Abstract
Single nucleotide polymorphisms in the ubiquilin-1 gene may confer risk for late-onset Alzheimer disease (AD). We have shown previously that ubiquilin-1 functions as a molecular chaperone for the amyloid precursor protein (APP) and that protein levels of ubiquilin-1 are decreased in the brains of AD patients. We have recently found that ubiquilin-1 regulates APP trafficking and subsequent secretase processing by stimulating non-degradative ubiquitination of a single lysine residue in the cytosolic domain of APP. Thus, ubiquilin-1 plays a central role in regulating APP biosynthesis, trafficking and ultimately toxicity. As ubiquilin-1 and other ubiquilin family members have now been implicated in the pathogenesis of numerous neurodegenerative diseases, these findings provide mechanistic insights into the central role of ubiquilin proteins in maintaining neuronal proteostasis.
Introduction
Alzheimer disease (AD) is the most common cause of progressive age-associated dementia. AD is characterized neuropathologically by the presence of amyloid plaques composed of an aggregated peptide termed Aβ. The discovery of Aβ and the gene encoding its precursor, amyloid precursor protein (APP), formed the basis of the amyloid cascade hypothesis, which postulates that Aβ production and aggregation are critical events in the pathogenesis of AD. An extensive body of research offers strong evidence that APP and its proteolytic products are central to AD pathogenesis, particularly in rare, dominantly inherited forms of the disease. Thus, determining the molecular mechanisms that regulate APP trafficking, degradation, processing and subsequent Aβ generation are critical to understanding the mechanisms of synaptic dysfunction and neuronal loss in AD.
Genetics of AD and Potential Contribution of Ubiquilin-1
Our current understanding of the pathogenesis of AD is largely based on the study of rare early-onset dominantly inherited forms of the disease, collectively known as familial AD (FAD). FAD is caused by alterations in the proteolytic processing of APP. APP can be cleaved by secretase enzymes generating proteolytic fragments of various lengths. Amyloidogenic processing of APP occurs via successive proteolysis by β-secretase and γ-secretase to produce a large secreted ectodomain fragment, the Aβ peptide and an intracellular domain that is released into the cytosol (AICD, APP intracellular domain). FAD is caused by mutations in the APP molecule or the presenilin proteins, which constitute the catalytic core of γ-secretase, all of which promote the formation of a particularly amyloidogenic version of the Aβ peptide (termed Aβ42). FAD cases account for less than ten percent of all AD cases, and little is known about the causes of sporadic late-onset AD. So far, inheritance of the ε4 allele of the APOE gene is the only established genetic risk factor for developing late-onset ADCitation1,Citation2 and thus there has been intense interest in identifying additional genetic loci associated with late forms of the disease. A positional candidate gene approach reported that single nucleotide polymorphisms in the UBQLN1 gene have a family-based association with late-onset AD.Citation3 It was suggested that polymorphisms in the UBQLN1 gene lead to changes in alternative splicing. However, the exact molecular mechanism by which the protein product of this gene, ubiquilin-1, contributes to disease pathogenesis remains to this day unclear. Furthermore, several other studies have failed to identify a genetic linkage of UBQLN1 with AD in other patient populations.Citation4-Citation8 Regardless, we have recently demonstrated that AD was associated with decreased ubiquilin-1 protein levels irrespective of UBQLN1 genotype,Citation9 suggesting that a more complex combination of genetic and environmental factors may ultimately lead to alterations in ubiquilin-1 function.
