Abstract
Over the past decade, immunohistochemical studies have provided compelling evidence that gray matter (GM) pathology in multiple sclerosis (MS) is extensive. Until recently, this GM pathology was difficult to visualize using standard magnetic resonance imaging (MRI) techniques. However, with newly developed MRI sequences, it has become clear that GM damage is present from the earliest stages of the disease and accrues with disease progression. GM pathology is clinically relevant, as GM lesions and/or GM atrophy were shown to be associated with MS motor deficits and cognitive impairment. Recent autopsy studies demonstrated significant GM demyelination and microglia activation. However, extensive immune cell influx, complement activation and blood-brain barrier leakage, like in WM pathology, are far less prominent in the GM. Hence, so far, the cause of GM damage in MS remains unknown, although several plausible underlying pathogenic mechanisms have been proposed. This paper provides an overview of GM damage in MS with a focus on its topology and histopathology.
Introduction
Multiple sclerosis (MS) is a chronic, demyelinating disease of the central nervous system (CNS) that typically affects young adults. The disease is expressed in different clinical phenotypes,Citation1 the most common of which is relapsing-remitting MS (RRMS), which is characterized by periodical relapses and remissions of neurological impairment. A large proportion of RRMS patients (30–40%) convert to secondary progressive (SP) MS within 10 y.Citation2 SPMS is characterized by a steady progression of neurological decline in the relative absence of relapses. The estimated median conversion time of RRMS onset to SPMS is about 19 y.Citation2 Approximately 15–20% of the MS cases are afflicted with primary progressive (PP) MS, which shows a steady progression generally without relapses from the onset of the disease.Citation3 However, still a minority (28%) of PPMS patients suffer from relapses of neurological impairment even decades after clinical onset of the disease.Citation4
MS has long been considered an auto-immune disease primarily affecting the white matter (WM).Citation5 However, it has become increasingly clear that gray matter (GM) pathology is an important aspect of the disease, as well. In 1962, Brownell and Hughes described GM lesions in a selected set of MS autopsy cases. The authors used conventional immunohistochemistry [Luxol Fast-Blue (LFB) or Klüver-Barrera] and detected a total of 1,595 lesions in 22 MS cases, of which 26% were located in the cortical GM or at the border of the WM and GM. Of these 26% of GM lesions, 17% were mixed GM/WM lesions and only 5% were found to be restricted to the cortex.Citation6 Later studies, however, using more sensitive immunohistochemical stainings with antibodies against proteolipid protein (PLP) and myelin basic protein (MBP), showed that GM demyelination was much more extensive.Citation7,Citation8 GM lesions were provisionally classified, based on their location within the cortex, into 4 different types (). Type I lesions are mixed GM/WM lesions, which are also visible on conventional histochemistry; Type II lesions are mostly located around cortical blood vessels; Type III lesions are subpial lesions and represent the most common type of cortical GM lesions; Type III lesions reach from the pial surface downwards into the cortex and may cover multiple gyri.Citation9 Finally, type IV lesions are large, cortex-spanning lesions covering all six layers of the cortex, while never reaching into the WM.Citation7,Citation8
Figure 1.Classification of GM lesions as described by Bö et al.Citation8 Type I lesions (A) are GM/WM mixed lesions (original magnification 2.5x). The black line indicates the border between WM and GM. The GM and WM parts of the lesion are indicated with an arrow and arrowhead, respectively. The red arrowhead indicates a WM lesion. Type II lesions (B) are located completely intracortically, while type III and IV lesions run from the pial surface toward deeper cortical layers (D and E) or all the way to the GM/WM border, respectively (C; original magnification 5x). Both D en E are sections showing the same type III lesion. The lesion is visible in the MBP staining (D), showing a clearly demyelinated area (arrows indicate lesion borders), while the same area is invisible in a consecutive slide stained with a more conventional histochemical staining (E; Luxol Fast-Blue). The original magnification is 5x. (A-D), sections stained using myelin basic protein (MBP) antibodies. (E) Section stained using LFB.
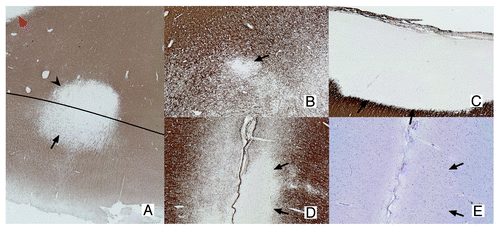
Topology of GM pathology in MS
Immunohistochemical analysis did not only reveal extensive demyelination of the neocortex, but also of archicortical structures (such as the hippocampus) and of deep GM structures like the thalamus, putamen, globus pallidus, caudate, claustrum, hypothalamus, substantia nigra, amygdala and of the cerebellum and spinal cord.Citation5,Citation7,Citation9-Citation21 provides a detailed overview of studies reporting on the topology of GM pathology in MS. Interestingly, in a subpopulation of MS patients the extent of GM demyelination seems to exceed that of WM demyelination,Citation5,Citation7,Citation9,Citation12,Citation13,Citation16,Citation19 and GM pathology was reported to increase significantly during the progression of the disease. Kutzelnigg et al.Citation9 investigated the extent and distribution of focal WM lesions, diffuse WM and GM lesions and their relation with inflammation in 11 acute MS, 6 RRMS, 15 PPMS and 20 SPMS. SPMS and PPMS distinguished itself by diffuse abnormalities, with reactive microglia, in the WM and extensive cortical demyelination. The cortical demyelination generally did not correlate with the number of WM lesions in that study. Acute MS and RRMS had less profound cortical demyelination but a high number of focal WM lesions. Vercellino et al.Citation19 also reported that extent of GM demyelination between patients varies. Two out of three SPMS patients within their study showed significantly more GM lesions than the three RRMS patients they examined. Of the brain areas that Vercellino and colleagues investigated, the cingulated gyrus was especially affected. In addition, the patients with most prominent GM demyelination showed neuronal loss and signs of apoptosis, as indicated by caspase-3 staining.
