORFs in S. cerevisiae as prion candidates based on the criterion of containing ≥30 Q + N within 80 consecutive amino acid residues.Citation28 Through an unbiased genetic screen, Derkatch et al. subsequently identified 11 ORFs that can function as Pin+, a factor or an event that can promote [PSI+] de novo formation upon overexpression of SUP35.Citation29 Remarkably, all 11 ORFs identified by this screen contain a Q/N-rich region. Among these ORFs are Ure2, a known prion protein, and New1, another protein that cannot exist as a prion by itself but can form a heritable prion when its Q/N-rich region is fused with the functional domain of Sup35.Citation30 It remains unknown how many of the ∼100 Q/N-rich ORFs in S. cerevisiae are real prion proteins. To test each candidate individually using conventional methodology is highly time-consuming, with no guarantee of success. Since the Michelitsch and Weissman study was published in 2000, [SWI+] is the only candidate whose identity as a prion has been confirmed (while this article was in its final step in print, identification of a new prion [MCA] was reported by Nemecek et al.).Citation32 A well-designed, systematic screening tactic will be helpful in identifying potential prion proteins. Obviously, being Q/N-rich alone is neither necessary nor sufficient to be qualified as a prion protein, as the mammalian PrP and Podospora anserina HET-s do not contain significant Q/N-rich sequences. Moreover, polyQ polypeptides and some very Q/N-rich proteins are not able to adopt a prion conformation, including the yeast Snf5 protein, which we tested previously.Citation1 A recent report shows that the [Het-s] prion can form de novo and be propagated in S. cerevisiae,Citation31 indicating that S. cerevisiae is fully capable of harboring non-Q/N-rich prions. Thus, it is likely that the S. cerevisiae proteome contains non-Q/N-rich prion proteins that remain to be identified.
A significant number of the prion protein candidates in S. cerevisiae are transcription factors,Citation28 suggesting that prion-like conformational changes play an important role in influencing gene expression. The functions of the Q/N-rich regions in these transcription factors are poorly characterized. Moreover, like Swi1, a considerable number of these Q/N-rich transcription factors are chromatin-remodeling factors. Is prion-mediated epigenetic regulation of chromatin-based epigenetic regulation a widespread phenomenon? This “double” epigenetic regulation could have critical biological importance.
Conclusions
We anticipate that the novel prion, [SWI+], will continue to provide unique opportunities to explore key issues in prion biology. The predominance of asparagine residues in the putative Swi1 prion domain, the high serine and threonine content, inclusion of bulky hydrophobic residues, and lack of oligopeptide repeats, have interesting implications for redefining the characteristics of yeast prion domains. Furthermore, the nuclear localization and low abundance of the Swi1 protein should provide further insight into de novo prion formation. The interaction of Swi1 with multiple SWI/SNF complex components, as well as transcriptional activators, has the potential to elucidate the relationship between prion aggregates and cellular proteins, and possibly to define an in vivo role for this prion. Finally, the relationship of [SWI+] with [PSI+] and other yeast prions will yield important insight into the nature of prion aggregation and transmissibility.
We suspect that prion phenomenon is more widespread than we have realized and we anticipate that more novel prions will be identified from S. cerevisiae and other organisms. The existence of Q/N-rich SWI/SNF component homologues in humans make [SWI+] an ideal system for exploring this link. Continuing work on [SWI+] and other yeast prions will facilitate the more accurate prediction of prion candidates and perhaps begin to illuminate the role of these unique genetic elements, whether beneficial or harmful, in nature.
Figures and Tables
Figure 1 Amino acid sequences of prion domains of known prions. Asparagine (N) residues are highlighted in blue, glutamine (Q) residues are highlighted in purple, and serine (S), tyrosine (Y) and threonine (T) are highlighted in red. Underlined areas indicate the imperfect oligopeptide repeats in Sup35 and Rnq1.
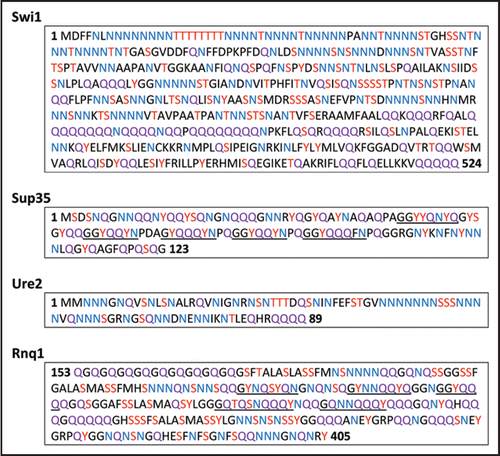
Table 1 Percentage of amino acids present in prion domains of known yeast prions
Table 2 Swi1 is unique among yeast prions proteins due to its nuclear localization and low cellular abundance
References
- Du Z, Park KW, Yu H, Fan Q, Li L. Newly identified prion linked to the chromatin-remodeling factor Swi1 in Saccharomyces cerevisiae. Nat Genet 2008; 40:460 - 465
- Echols N, Harrison P, Balasubramanian S, Luscombe NM, Bertone P, Zhang Z, et al. Comprehensive analysis of amino acid and nucleotide composition in eukaryotic genomes, comparing genes and pseudogenes. Nucleic Acids Res 2002; 30:2515 - 2523