Ubiquilin-1 Structure
Ubiquilin-1 is a member of a superfamily of proteins containing an N-terminal ubiquitin-like domain (UBL) and a C-terminal ubiquitin-associated domain (UBA). Both domains have been implicated in targeting proteins for degradation by the proteasome.Citation10,Citation11 The UBL domain directly interacts with the S5a/Rpn10/p54 subunit of the proteasome,Citation10 while the UBA domain binds mono and polyubiquitinated substrates. The central region of ubiquilin contains Sti1 motifs implicated in protein-protein interactions,Citation12 and these motifs have been shown to possess molecular chaperone activity.Citation13 We have recently shown that ubiquilin displays molecular chaperone function toward the model clients citrate synthase and firefly luciferase and, more relevantly, toward APP.Citation9 Furthermore, we found that the Sti1 domains of ubiquilin-1 bind APP early in the secretory pathway and prevent inappropriate intermolecular interactions of the AICD, demonstrating the ability of ubiquilin-1 to prevent the aggregation of AICD/APP in in vitro and in cellular models.Citation9
Protein Ubiquitination and Regulation by UBL/UBA Domain-Containing Proteins
Ubiquitin is a 76 amino acid protein which is covalently attached to the ε-amino group of lysine residues on target proteins via an isopeptide bond. Ubiquitin can be conjugated as a single molecule. Alternatively, additional ubiquitin molecules can be further conjugated to one of several lysine residues present on the previously attached ubiquitin moiety itself to make a polyubiquitin chain. Polyubiquitin chains conjugated to lysine 29 (K29) or lysine 48 (K48) of ubiquitin constitute a degradative signal, whereas monoubiquitin and chains conjugated to lysine 63 (K63) function in a signaling capacity.Citation14 These reactions are catalyzed by ubiquitin E2 and E3 ligases, with the latter important in determining substrate specificity. RING finger E3 ligases function as scaffolds to bring E2 enzymes in close proximity to substrates and are representative of the majority of E3s in the human genome.Citation15 Once a polypeptide chain is modified by a ubiquitin molecule, it has the potential to be bound by a UBA domain-containing protein.
UBA domains can bind to a variety of ubiquitin modifications, including K48/K63 and monoubiquitin.Citation16 The UBA domains of ubiquilin-1 and its fungal homolog Dsk2 are quite promiscuous and can bind mono, K48 and K63 chains with equivalent affinities.Citation17,Citation18 The functions of UBA domains are quite diverse, but are best characterized for the DNA repair protein and ubiquilin homolog hRad23. K48 ubiquitination of hRad23 substrates results in hRad23 binding via the UBA domain. The substrate is subsequently recruited to the S5a subunit of the proteasome via the UBL domain of hRad23. Thus, hRad23 is thought to function as a “shuttle” that delivers ubiquitinated substrates to the proteasome.Citation19 Counter intuitively, hRad23 has also been shown to stabilize substrates and allow accumulation of polyubiquitinated proteins.Citation20 This finding has now been demonstrated for numerous other UBA domain containing proteins. This mechanism may be related to the ability of UBA domains to “cap” small chain ubiquitinated substrates preventing further chain elongation.Citation16 There is strong evidence that ubiquilin-1 also stabilizes ubiquitinated substrates, preventing proteasomal degradation,Citation21-Citation24 but the significance of this finding is not entirely clear. It is also possible that increased ubiquitination of substrates by UBL/UBA domain proteins is not due to stabilization of ubiquitinated species, but rather to an active recruitment of E3 ligases to substrates, as is the case for the ubiquilin homolog KPC2.Citation25
Ubiquilin-1 is a Regulator of Membrane Protein Trafficking
Although ubiquitination is a well-established regulator of endocytosis and protein trafficking from the Golgi apparatus,Citation26 very little is known about the role of UBA/UBL domain proteins in this process. One prominent exception is ubiquilin-1, which regulates the trafficking of multiple transmembrane proteins. Ubiquilin-1 increases polyubiquitinated species of GABA receptors, and, similar to other UBA/UBL substrates, stabilizes them.Citation24 Interestingly, GABA receptor stabilization occurs in early secretory compartments, increasing their availability for rapid movement to the plasma membrane as a result of neuronal activity. Ubiquilin also negatively regulates stimulus-dependent G protein coupled receptor endocytosis.Citation27 This effect is mediated by the UBL domain binding to the cargo binding proteins epsins 1,2 and Eps15 of the clathrin-mediated endocytosis pathway,Citation27 but the role of receptor ubiquitination has not been investigated. Ubiquilin-1 has also been shown to associate with multiple E3 ligases to promote ubiquitination and stabilization of substrates, including E6AP and βTRCP.Citation23 Significant questions remain regarding these studies, including (1) why ubiquilin-1 stimulated ubiquitination of substrates results in substrate stabilization, (2) the precise nature of the polyubiquitin chains and (3) how ubiquilin is regulating trafficking to different subcellular compartments in mechanistic detail.