Table 1. topology of GM pathology in MS
Another brain area with extensive cortical demyelination is the cerebellum.Citation16 About 38.8% of the cerebellar cortex was found to be demyelinated in 19 SPMS patients and 36.9% in 10 PPMS patients.Citation16 One of the patients in this study showed near-total loss of myelin in up to 92% of analyzed cerebellar cortex. Cerebellar demyelination in this study exhibited a band-like pattern, involving multiple cerebellar folia, and did not correlate with WM demyelination within the same samples. Some axonal spheroids were found in the cerebellar samples, as well as a reduction of Purkinje cells, both signs of ongoing neurodegeneration.Citation16
Although the majority of the cortical lesions are subpially located (i.e., Type III lesions), lesions in deep GM structures, spinal cord and hippocampus are generally mixed GM/WM lesions, and may be slightly more inflammatory.Citation11-Citation14,Citation17,Citation21 Gilmore and colleaguesCitation13 investigated the extent of GM demyelination and the morphology of the lesions in the cerebellum, motor cortex, cingulated gyrus, thalamus and in different levels of the spinal cord. GM demyelination was most extensive in the spinal cord and cerebellum. The authors noted that the majority of GM lesions in cerebellum and cerebrum were subpial, while in the spinal cord, a proportionally higher number of lesions was mixed. A much smaller portion of the spinal lesions expanded within the GM while strictly respecting the GM/WM boundary. In addition, almost all spinal cord lesions were chronic, i.e., without overt signs of inflammation.Citation12
A recent post-mortem study specifically investigated deep GM demyelination in 14 MS patients.Citation21 Most of the lesions were found in the thalamus and caudate nucleus, but they were also present in the putamen, pallidum, claustrum, amygdala, hypothalamus and substantia nigra. Just like spinal cord lesions, lesions within deep GM structures are mostly mixed. Especially the WM part of these lesions may show activated microglia, foamy macrophages or perivascular lymphocytes. Moreover, neuronal loss was observed in both demyelinated and normally myelinated deep GM structures.
Another non-neocortical area with prominent GM pathology is the hippocampus,Citation11,Citation17 a structure that is essential to memory function.Citation22,Citation23 A post mortem study found extensive hippocampal demyelination in 15 out of 19 chronic MS patients.Citation11 Several types of intrahippocampal and mixed intrahippocampal-perihippocampal lesions were found and the largest mixed lesions correlated with cognitive decline (as derived from retrospective medical chart analysis). Hippocampal lesions were found to be large and anatomical borders were generally not spared by these lesions. Moderate microglial activation was frequently found, but mostly at the edges of the lesions. Isolated intrahippocampal lesions were generally smaller and affected specific hippocampal subregions, although the Cornu Ammonis (CA) 2 subregion and the hilus of the dentate gyrus were mostly spared.Citation11 Another study found hippocampal demyelination in 28 of 45 chronic MS patients examined.Citation17 The majority of the lesions were chronic, with a few areas of activated microglia. In addition, this study provided a detailed, quantitative analysis of the extent of tissue atrophy and neuronal loss. The average cross-sectional area of the hippocampus was reduced by 22%, indicating significant hippocampal atrophy. The same study found a reduction of 30% of neurons in the CA3-2 regions and 27% in the CA1 region. Also, the size of the neurons was decreased in CA1. Interestingly, Dutta and colleaguesCitation10 did not find neuronal loss in demyelinated hippocampi. Instead, they reported significant decreases in synaptic density and neuronal proteins that are essential for axonal transport, synaptic plasticity, glutamate neurotransmission, glutamate homeostasis and memory/learning.
Detection of GM pathology with MRI
Detection of cortical GM lesions with conventional T2-weighted MRI has proven difficult, since the cortex has intrinsically low myelin densities and demyelination of the cortex generates little contrast on MRI. In addition, cortical GM lesions may be small and partial volume effects with cerebrospinal fluid in the sulci may interfere with lesion identification. The introduction of more advanced imaging techniques, like the fluid-attenuated inversion recovery (FLAIR) MR sequence, have greatly improved lesion detection in (juxta)cortical areas.Citation24 However, the sensitivity of these techniques to purely intracortical lesions is still rather poor.Citation25 Double inversion recovery (DIR) was shown to have a higher sensitivity to intracortical lesions than FLAIR and conventional T2-weighted imaging.Citation26 However, post mortem evaluation uncovered that a staggering 80% of cortical lesions still remain undetected on DIR.Citation27 Visualization of cortical GM lesions in vivo may require the combination of several new techniques, such as DIR, phase-sensitive inversion recovery (PSIR)Citation28 and/or 3D T1-based techniquesCitation29 in the future. Alternatively, higher magnetic field-strengths may be helpful in assessing cortical GM lesions in MSCitation30,Citation31 ().
Figure 2. Post-mortem MRI and accompanying PLP immunohistochemical staining of a coronal section. T2 weighted MRI scans (B) were compared with PLP stainings (A) of the same coronal sections. This figure clearly shows the difficulty to detect cortical lesions with conventional MRI sequences. (A): MRI-visible cortical lesions are shown in red, while MRI-invisible cortical lesions are shown in blue (original magnification 0.7x); (B1): MRI-visible lesions are indicated with arrowheads. MRI-visible cortical lesions show only very subtle increase of signal intensity; (B2) MRI-invisible lesions. Reproduced from Seewann et al.,Citation25 with permission from SAGE Publishers.