- Tuite MF. Yeast prions and their prion-forming domain. Science 2000; 287:562 - 563
- Alexandrov IM, Vishnevskaya AB, Ter-Avanesyan MD, Kushnirov VV. Appearance and propagation of polyglutamine-based amyloids in yeast: Tyrosine residues enable polymer fragmentation. J Biol Chem 2008; 283:15185 - 15192
- Chen J, Liang H, Fernandez A. Protein structure protection commits gene expression patterns. Genome Biol 2008; 9:107
- Lindquist S. Mad cows meet psi-chotic yeast: The expansion of the prion hypothesis. Cell 1997; 89:495 - 498
- True HL, Berlin I, Lindquist SL. Epigenetic regulation of translation reveals hidden genetic variation to produce complex traits. Nature 2004; 431:184 - 187
- True HL, Lindquist SL. A yeast prion provides a mechanism for genetic variation and phenotypic diversity. Nature 2000; 407:477 - 483
- Nakayashiki T, Kurtzman CP, Edskes HK, Wickner RB. Yeast prions [URE3] and [PSI+] are diseases. Proc Natl Acad Sci USA 2005; 102:10575 - 10580
- Resende CG, Outeiro TF, Sands L, Lindquist S, Tuite MF. Prion protein gene polymorphisms in Saccharomyces cerevisiae. Molecular Microbiology 2003; 49:1005 - 1017
- Wickner RB, Shewmaker F, Kryndushkin D, Edskes HK. Protein inheritance (prions) based on parallel in-register beta-sheet amyloid structures. Bioessays 2008; 30:955 - 964
- Coustou V, Deleu C, Saupe S, Begueret J. The protein product of the het-s heterokaryon incompatibility gene of the fungus Podospora anserina behaves as a prion analog. Proc Natl Acad Sci USA 1997; 94:9773 - 9778
- Osherovich LZ, Weissman JS. The utility of prions. Dev Cell 2002; 2:143 - 151
- Ter-Avanesyan MD, Kushnirov VV. Prions: Infectious proteins with genetic properties. Biochemistry (Mosc) 1999; 64:1382 - 1390
- Wickner RB. A new prion controls fungal cell fusion incompatibility. Proc Natl Acad Sci USA 1997; 94:10012 - 10014
- Martens JA, Winston F. Evidence that Swi/Snf directly represses transcription in S. cerevisiae. Genes Dev 2002; 16:2231 - 2236
- Cairns BR, Erdjument-Bromage H, Tempst P, Winston F, Kornberg RD. Two actin-related proteins are shared functional components of the chromatin-remodeling complexes RSC and SWI/SNF. Mol Cell 1998; 2:639 - 651
- Graumann J, Dunipace LA, Seol JH, McDonald WH, Yates JR 3rd, Wold BJ, et al. Applicability of tandem affinity purification MudPIT to pathway proteomics in yeast. Mol Cell Proteomics 2004; 3:226 - 237
- Chernoff YO, Derkatch IL, Inge-Vechtomov SG. Multicopy SUP35 gene induces de-novo appearance of psi-like factors in the yeast Saccharomyces cerevisiae. Curr Genet 1993; 24:268 - 270
- Masison DC, Wickner RB. Prion-inducing domain of yeast Ure2p and protease resistance of Ure2p in prion-containing cells. Science 1995; 270:93 - 95
- Sondheimer N, Lindquist S. Rnq1: An epigenetic modifier of protein function in yeast. Mol Cell 2000; 5:163 - 172
- Chiba H, Muramatsu M, Nomoto A, Kato H. Two human homologues of Saccharomyces cerevisiae SWI2/SNF2 and Drosophila brahma are transcriptional coactivators cooperating with the estrogen receptor and the retinoic acid receptor. Nucleic Acids Res 1994; 22:1815 - 1820
- Khavari PA, Peterson CL, Tamkun JW, Mendel DB, Crabtree GR. BRG1 contains a conserved domain of the SWI2/SNF2 family necessary for normal mitotic growth and transcription. Nature 1993; 366:170 - 174
- Muchardt C, Yaniv M. A human homologue of Saccharomyces cerevisiae SNF2/SWI2 and Drosophila brm genes potentiates transcriptional activation by the glucocorticoid receptor. EMBO J 1993; 12:4279 - 4290
- Wang W, Xue Y, Zhou S, Kuo A, Cairns BR, Crabtree GR. Diversity and specialization of mammalian SWI/SNF complexes. Genes Dev 1996; 10:2117 - 2130
- Mertens F, Johansson B, Hoglund M, Mitelman F. Chromosomal imbalance maps of malignant solid tumors: A cytogenetic survey of 3185 neoplasms. Cancer Res 1997; 57:2765 - 2780
- Nagl NG Jr, Patsialou A, Haines DS, Dallas PB, Beck GR Jr, Moran E. The p270 (ARID1A/SMARCF1) subunit of mammalian SWI/SNF-related complexes is essential for normal cell cycle arrest. Cancer Res 2005; 65:9236 - 9244
- Michelitsch MD, Weissman JS. A census of glutamine/asparagine-rich regions: Implications for their conserved function and the prediction of novel prions. Proc Natl Acad Sci USA 2000; 97:11910 - 11915
- Derkatch IL, Bradley ME, Hong JY, Liebman SW. Prions affect the appearance of other prions: The story of [PIN+]. Cell 2001; 106:171 - 182
- Santoso A, Chien P, Osherovich LZ, Weissman JS. Molecular basis of a yeast prion species barrier. Cell 2000; 100:277 - 288
- Taneja V, Maddelein ML, Talarek N, Saupe SJ, Liebman SW. A non-Q/N-rich prion domain of a foreign prion, [Het-s], can propagate as a prion in yeast. Mol Cell 2007; 27:67 - 77
- Nemecek J, Nakayashiki T, Wickner RB. A prion of yeast metacaspase homolog (Mca1p) detected by a genetic screen. Proc Natl Acad Sci USA 2009; 106:1892 - 1896