Ubiquilin-1 Regulates APP Trafficking Through Non-Degradative Ubiquitination
APP maturation is known to be regulated by ubiquilin-1.Citation28,Citation29 APP matures through the secretory pathway, where it undergoes classical N- and O-linked glycosylation and homodimerization as it transits through the endoplasmic reticulum and Golgi apparatus.Citation30-Citation32 After transport to the plasma membrane, APP can be recycled through the endocytic pathway.Citation33 In some cell types, up to 70% of APP is degraded by lysosomes, although it is unclear if degradation first requires transport to the plasma membrane.Citation30,Citation34,Citation35 Regardless, APP half-life is very short (less than one hour) and the fraction of total APP protein present at the plasma membrane is minimal.Citation34,Citation36 Almost nothing is known about the proteasomal degradation of APP. However, ubiquitination of APP may be partially mediated by the bifunctional chaperone/E3 ubiquitin ligase CHIP.Citation37
Various proteins regulate the trafficking of APP to different subcellular compartments and thus regulate proteolytic processing. SorLA or LR11 acts as a sorting receptor that sequesters APP in the Golgi apparatus and reduces secretase processing of APP by limiting access to the secretase.Citation38 Numerous other proteins bind to the AICD to regulate vesicular trafficking and endocytosis of APP, notably X11α/Fe65.Citation33,Citation36,Citation39
Ubiquilin-1 knockdown accelerates APP maturation and increases amyloidogenic processing.Citation29 Our group and others have shown that ubiquilin-1 binds to APP.Citation29,Citation34 As stated above, ubiquilin-1 binds to APP and exerts chaperone activity on the molecule, most likely via its Sti1 domains.Citation9 More recently, we have shown that ubiquilin-1 inhibits the exit of APP from the Golgi apparatus in a very similar fashion to SorLA.Citation38 Expression of ubiquilin-1 stimulates K63-linked polyubiquitination of lysine 688 (K688) in the AICD. K688 polyubiquitination results in decreased transport of APP to the plasma membrane, diminished amyloidogenic processing and reduced cell death.Citation9,Citation34 Importantly, mutation of K688 to arginine in the AICD abrogated ubiquilin-1 dependent ubiquitination and Golgi sequestration,Citation34 providing strong evidence that ubiquitination of a single lysine residue in the AICD of APP is necessary for the observed effects on APP trafficking. Presumably, ubiquilin-1 recruits a yet unidentified E3 ligase to APP. Candidates include E3 ligases known to bind ubiquilin-1, such as HRD1,Citation40 E6APCitation23 and βTRCP.Citation23 Importantly, how K63-linked ubiquitination of APP results in decreased trafficking out of the Golgi apparatus is not readily apparent. One mechanism by which secretory proteins exit the Golgi apparatus is by binding to adaptor proteins that couple cargo to the vesicular transport machinery. It has recently been shown for other substrates that K63-linked polyubiquitin chains are a sorting signal from the Golgi.Citation41 The adaptor protein optineurin is a cargo adaptorCitation42,Citation43 that binds K63-linked polyubiquitin chains,Citation44 and thus constitutes an attractive target for further investigation. K688 is positioned C-terminally only one amino acid away from the critical YENPTY sequence (single amino acid code) known to mediate APP sorting and endocytosis by binding to proteins such as X11α/Fe65.Citation45-Citation48 Thus, it is possible that polyubiquitination of K688 results in a steric barrier regulating the binding of these proteins critical for APP trafficking.
Ubiquilin-1 as a Regulator of Protein Degradation
Ubiquilin-1 is involved in the regulation of other quality control pathways such as the ER-associated degradation (ERAD) pathway and autophagy. Ubiquilin-1 forms a complex with the ERAD protein ERASIN and the molecular chaperone p97/VCP to help clear misfolded proteins from the ER and deliver them to the proteasome.Citation49 Ubiquilin-1 also interacts with the ER stress protein Herp to enhance ER-associated degradation of certain substrates.Citation40 APP is synthesized and N-glycosylated in the ER, and misfolded APP may be degraded through the ERAD system before maturation through the Golgi apparatus and transport to the cell surface. Furthermore, APP has been shown to interact with the ER luminal chaperone BiP/ GRP78 and the stress-responsive chaperone/protease HtrA2/Omi.Citation50 A subpopulation of HtrA2 localizes to the cytosolic side of the ER membrane where it contributes to ERAD-mediated degradation of APP.Citation50 It is thus logical that ubiquilin-1 may contribute to ERAD of immature APP, however our overexpression studies indicates that ubiquilin-1 stabilizes this form of APP.Citation34 Future studies will be needed to elucidate the effects of ubiquilin-1 on ERAD of APP. In addition to ERAD, ubiquilin-1 has an established role in the regulation of aggresome formation,Citation51 targeting presenilins to aggresomes,Citation52 is co-localized with various autophagy markers,Citation53,Citation54 and is itself degraded by chaperone-mediated autophagy.Citation55,Citation56 Thus, ubiquilin-1 has a central role in regulating protein trafficking, accumulation and degradation of diverse proteins relevant to neurodegenerative disease.