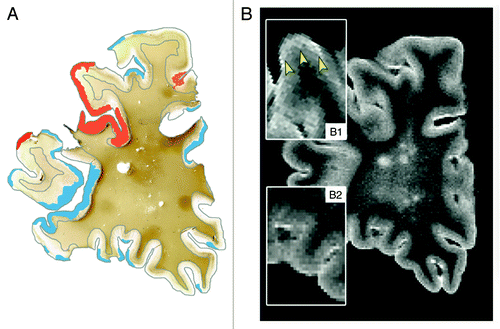
Clinical relevance of GM pathology
Both cortical GM lesions and GM atrophy are already prominent in the early stages of MS, and are predictive of disease progression.Citation32-Citation43 Early involvement of cortical GM pathology has been demonstrated in all MS phenotypes, as well as in clinically isolated syndrome (CIS) patients.Citation36 Both GM lesion volume and the number of GM lesions are known to increase over time in patients that worsen faster compared with patients that are clinically stable.Citation39 The number of GM lesions in the primary motor cortex correlates strongly with the severity of motor dysfunction.Citation40 In addition, overall GM lesion loads are associated with overall cognitive impairment,Citation41 while specific cortical and hippocampal lesions are strongly associated with impaired visuospatial memory and processing speed.Citation42,Citation43 Finally, GM atrophy of specific brain areas, including thalamus, superior frontal gyrus and cerebellum correlates with disease progression.Citation32-Citation35 Whereas the detection of cortical lesions is difficult using conventional MRI sequences,Citation44 overall GM atrophy measurements provide robust and reliable results based on standard MRI sequences.Citation45 GM atrophy rate was reported to increase dramatically in SPMS compared with RRMS patients, while WM atrophy rates remained similar over time.Citation46,Citation47 Therefore, observed whole-brain atrophy in chronic MS may be mostly driven by GM atrophy. The pathobiological mechanisms leading to GM atrophy are currently poorly understood, but the idea that GM atrophy is generated by a combination of neurite transaction, synapse loss and demyelination seems plausible.Citation20
What causes GM demyelination and GM atrophy?
Classification and visualization of GM pathology have received much attention over the last years, but the pathogenesis of GM pathology is still unclear. MS autopsy studies, which usually deal with tissue from progressive MS patients, report extensive signs of inflammation in the WM, including lymphocyte infiltration, complement deposition and blood-brain barrier disruption.Citation8,Citation48,Citation49 Inflammation was also reported in biopsy material of atypical MS cases.Citation50 However, large screenings of autopsy material have revealed that GM demyelination is typically not associated with inflammation.Citation8,Citation19 Apart from demyelination, subtle neuroaxonal degeneration, neurite transection and synapse loss was found in cortical MS lesions.Citation18 Microglia may play a role in cortical MS pathology,Citation18 as rims of activated microglia were found surrounding type III cortical lesions in patients with a more severe disease course.Citation51 In vivo imaging of microglia activation by 11C-PK11195 labeling revealed extensive regional activation of microglia in MS cortex.Citation52 Moreover, 11C-PK11195 labeling was increased by 60% in RRMS patient and by almost 100% in SPMS patients compared with healthy controls. Increased 11C-PK11195 binding correlated with clinical disability in SPMS patients, but not in RRMS patients. However, so far, it is unclear whether these microglia have a predominantly (neuro)protective or a proinflammatory, neurotoxic effect. Microglia are thought to contribute to tissue repair neuronal and myelin damage,Citation53,Citation54 but can also be responsible for neuronal death and are often involved in pathogenic processes in neurodegenerative diseases.Citation55-Citation57
Thus, the initial stages of MS are characterized by inflammation in the WM and possibly also the GM. In the progressive stage, however, new inflammatory WM lesions appear less frequently and inflammatory GM lesions have rarely been reported.Citation8,Citation48,Citation49 Anti-inflammatory therapies currently used for the treatment of MS are partly effective in the early stage of MS but are of limited efficacy when patients have already entered the progressive phase.Citation58 The progressive phase is probably dominated by cytodegenerative processes (neurons, axons, oligodendrocytes), which continue to develop at least partly independently from demyelination.Citation20 This was emphasized in a detailed quantitative post mortem study of normal myelinated cortex and cortical lesions in 22 chronic MS patients investigated cortical thinning and neuronal, glial and synapse loss in cortical lesions and normal myelinated cortex.Citation20 The mean neuronal (10%), synapse (47%) and glia (36%) density was reduced by 10% in type I lesions compared with normally myelinated cortex. Interestingly, the neurons in the normally myelinated cortex had lost polarization, perhaps due to loss of dendrites and axons. Furthermore, a mean cortical thinning of 10% was found independently of cortical lesions, suggesting that there are mechanisms contributing to cortical atrophy besides cortical demyelination.
Regarding GM pathogenesis, several theories exist. One of the leading ideas has been that cortical GM demyelination may be due to meningeal inflammation and associated secretion of myelinotoxic factors (cytokines, chemokines) by meningeal immune cell aggregates.Citation59 Several results support this idea: substantial meningeal inflammation was convincingly shown in MS, sometimes associated with the formation of ectopic B cell follicle-like structures, possibly infected by Epstein-Barr virus.Citation59-Citation61 Patients with extensive meningeal inflammation and ectopic B cell follicle-like structures were found to have more GM demyelination and a gradual loss of neurons in cortical layers I-IV.Citation62 However, it should be noted that patients with ectopic B cell follicle-like structures also had a more aggressive disease course, with an earlier age at clinical onset and death.Citation59 Moreover, Kooi et al.Citation63 found that a one-on-one spatial correlation between meningeal inflammatory infiltrates and cortical demyelination was absent in progressive MS cases, which emphasizes the need for further investigation into the causal relation between meningeal inflammation and cortical demyelination and/or neurodegeneration in MS ().
Figure 3. Meningeal inflammation in MS. Immunostainings of MS brain sections show intrameningeal ectopic B-cell follicle like structures in a cerebral sulcus containing CD20+ B cells (A), ramified stromal cells/FCD expressing CD35 (inset A) and expressing CXCLI3 (B), Ki67+ proliferating B cells (C), and plasmablast/plasma cells stained with an anti- Ig-G, -A, -M polyclonal antibody (D; the inset shows two intrafollicular plasma cells at high magnification). CD20+ B cells are also present in periventricular WM lesions (E) and in scarcely inflamed meninges entering a cerebral sulcus in a SPMS (F) and PPMS case (G). The lower schematic picture illustrates the localization of ectopic B-cell follicle like structures in the MS brain. These follicle like structures are present along (H) and in the depth (I) of the cerebral sulci, whereas scattered B lymphocytes (J) are detected in the meninges covering the external brain surface. Original magnifications: E-G = 100x; A, D, H-J = 200x, B, C and insets in A and D = 400x. Reproduced from Magliozzi et al.Citation59 with permission from Oxford University Press.
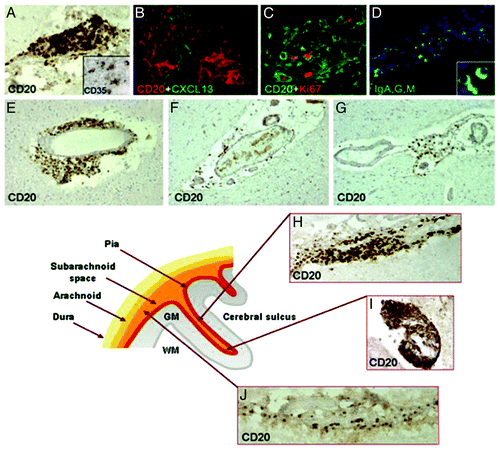
WM demyelination and axon transection might also contribute to GM pathology, e.g., via retrograde myelinoaxonal degradation. Retrograde degradation could lead to demyelination and degeneration of the axons in the GM, which could subsequently become evident as a GM lesion.
Demyelination hampers the transduction of electric signals along axons and the damaged axons redistribute and upregulate ion channels (Nav1.2 and Nav1.6) to counterbalance impaired signal propagation.Citation64 However, this requires increased adenosine triphospate (ATP) levels, which dysfunctioning mitochondria cannot produce.Citation65 Mitochondrial dysfunction is probably caused by free oxygen radicals produced during inflammation by immune cells in the WM, but also by the dysfunctioning mitochondria themselves.Citation66,Citation67 The imbalance between increased energy demand and decreased energy supply has been termed “virtual hypoxia.”Citation68,Citation69 A state of virtual hypoxia leads to excessive amounts of intracellular calcium (Ca2+) due to reversed Na+/Ca2+ exchangers and activation of apoptotic Ca2+ dependent signaling pathways, which eventually leads to degeneration of the axon in the WM.
Glutamate excitotoxicity may also activate Ca2+ dependent intracellular signaling pathways. Several studies report alterations in glutamate homeostasis.Citation70-Citation72 MS brains show excessive expression of ionotropic and metabotropic glutamate receptors on axons, oligodendrocytes, astrocytes and macrophages/microglia.Citation70-Citation72 Overactivation of glutamate receptors in combination with downregulation of glutamate transporters may finally lead to opening of voltage gated Ca2+ channels and cause an elevated internal release of Ca2+.Citation69
Another pathological process of relevance might be selective vulnerability of specific cell populations as is observed in other neurodegenerative diseases, like e.g., Alzheimer and Parkinson disease. Recently, an imbalance in the cholinergic neurotransmitter system within the hippocampus has been described.Citation15 Both the expression and activity of the acetylcholine producing enzyme, choline acetyltransferase (ChAT) and the degrading enzyme, acetylcholinesterase (AChE) were compared with Alzheimer disease (AD) patients and non-neurological controls (NC). Compared with AD and controls, ChAT expression was decreased in MS, while the AChE levels and activity remained the same in MS hippocampi. This in turn may cause reductions of available acetylcholine in the synaptic cleft. Moreover, this imbalance was independent of demyelination, thus once more underscoring the relative independence of neurodegeneration and demyelination.
Conclusion and future prospects
The last few years have shown that MS pathology critically involves the GM. GM pathology is extensive and manifests throughout all phases of the disease. Demyelination of the GM has been found in the neocortex, hippocampus, spinal cord and deep GM structures, and has been reported to increase significantly with progression of disease. The majority of cortical GM lesions are subpial (Type III) lesions, while lesions in the deep GM structures, spinal cored and hippocampus are generally mixed GM/WM lesions. Interestingly, some of these mixed lesions are inflammatory, while most pure GM lesions are not.
Although it was initially difficult to visualize GM pathology in vivo, newer imaging techniques have been instrumental and GM damage was found to be clinically highly relevant. GM lesions and GM atrophy correlate with clinical disability and cognitive dysfunction. The clinical importance of GM pathology in MS notwithstanding, the mechanisms underlying GM pathology so far remain poorly understood. The next decade of MS research will deliver crucial new insights in this field and will hopefully result in a better understanding of what causes GM pathology in MS.
Abbreviations: | ||
MS | = | multiple sclerosis |
RRMS | = | relapsing-remitting MS |
SPMS | = | secondary progressive MS |
PPMS | = | primary progressive MS |
GM | = | grey matter |
WM | = | white matter |
LFB | = | luxal fast blue |
PLP | = | proteolipid protein |
MBP | = | myelin basic protein |
CIS | = | clinically isolated syndrome |
MRI | = | magnetic resonance imaging |
FLAIR | = | fluid-attenuated inversion recovery |
MTR | = | magnetic transfer imaging |
DIR | = | double inversion recovery |
PSIR | = | phase sensitive inversion recovery |
RAM | = | rims of activated microglia |
ATP | = | adenosine triphosphate |
AD | = | Alzheimer’s Disease |
ALS | = | amyotrophic lateral sclerosis |
NC | = | non-neurological control |
ChAT | = | choline acetyl transferase |
AChE | = | acetylcholinesterase |
Acknowledgments
The authors thank the Dutch MS Research Foundation for support via a program grant to the VUmc MS Centre Amsterdam (grant no. 09-358d).