A Model for Ubiquilin-1 as a Critical Regulator of APP Proteostasis
Our current model for ubiquilin-1 function is depicted in . We postulate that first ubiquilin-1 binds to the AICD via the Sti-1 domain(s). This stabilizes an open conformation of ubiquilin-1 that results in an interaction of moderate affinity allowing binding and release events (consistent with a chaperone/client interaction). This prevents aggregation of APPCitation28 but allows subsequent maturation events. Next, ubiquilin-1 promotes ubiquitination of AICD, possibly by directly recruiting an E3 ligase. Ubiquilin-1 now binds to AICD via both the Sti1 domain(s) and UBA domains. This result in a high affinity interaction, which prevents the interaction of APP with the Golgi export machinery,Citation32,Citation57,Citation58 thus sequestering it in this part of the secretory pathway. Finally, conformational maturation (acquisition of appropriate tertiary and quaternary structure of APP, e.g., dimer formation) results in displacement of Sti-1 domains, thus resulting in a weakening of the interaction and eventual release. This could be a simple result of two APP protomers coming together and sterically hindering the Sti1 binding site and/or a conformational change on the protomer. Importantly, we have shown that ubiquilin-1 expression inhibits secretase/proteasomal degradation of APP without affecting lysosomal degradation.Citation34 Thus, ubiquilin-1 expression would function to increase net proteolysis of APP by lysosomal pathways. The end result is increased APP quality control, enhanced APP retention in the Golgi apparatus, increased lysosomal degradation and ultimately decreased secretase processing.
Figure 1. Schematic overview of the potential role of ubiquilin-1 in regulating APP maturation and trafficking. Ubiquilin-1 binds to APP via the Sti1 domains and possibly recruits an E3 ligase. Ubiquitination greatly increases the affinity of ubiquilin-1 through interactions with the UBA domain. Maturation of APP decreases the affinity of ubiquilin-1 for APP leading to eventual release. See text for details.
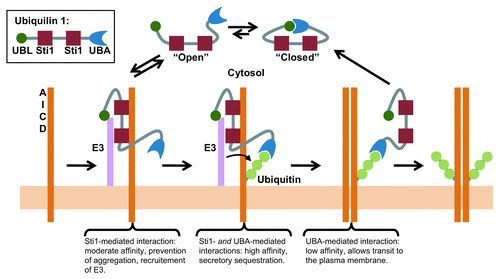
Conclusions
Our results suggest that ubiquilin-1 is a critical component of a trafficking checkpoint within the secretory pathway that prevents excessive amyloidogenic processing of APP by limiting access to secretase enzymes.Citation34 Consistent with this notion, we have shown that ubiquilin-1 overexpression decreases the Aβ42/40 ratio and prevents APP-induced toxicity in cells, while ubiquilin-1 knockdown exacerbates APP-induced toxicity.Citation28 A similar scenario may happen in AD patients, as ubiquilin-1 protein levels are inversely correlated with the Braak staging of the disease,Citation28 suggesting that therapies which restore ubiquilin-1 expression in the brain may represent a potential treatment for AD.
Acknowledgments
This work was supported by NIH grants R21AG031948 (J.M.B. and D.B.) and F30AG030878 (E.S.S.) and funds provided by the Jean C. and William D. Willis Neuroscience Research Endowment (E.S.S.).
Disclosure of Potential Conflicts of Interest
No potential conflicts of interest were disclosed.