Disclosure of Potential Conflicts of Interest
The authors declare no conflicts of interest
References
- Lublin FD, Reingold SC, National Multiple Sclerosis Society (USA) Advisory Committee on Clinical Trials of New Agents in Multiple Sclerosis. Defining the clinical course of multiple sclerosis: results of an international survey. Neurology 1996; 46:907 - 11; http://dx.doi.org/10.1212/WNL.46.4.907; PMID: 8780061
- Rovaris M, Confavreux C, Furlan R, Kappos L, Comi G, Filippi M. Secondary progressive multiple sclerosis: current knowledge and future challenges. Lancet Neurol 2006; 5:343 - 54; http://dx.doi.org/10.1016/S1474-4422(06)70410-0; PMID: 16545751
- Miller DH, Leary SM. Primary-progressive multiple sclerosis. Lancet Neurol 2007; 6:903 - 12; http://dx.doi.org/10.1016/S1474-4422(07)70243-0; PMID: 17884680
- Cottrell DA, Kremenchutzky M, Rice GP, Koopman WJ, Hader W, Baskerville J, et al. The natural history of multiple sclerosis: a geographically based study. 5. The clinical features and natural history of primary progressive multiple sclerosis. Brain 1999; 122:625 - 39; http://dx.doi.org/10.1093/brain/122.4.625; PMID: 10219776
- Bö L, Geurts JJG, van der Valk P, Polman C, Barkhof F. Lack of correlation between cortical demyelination and white matter pathologic changes in multiple sclerosis. Arch Neurol 2007; 64:76 - 80; http://dx.doi.org/10.1001/archneur.64.1.76; PMID: 17210812
- Brownell B, Hughes JT. The distribution of plaques in the cerebrum in multiple sclerosis. J Neurol Neurosurg Psychiatry 1962; 25:315 - 20; http://dx.doi.org/10.1136/jnnp.25.4.315; PMID: 14016083
- Bø L, Vedeler CA, Nyland HI, Trapp BD, Mørk SJ. Subpial demyelination in the cerebral cortex of multiple sclerosis patients. J Neuropathol Exp Neurol 2003; 62:723 - 32; PMID: 12901699
- Bø L, Vedeler CA, Nyland H, Trapp BD, Mørk SJ. Intracortical multiple sclerosis lesions are not associated with increased lymphocyte infiltration. Mult Scler 2003; 9:323 - 31; http://dx.doi.org/10.1191/1352458503ms917oa; PMID: 12926836
- Kutzelnigg A, Lucchinetti CF, Stadelmann C, Brück W, Rauschka H, Bergmann M, et al. Cortical demyelination and diffuse white matter injury in multiple sclerosis. Brain 2005; 128:2705 - 12; http://dx.doi.org/10.1093/brain/awh641; PMID: 16230320
- Dutta R, Chang A, Doud MK, Kidd GJ, Ribaudo MV, Young EA, et al. Demyelination causes synaptic alterations in hippocampi from multiple sclerosis patients. Ann Neurol 2011; 69:445 - 54; http://dx.doi.org/10.1002/ana.22337; PMID: 21446020
- Geurts JJG, Bö L, Roosendaal SD, Hazes T, Daniëls R, Barkhof F, et al. Extensive hippocampal demyelination in multiple sclerosis. J Neuropathol Exp Neurol 2007; 66:819 - 27; http://dx.doi.org/10.1097/nen.0b013e3181461f54; PMID: 17805012
- Gilmore CP, Bö L, Owens T, Lowe J, Esiri MM, Evangelou N. Spinal cord gray matter demyelination in multiple sclerosis-a novel pattern of residual plaque morphology. Brain Pathol 2006; 16:202 - 8; http://dx.doi.org/10.1111/j.1750-3639.2006.00018.x; PMID: 16911477
- Gilmore CP, Donaldson I, Bö L, Owens T, Lowe J, Evangelou N. Regional variations in the extent and pattern of grey matter demyelination in multiple sclerosis: a comparison between the cerebral cortex, cerebellar cortex, deep grey matter nuclei and the spinal cord. J Neurol Neurosurg Psychiatry 2009; 80:182 - 7; http://dx.doi.org/10.1136/jnnp.2008.148767; PMID: 18829630
- Huitinga I, De Groot CJ, Van der Valk P, Kamphorst W, Tilders FJ, Swaab DF. Hypothalamic lesions in multiple sclerosis. J Neuropathol Exp Neurol 2001; 60:1208 - 18; PMID: 11764093
- Kooi E-J, Prins M, Bajic N, Beliën JAM, Gerritsen WH, van Horssen J, et al. Cholinergic imbalance in the multiple sclerosis hippocampus. Acta Neuropathol 2011; 122:313 - 22; http://dx.doi.org/10.1007/s00401-011-0849-4; PMID: 21691765
- Kutzelnigg A, Faber-Rod JC, Bauer J, Lucchinetti CF, Sorensen PS, Laursen H, et al. Widespread demyelination in the cerebellar cortex in multiple sclerosis. Brain Pathol 2007; 17:38 - 44; http://dx.doi.org/10.1111/j.1750-3639.2006.00041.x; PMID: 17493036
- Papadopoulos D, Dukes S, Patel R, Nicholas R, Vora A, Reynolds R. Substantial archaeocortical atrophy and neuronal loss in multiple sclerosis. Brain Pathol 2009; 19:238 - 53; http://dx.doi.org/10.1111/j.1750-3639.2008.00177.x; PMID: 18492094
- Peterson JW, Bö L, Mörk S, Chang A, Trapp BD. Transected neurites, apoptotic neurons, and reduced inflammation in cortical multiple sclerosis lesions. Ann Neurol 2001; 50:389 - 400; http://dx.doi.org/10.1002/ana.1123; PMID: 11558796
- Vercellino M, Plano F, Votta B, Mutani R, Giordana MT, Cavalla P. Grey matter pathology in multiple sclerosis. J Neuropathol Exp Neurol 2005; 64:1101 - 7; http://dx.doi.org/10.1097/01.jnen.0000190067.20935.42; PMID: 16319720
- Wegner C, Esiri MM, Chance SA, Palace J, Matthews PM. Neocortical neuronal, synaptic, and glial loss in multiple sclerosis. Neurology 2006; 67:960 - 7; http://dx.doi.org/10.1212/01.wnl.0000237551.26858.39; PMID: 17000961
- Vercellino M, Masera S, Lorenzatti M, Condello C, Merola A, Mattioda A, et al. Demyelination, inflammation, and neurodegeneration in multiple sclerosis deep gray matter. J Neuropathol Exp Neurol 2009; 68:489 - 502; http://dx.doi.org/10.1097/NEN.0b013e3181a19a5a; PMID: 19525897
- Squire LR, Stark CEL, Clark RE. The medial temporal lobe. Annu Rev Neurosci 2004; 27:279 - 306; http://dx.doi.org/10.1146/annurev.neuro.27.070203.144130; PMID: 15217334
- Moscovitch M, Nadel L, Winocur G, Gilboa A, Rosenbaum RS. The cognitive neuroscience of remote episodic, semantic and spatial memory. Curr Opin Neurobiol 2006; 16:179 - 90; http://dx.doi.org/10.1016/j.conb.2006.03.013; PMID: 16564688
- Bakshi R, Ariyaratana S, Benedict RH, Jacobs L. Fluid-attenuated inversion recovery magnetic resonance imaging detects cortical and juxtacortical multiple sclerosis lesions. Arch Neurol 2001; 58:742 - 8; http://dx.doi.org/10.1001/archneur.58.5.742; PMID: 11346369
- Seewann A, Vrenken H, Kooi EJ, van der Valk P, Knol DL, Polman CH, et al. Imaging the tip of the iceberg: visualization of cortical lesions in multiple sclerosis. Mult Scler 2011; 17:1202 - 10; http://dx.doi.org/10.1177/1352458511406575; PMID: 21561955
- Geurts JJG, Pouwels PJW, Uitdehaag BMJ, Polman CH, Barkhof F, Castelijns JA. Intracortical lesions in multiple sclerosis: improved detection with 3D double inversion-recovery MR imaging. Radiology 2005; 236:254 - 60; http://dx.doi.org/10.1148/radiol.2361040450; PMID: 15987979
- Seewann A, Kooi E-J, Roosendaal SD, Pouwels PJW, Wattjes MP, van der Valk P, et al. Postmortem verification of MS cortical lesion detection with 3D DIR. Neurology 2012; 78:302 - 8; http://dx.doi.org/10.1212/WNL.0b013e31824528a0; PMID: 22218278
- Sethi V, Yousry TA, Muhlert N, Ron M, Golay X, Wheeler-Kingshott C, et al. Improved detection of cortical MS lesions with phase-sensitive inversion recovery MRI. J Neurol Neurosurg Psychiatry 2012; 83:877 - 82; http://dx.doi.org/10.1136/jnnp-2012-303023; PMID: 22807559
- Nelson F, Poonawalla A, Hou P, Wolinsky JS, Narayana PA. 3D MPRAGE improves classification of cortical lesions in multiple sclerosis. Mult Scler 2008; 14:1214 - 9; http://dx.doi.org/10.1177/1352458508094644; PMID: 18952832
- Nielsen AS, Kinkel RP, Tinelli E, Benner T, Cohen-Adad J, Mainero C. Focal cortical lesion detection in multiple sclerosis: 3 Tesla DIR versus 7 Tesla FLASH-T2. J Magn Reson Imaging 2012; 35:537 - 42; http://dx.doi.org/10.1002/jmri.22847; PMID: 22045554
- de Graaf WL, Kilsdonk ID, Lopez-Soriano A, Zwanenburg JJM, Visser F, Polman CH, et al. Clinical application of multi-contrast 7-T MR imaging in multiple sclerosis: increased lesion detection compared to 3 T confined to grey matter. Eur Radiol 2012; PMID: 22898935
- Audoin B, Zaaraoui W, Reuter F, Rico A, Malikova I, Confort-Gouny S, et al. Atrophy mainly affects the limbic system and the deep grey matter at the first stage of multiple sclerosis. J Neurol Neurosurg Psychiatry 2010; 81:690 - 5; http://dx.doi.org/10.1136/jnnp.2009.188748; PMID: 20392976
- Batista S, Zivadinov R, Hoogs M, Bergsland N, Heininen-Brown M, Dwyer MG, et al. Basal ganglia, thalamus and neocortical atrophy predicting slowed cognitive processing in multiple sclerosis. J Neurol 2012; 259:139 - 46; http://dx.doi.org/10.1007/s00415-011-6147-1; PMID: 21720932
- Calabrese M, Rinaldi F, Mattisi I, Bernardi V, Favaretto A, Perini P, et al. The predictive value of gray matter atrophy in clinically isolated syndromes. Neurology 2011; 77:257 - 63; http://dx.doi.org/10.1212/WNL.0b013e318220abd4; PMID: 21613600
- Roosendaal SD, Bendfeldt K, Vrenken H, Polman CH, Borgwardt S, Radue EW, et al. Grey matter volume in a large cohort of MS patients: relation to MRI parameters and disability. Mult Scler 2011; 17:1098 - 106; http://dx.doi.org/10.1177/1352458511404916; PMID: 21586487
- Calabrese M, De Stefano N, Atzori M, Bernardi V, Mattisi I, Barachino L, et al. Detection of cortical inflammatory lesions by double inversion recovery magnetic resonance imaging in patients with multiple sclerosis. Arch Neurol 2007; 64:1416 - 22; http://dx.doi.org/10.1001/archneur.64.10.1416; PMID: 17923625