References
- Strittmatter WJ, Saunders AM, Schmechel D, Pericak-Vance M, Enghild J, Salvesen GS, et al. Apolipoprotein E: high-avidity binding to beta-amyloid and increased frequency of type 4 allele in late-onset familial Alzheimer disease. Proc Natl Acad Sci U S A 1993; 90:1977 - 81; http://dx.doi.org/10.1073/pnas.90.5.1977; PMID: 8446617
- Farrer LA, Cupples LA, Haines JL, Hyman B, Kukull WA, Mayeux R, et al, APOE and Alzheimer Disease Meta Analysis Consortium. Effects of age, sex, and ethnicity on the association between apolipoprotein E genotype and Alzheimer disease. A meta-analysis. JAMA 1997; 278:1349 - 56; http://dx.doi.org/10.1001/jama.1997.03550160069041; PMID: 9343467
- Bertram L, Hiltunen M, Parkinson M, Ingelsson M, Lange C, Ramasamy K, et al. Family-based association between Alzheimer’s disease and variants in UBQLN1. N Engl J Med 2005; 352:884 - 94; http://dx.doi.org/10.1056/NEJMoa042765; PMID: 15745979
- Bensemain F, Chapuis J, Tian J, Shi J, Thaker U, Lendon C, et al. Association study of the Ubiquilin gene with Alzheimer’s disease. Neurobiol Dis 2006; 22:691 - 3; http://dx.doi.org/10.1016/j.nbd.2006.01.007; PMID: 16504527
- Brouwers N, Sleegers K, Engelborghs S, Bogaerts V, van Duijn CM, De Deyn PP, et al. The UBQLN1 polymorphism, UBQ-8i, at 9q22 is not associated with Alzheimer’s disease with onset before 70 years. Neurosci Lett 2006; 392:72 - 4; http://dx.doi.org/10.1016/j.neulet.2005.08.064; PMID: 16214290
- Slifer MA, Martin ER, Bronson PG, Browning-Large C, Doraiswamy PM, Welsh-Bohmer KA, et al. Lack of association between UBQLN1 and Alzheimer disease. Am J Med Genet B Neuropsychiatr Genet 2006; 141B:208 - 13; http://dx.doi.org/10.1002/ajmg.b.30298; PMID: 16526030
- Smemo S, Nowotny P, Hinrichs AL, Kauwe JS, Cherny S, Erickson K, et al. Ubiquilin 1 polymorphisms are not associated with late-onset Alzheimer’s disease. Ann Neurol 2006; 59:21 - 6; http://dx.doi.org/10.1002/ana.20673; PMID: 16278862
- Arias-Vásquez A, de Lau L, Pardo L, Liu F, Feng BJ, Bertoli-Avella A, et al. Relationship of the Ubiquilin 1 gene with Alzheimer’s and Parkinson’s disease and cognitive function. Neurosci Lett 2007; 424:1 - 5; http://dx.doi.org/10.1016/j.neulet.2007.07.015; PMID: 17709205
- Stieren ES, El Ayadi A, Xiao Y, Siller E, Landsverk ML, Oberhauser AF, et al. Ubiquilin-1 is a molecular chaperone for the amyloid precursor protein. J Biol Chem 2011; 286:35689 - 98; http://dx.doi.org/10.1074/jbc.M111.243147; PMID: 21852239
- Walters KJ, Kleijnen MF, Goh AM, Wagner G, Howley PM. Structural studies of the interaction between ubiquitin family proteins and proteasome subunit S5a. Biochemistry 2002; 41:1767 - 77; http://dx.doi.org/10.1021/bi011892y; PMID: 11827521
- Ko HS, Uehara T, Tsuruma K, Nomura Y. Ubiquilin interacts with ubiquitylated proteins and proteasome through its ubiquitin-associated and ubiquitin-like domains. FEBS Lett 2004; 566:110 - 4; http://dx.doi.org/10.1016/j.febslet.2004.04.031; PMID: 15147878
- Ficklin MB, Zhao S, Feng G. Ubiquilin-1 regulates nicotine-induced up-regulation of neuronal nicotinic acetylcholine receptors. J Biol Chem 2005; 280:34088 - 95; http://dx.doi.org/10.1074/jbc.M506781200; PMID: 16091357
- Zhao G, Zhou X, Wang L, Li G, Kisker C, Lennarz WJ, et al. Structure of the mouse peptide N-glycanase-HR23 complex suggests co-evolution of the endoplasmic reticulum-associated degradation and DNA repair pathways. J Biol Chem 2006; 281:13751 - 61; http://dx.doi.org/10.1074/jbc.M600137200; PMID: 16500903
- Mukhopadhyay D, Riezman H. Proteasome-independent functions of ubiquitin in endocytosis and signaling. Science 2007; 315:201 - 5; http://dx.doi.org/10.1126/science.1127085; PMID: 17218518