- Calabrese M, Filippi M, Rovaris M, Bernardi V, Atzori M, Mattisi I, et al. Evidence for relative cortical sparing in benign multiple sclerosis: a longitudinal magnetic resonance imaging study. Mult Scler 2009; 15:36 - 41; http://dx.doi.org/10.1177/1352458508096686; PMID: 18755823
- Calabrese M, Gallo P. Magnetic resonance evidence of cortical onset of multiple sclerosis. Mult Scler 2009; 15:933 - 41; http://dx.doi.org/10.1177/1352458509106510; PMID: 19667021
- Calabrese M, Rocca MA, Atzori M, Mattisi I, Favaretto A, Perini P, et al. A 3-year magnetic resonance imaging study of cortical lesions in relapse-onset multiple sclerosis. Ann Neurol 2010; 67:376 - 83; PMID: 20373349
- Cohen-Adad J, Benner T, Greve D, Kinkel RP, Radding A, Fischl B, et al. In vivo evidence of disseminated subpial T2* signal changes in multiple sclerosis at 7 T: a surface-based analysis. Neuroimage 2011; 57:55 - 62; http://dx.doi.org/10.1016/j.neuroimage.2011.04.009; PMID: 21511042
- Calabrese M, Agosta F, Rinaldi F, Mattisi I, Grossi P, Favaretto A, et al. Cortical lesions and atrophy associated with cognitive impairment in relapsing-remitting multiple sclerosis. Arch Neurol 2009; 66:1144 - 50; http://dx.doi.org/10.1001/archneurol.2009.174; PMID: 19752305
- Roosendaal SD, Moraal B, Pouwels PJ, Vrenken H, Castelijns JA, Barkhof F, et al. Accumulation of cortical lesions in MS: relation with cognitive impairment. Mult Scler 2009; 15:708 - 14; http://dx.doi.org/10.1177/1352458509102907; PMID: 19435749
- Roosendaal SD, Moraal B, Vrenken H, Castelijns JA, Pouwels PJW, Barkhof F, et al. In vivo MR imaging of hippocampal lesions in multiple sclerosis. J Magn Reson Imaging 2008; 27:726 - 31; http://dx.doi.org/10.1002/jmri.21294; PMID: 18302199
- Geurts JJG, Bö L, Pouwels PJW, Castelijns JA, Polman CH, Barkhof F. Cortical lesions in multiple sclerosis: combined postmortem MR imaging and histopathology. AJNR Am J Neuroradiol 2005; 26:572 - 7; PMID: 15760868
- Nakamura K, Fisher E. Segmentation of brain magnetic resonance images for measurement of gray matter atrophy in multiple sclerosis patients. Neuroimage 2009; 44:769 - 76; http://dx.doi.org/10.1016/j.neuroimage.2008.09.059; PMID: 19007895
- Fisher E, Lee J-C, Nakamura K, Rudick RA. Gray matter atrophy in multiple sclerosis: a longitudinal study. Ann Neurol 2008; 64:255 - 65; http://dx.doi.org/10.1002/ana.21436; PMID: 18661561
- Fisniku LK, Chard DT, Jackson JS, Anderson VM, Altmann DR, Miszkiel KA, et al. Gray matter atrophy is related to long-term disability in multiple sclerosis. Ann Neurol 2008; 64:247 - 54; http://dx.doi.org/10.1002/ana.21423; PMID: 18570297
- Brink BP, Veerhuis R, Breij ECW, van der Valk P, Dijkstra CD, Bö L. The pathology of multiple sclerosis is location-dependent: no significant complement activation is detected in purely cortical lesions. J Neuropathol Exp Neurol 2005; 64:147 - 55; PMID: 15751229
- van Horssen J, Brink BP, de Vries HE, van der Valk P, Bø L. The blood-brain barrier in cortical multiple sclerosis lesions. J Neuropathol Exp Neurol 2007; 66:321 - 8; http://dx.doi.org/10.1097/nen.0b013e318040b2de; PMID: 17413323
- Lucchinetti CF, Popescu BFG, Bunyan RF, Moll NM, Roemer SF, Lassmann H, et al. Inflammatory cortical demyelination in early multiple sclerosis. N Engl J Med 2011; 365:2188 - 97; http://dx.doi.org/10.1056/NEJMoa1100648; PMID: 22150037
- Kooi EJ, Strijbis EM, van der Valk P, Geurts JJ. Heterogeneity of cortical lesions in multiple sclerosis: clinical and pathologic implications. Neurology 2012; 79:1369 - 76; http://dx.doi.org/10.1212/WNL.0b013e31826c1b1c; PMID: 22972651
- Politis M, Giannetti P, Su P, Turkheimer F, Keihaninejad S, Wu K, et al. Increased PK11195 PET binding in the cortex of patients with MS correlates with disability. Neurology 2012; 79:523 - 30; http://dx.doi.org/10.1212/WNL.0b013e3182635645; PMID: 22764258
- Nimmerjahn A, Kirchhoff F, Helmchen F. Resting microglial cells are highly dynamic surveillants of brain parenchyma in vivo. Science 2005; 308:1314 - 8; http://dx.doi.org/10.1126/science.1110647; PMID: 15831717
- Davalos D, Grutzendler J, Yang G, Kim JV, Zuo Y, Jung S, et al. ATP mediates rapid microglial response to local brain injury in vivo. Nat Neurosci 2005; 8:752 - 8; http://dx.doi.org/10.1038/nn1472; PMID: 15895084
- Melton LM, Keith AB, Davis S, Oakley AE, Edwardson JA, Morris CM. Chronic glial activation, neurodegeneration, and APP immunoreactive deposits following acute administration of double-stranded RNA. Glia 2003; 44:1 - 12; http://dx.doi.org/10.1002/glia.10276; PMID: 12951652
- Nakanishi H. Microglial functions and proteases. Mol Neurobiol 2003; 27:163 - 76; http://dx.doi.org/10.1385/MN:27:2:163; PMID: 12777686
- Taylor DL, Jones F, Kubota ESFCS, Pocock JM. Stimulation of microglial metabotropic glutamate receptor mGlu2 triggers tumor necrosis factor α-induced neurotoxicity in concert with microglial-derived Fas ligand. J Neurosci 2005; 25:2952 - 64; http://dx.doi.org/10.1523/JNEUROSCI.4456-04.2005; PMID: 15772355
- Noseworthy JH, Lucchinetti C, Rodriguez M, Weinshenker BG. Multiple sclerosis. N Engl J Med 2000; 343:938 - 52; http://dx.doi.org/10.1056/NEJM200009283431307; PMID: 11006371
- Magliozzi R, Howell O, Vora A, Serafini B, Nicholas R, Puopolo M, et al. Meningeal B-cell follicles in secondary progressive multiple sclerosis associate with early onset of disease and severe cortical pathology. Brain 2007; 130:1089 - 104; http://dx.doi.org/10.1093/brain/awm038; PMID: 17438020
- Torkildsen Ø, Stansberg C, Angelskår SM, Kooi E-J, Geurts JJG, van der Valk P, et al. Upregulation of immunoglobulin-related genes in cortical sections from multiple sclerosis patients. Brain Pathol 2010; 20:720 - 9; http://dx.doi.org/10.1111/j.1750-3639.2009.00343.x; PMID: 19919606
- Willis SN, Stadelmann C, Rodig SJ, Caron T, Gattenloehner S, Mallozzi SS, et al. Epstein-Barr virus infection is not a characteristic feature of multiple sclerosis brain. Brain 2009; 132:3318 - 28; http://dx.doi.org/10.1093/brain/awp200; PMID: 19638446
- Magliozzi R, Howell OW, Reeves C, Roncaroli F, Nicholas R, Serafini B, et al. A Gradient of neuronal loss and meningeal inflammation in multiple sclerosis. Ann Neurol 2010; 68:477 - 93; http://dx.doi.org/10.1002/ana.22230; PMID: 20976767
- Kooi E-J, Geurts JJG, van Horssen J, Bø L, van der Valk P. Meningeal inflammation is not associated with cortical demyelination in chronic multiple sclerosis. J Neuropathol Exp Neurol 2009; 68:1021 - 8; http://dx.doi.org/10.1097/NEN.0b013e3181b4bf8f; PMID: 19680141
- Craner MJ, Newcombe J, Black JA, Hartle C, Cuzner ML, Waxman SG. Molecular changes in neurons in multiple sclerosis: altered axonal expression of Nav1.2 and Nav1.6 sodium channels and Na+/Ca2+ exchanger. Proc Natl Acad Sci U S A 2004; 101:8168 - 73; http://dx.doi.org/10.1073/pnas.0402765101; PMID: 15148385
- Dutta R, McDonough J, Yin X, Peterson J, Chang A, Torres T, et al. Mitochondrial dysfunction as a cause of axonal degeneration in multiple sclerosis patients. Ann Neurol 2006; 59:478 - 89; http://dx.doi.org/10.1002/ana.20736; PMID: 16392116
- Lu F, Selak M, O’Connor J, Croul S, Lorenzana C, Butunoi C, et al. Oxidative damage to mitochondrial DNA and activity of mitochondrial enzymes in chronic active lesions of multiple sclerosis. J Neurol Sci 2000; 177:95 - 103; http://dx.doi.org/10.1016/S0022-510X(00)00343-9; PMID: 10980305
- Lin MT, Beal MF. Mitochondrial dysfunction and oxidative stress in neurodegenerative diseases. Nature 2006; 443:787 - 95; http://dx.doi.org/10.1038/nature05292; PMID: 17051205
- Stys PK. Axonal degeneration in multiple sclerosis: is it time for neuroprotective strategies?. Ann Neurol 2004; 55:601 - 3; http://dx.doi.org/10.1002/ana.20082; PMID: 15122698
- Trapp BD, Stys PK. Virtual hypoxia and chronic necrosis of demyelinated axons in multiple sclerosis. Lancet Neurol 2009; 8:280 - 91; http://dx.doi.org/10.1016/S1474-4422(09)70043-2; PMID: 19233038
- Geurts JJG, Wolswijk G, Bö L, van der Valk P, Polman CH, Troost D, et al. Altered expression patterns of group I and II metabotropic glutamate receptors in multiple sclerosis. Brain 2003; 126:1755 - 66; http://dx.doi.org/10.1093/brain/awg179; PMID: 12805104
- Geurts JJG, Wolswijk G, Bö L, Redeker S, Ramkema M, Troost D, et al. Expression patterns of Group III metabotropic glutamate receptors mGluR4 and mGluR8 in multiple sclerosis lesions. J Neuroimmunol 2005; 158:182 - 90; http://dx.doi.org/10.1016/j.jneuroim.2004.08.012; PMID: 15589052
- Newcombe J, Uddin A, Dove R, Patel B, Turski L, Nishizawa Y, et al. Glutamate receptor expression in multiple sclerosis lesions. Brain Pathol 2008; 18:52 - 61; http://dx.doi.org/10.1111/j.1750-3639.2007.00101.x; PMID: 17924980
- Schmierer K, Parkes HG, So P-W, An SF, Brandner S, Ordidge RJ, et al. High field (9.4 Tesla) magnetic resonance imaging of cortical grey matter lesions in multiple sclerosis. Brain 2010; 133:858 - 67; http://dx.doi.org/10.1093/brain/awp335; PMID: 20123726