- Deshaies RJ, Joazeiro CA. RING domain E3 ubiquitin ligases. Annu Rev Biochem 2009; 78:399 - 434; http://dx.doi.org/10.1146/annurev.biochem.78.101807.093809; PMID: 19489725
- Su V, Lau AF. Ubiquitin-like and ubiquitin-associated domain proteins: significance in proteasomal degradation. Cell Mol Life Sci 2009; 66:2819 - 33; http://dx.doi.org/10.1007/s00018-009-0048-9; PMID: 19468686
- Zhang D, Raasi S, Fushman D. Affinity makes the difference: nonselective interaction of the UBA domain of Ubiquilin-1 with monomeric ubiquitin and polyubiquitin chains. J Mol Biol 2008; 377:162 - 80; http://dx.doi.org/10.1016/j.jmb.2007.12.029; PMID: 18241885
- Raasi S, Varadan R, Fushman D, Pickart CM. Diverse polyubiquitin interaction properties of ubiquitin-associated domains. Nat Struct Mol Biol 2005; 12:708 - 14; http://dx.doi.org/10.1038/nsmb962; PMID: 16007098
- Buchberger A. From UBA to UBX: new words in the ubiquitin vocabulary. Trends Cell Biol 2002; 12:216 - 21; http://dx.doi.org/10.1016/S0962-8924(02)02269-9; PMID: 12062168
- Hwang GW, Sasaki D, Naganuma A. Overexpression of Rad23 confers resistance to methylmercury in saccharomyces cerevisiae via inhibition of the degradation of ubiquitinated proteins. Mol Pharmacol 2005; 68:1074 - 8; http://dx.doi.org/10.1124/mol.105.013516; PMID: 15998872
- Mah AL, Perry G, Smith MA, Monteiro MJ. Identification of ubiquilin, a novel presenilin interactor that increases presenilin protein accumulation. J Cell Biol 2000; 151:847 - 62; http://dx.doi.org/10.1083/jcb.151.4.847; PMID: 11076969
- Massey LK, Mah AL, Ford DL, Miller J, Liang J, Doong H, et al. Overexpression of ubiquilin decreases ubiquitination and degradation of presenilin proteins. J Alzheimers Dis 2004; 6:79 - 92; PMID: 15004330
- Kleijnen MF, Shih AH, Zhou P, Kumar S, Soccio RE, Kedersha NL, et al. The hPLIC proteins may provide a link between the ubiquitination machinery and the proteasome. Mol Cell 2000; 6:409 - 19; http://dx.doi.org/10.1016/S1097-2765(00)00040-X; PMID: 10983987
- Saliba RS, Pangalos M, Moss SJ. The ubiquitin-like protein Plic-1 enhances the membrane insertion of GABAA receptors by increasing their stability within the endoplasmic reticulum. J Biol Chem 2008; 283:18538 - 44; http://dx.doi.org/10.1074/jbc.M802077200; PMID: 18467327
- Hara T, Kamura T, Kotoshiba S, Takahashi H, Fujiwara K, Onoyama I, et al. Role of the UBL-UBA protein KPC2 in degradation of p27 at G1 phase of the cell cycle. Mol Cell Biol 2005; 25:9292 - 303; http://dx.doi.org/10.1128/MCB.25.21.9292-9303.2005; PMID: 16227581
- d’Azzo A, Bongiovanni A, Nastasi T. E3 ubiquitin ligases as regulators of membrane protein trafficking and degradation. Traffic 2005; 6:429 - 41; http://dx.doi.org/10.1111/j.1600-0854.2005.00294.x; PMID: 15882441
- N’Diaye EN, Hanyaloglu AC, Kajihara KK, Puthenveedu MA, Wu P, von Zastrow M, et al. The ubiquitin-like protein PLIC-2 is a negative regulator of G protein-coupled receptor endocytosis. Mol Biol Cell 2008; 19:1252 - 60; http://dx.doi.org/10.1091/mbc.E07-08-0775; PMID: 18199683
- Stieren ES, El Ayadi A, Xiao Y, Siller E, Landsverk ML, Oberhauser AF, et al. Ubiquilin-1 is a molecular chaperone for the amyloid precursor protein. J Biol Chem 2011; 286:35689 - 98; http://dx.doi.org/10.1074/jbc.M111.243147; PMID: 21852239
- Hiltunen M, Lu A, Thomas AV, Romano DM, Kim M, Jones PB, et al. Ubiquilin 1 modulates amyloid precursor protein trafficking and Abeta secretion. J Biol Chem 2006; 281:32240 - 53; http://dx.doi.org/10.1074/jbc.M603106200; PMID: 16945923
- De Strooper B, Annaert W. Proteolytic processing and cell biological functions of the amyloid precursor protein. J Cell Sci 2000; 113:1857 - 70; PMID: 10806097
- Suzuki T, Araki Y, Yamamoto T, Nakaya T. Trafficking of Alzheimer’s disease-related membrane proteins and its participation in disease pathogenesis. J Biochem 2006; 139:949 - 55; http://dx.doi.org/10.1093/jb/mvj121; PMID: 16788045
- Scheuermann S, Hambsch B, Hesse L, Stumm J, Schmidt C, Beher D, et al. Homodimerization of amyloid precursor protein and its implication in the amyloidogenic pathway of Alzheimer’s disease. J Biol Chem 2001; 276:33923 - 9; http://dx.doi.org/10.1074/jbc.M105410200; PMID: 11438549
- Small SA, Gandy S. Sorting through the cell biology of Alzheimer’s disease: intracellular pathways to pathogenesis. Neuron 2006; 52:15 - 31; http://dx.doi.org/10.1016/j.neuron.2006.09.001; PMID: 17015224
- El Ayadi A, Stieren ES, Barral JM, Boehning D. Ubiquilin-1 regulates amyloid precursor protein maturation and degradation by stimulating K63-linked polyubiquitination of lysine 688. Proc Natl Acad Sci U S A 2012; 109:13416 - 21; http://dx.doi.org/10.1073/pnas.1206786109; PMID: 22847417
- Knops J, Lieberburg I, Sinha S. Evidence for a nonsecretory, acidic degradation pathway for amyloid precursor protein in 293 cells. Identification of a novel, 22-kDa, beta-peptide-containing intermediate. J Biol Chem 1992; 267:16022 - 4; PMID: 1644790
- Koo EH, Sisodia SS, Archer DR, Martin LJ, Weidemann A, Beyreuther K, et al. Precursor of amyloid protein in Alzheimer disease undergoes fast anterograde axonal transport. Proc Natl Acad Sci U S A 1990; 87:1561 - 5; http://dx.doi.org/10.1073/pnas.87.4.1561; PMID: 1689489
- Kumar P, Ambasta RK, Veereshwarayya V, Rosen KM, Kosik KS, Band H, et al. CHIP and HSPs interact with beta-APP in a proteasome-dependent manner and influence Abeta metabolism. Hum Mol Genet 2007; 16:848 - 64; http://dx.doi.org/10.1093/hmg/ddm030; PMID: 17317785
- Andersen OM, Reiche J, Schmidt V, Gotthardt M, Spoelgen R, Behlke J, et al. Neuronal sorting protein-related receptor sorLA/LR11 regulates processing of the amyloid precursor protein. Proc Natl Acad Sci U S A 2005; 102:13461 - 6; http://dx.doi.org/10.1073/pnas.0503689102; PMID: 16174740
- Traub LM. Common principles in clathrin-mediated sorting at the Golgi and the plasma membrane. Biochim Biophys Acta 2005; 1744:415 - 37; http://dx.doi.org/10.1016/j.bbamcr.2005.04.005; PMID: 15922462
- Kim TY, Kim E, Yoon SK, Yoon JB. Herp enhances ER-associated protein degradation by recruiting ubiquilins. Biochem Biophys Res Commun 2008; 369:741 - 6; http://dx.doi.org/10.1016/j.bbrc.2008.02.086; PMID: 18307982
- Lauwers E, Jacob C, André B. K63-linked ubiquitin chains as a specific signal for protein sorting into the multivesicular body pathway. J Cell Biol 2009; 185:493 - 502; http://dx.doi.org/10.1083/jcb.200810114; PMID: 19398763
- Sahlender DA, Roberts RC, Arden SD, Spudich G, Taylor MJ, Luzio JP, et al. Optineurin links myosin VI to the Golgi complex and is involved in Golgi organization and exocytosis. J Cell Biol 2005; 169:285 - 95; http://dx.doi.org/10.1083/jcb.200501162; PMID: 15837803
- del Toro D, Alberch J, Lázaro-Diéguez F, Martín-Ibáñez R, Xifró X, Egea G, et al. Mutant huntingtin impairs post-Golgi trafficking to lysosomes by delocalizing optineurin/Rab8 complex from the Golgi apparatus. Mol Biol Cell 2009; 20:1478 - 92; http://dx.doi.org/10.1091/mbc.E08-07-0726; PMID: 19144827
- Laplantine E, Fontan E, Chiaravalli J, Lopez T, Lakisic G, Véron M, et al. NEMO specifically recognizes K63-linked poly-ubiquitin chains through a new bipartite ubiquitin-binding domain. EMBO J 2009; 28:2885 - 95; http://dx.doi.org/10.1038/emboj.2009.241; PMID: 19763089
- Borg JP, Ooi J, Levy E, Margolis B. The phosphotyrosine interaction domains of X11 and FE65 bind to distinct sites on the YENPTY motif of amyloid precursor protein. Mol Cell Biol 1996; 16:6229 - 41; PMID: 8887653
- King GD, Perez RG, Steinhilb ML, Gaut JR, Turner RS. X11alpha modulates secretory and endocytic trafficking and metabolism of amyloid precursor protein: mutational analysis of the YENPTY sequence. Neuroscience 2003; 120:143 - 54; http://dx.doi.org/10.1016/S0306-4522(03)00284-7; PMID: 12849748
- Sabo SL, Lanier LM, Ikin AF, Khorkova O, Sahasrabudhe S, Greengard P, et al. Regulation of beta-amyloid secretion by FE65, an amyloid protein precursor-binding protein. J Biol Chem 1999; 274:7952 - 7; http://dx.doi.org/10.1074/jbc.274.12.7952; PMID: 10075692
- Mueller HT, Borg JP, Margolis B, Turner RS. Modulation of amyloid precursor protein metabolism by X11alpha /Mint-1. A deletion analysis of protein-protein interaction domains. J Biol Chem 2000; 275:39302 - 6; http://dx.doi.org/10.1074/jbc.M008453200; PMID: 11010978
- Liang J, Yin C, Doong H, Fang S, Peterhoff C, Nixon RA, et al. Characterization of erasin (UBXD2): a new ER protein that promotes ER-associated protein degradation. J Cell Sci 2006; 119:4011 - 24; http://dx.doi.org/10.1242/jcs.03163; PMID: 16968747
- Huttunen HJ, Guénette SY, Peach C, Greco C, Xia W, Kim DY, et al. HtrA2 regulates beta-amyloid precursor protein (APP) metabolism through endoplasmic reticulum-associated degradation. J Biol Chem 2007; 282:28285 - 95; http://dx.doi.org/10.1074/jbc.M702951200; PMID: 17684015
- Heir R, Ablasou C, Dumontier E, Elliott M, Fagotto-Kaufmann C, Bedford FK. The UBL domain of PLIC-1 regulates aggresome formation. EMBO Rep 2006; 7:1252 - 8; http://dx.doi.org/10.1038/sj.embor.7400823; PMID: 17082820
- Viswanathan J, Haapasalo A, Böttcher C, Miettinen R, Kurkinen KM, Lu A, et al. Alzheimer’s disease-associated ubiquilin-1 regulates presenilin-1 accumulation and aggresome formation. Traffic 2011; 12:330 - 48; http://dx.doi.org/10.1111/j.1600-0854.2010.01149.x; PMID: 21143716
- Biswas N, Liu S, Ronni T, Aussenberg SE, Liu W, Fujita T, et al. The ubiquitin-like protein PLIC-1 or ubiquilin 1 inhibits TLR3-Trif signaling. PLoS One 2011; 6:e21153; http://dx.doi.org/10.1371/journal.pone.0021153; PMID: 21695056
- N’Diaye EN, Kajihara KK, Hsieh I, Morisaki H, Debnath J, Brown EJ. PLIC proteins or ubiquilins regulate autophagy-dependent cell survival during nutrient starvation. EMBO Rep 2009; 10:173 - 9; http://dx.doi.org/10.1038/embor.2008.238; PMID: 19148225
- Rothenberg C, Srinivasan D, Mah L, Kaushik S, Peterhoff CM, Ugolino J, et al. Ubiquilin functions in autophagy and is degraded by chaperone-mediated autophagy. Hum Mol Genet 2010; 19:3219 - 32; http://dx.doi.org/10.1093/hmg/ddq231; PMID: 20529957
- Rothenberg C, Monteiro MJ. Ubiquilin at a crossroads in protein degradation pathways. Autophagy 2010; 6:979 - 80; http://dx.doi.org/10.4161/auto.6.7.13118; PMID: 20729634
- Soba P, Eggert S, Wagner K, Zentgraf H, Siehl K, Kreger S, et al. Homo- and heterodimerization of APP family members promotes intercellular adhesion. EMBO J 2005; 24:3624 - 34; http://dx.doi.org/10.1038/sj.emboj.7600824; PMID: 16193067
- Munter LM, Voigt P, Harmeier A, Kaden D, Gottschalk KE, Weise C, et al. GxxxG motifs within the amyloid precursor protein transmembrane sequence are critical for the etiology of Abeta42. EMBO J 2007; 26:1702 - 12; http://dx.doi.org/10.1038/sj.emboj.7601616; PMID: 17332749