Abstract
Yeast prions are infectious proteins that spread exclusively by mating. The frequency of prions in the wild therefore largely reflects the rate of spread by mating counterbalanced by prion growth slowing effects in the host. We recently showed that the frequency of outcross mating is about 1% of mitotic doublings with 23–46% of total matings being outcrosses. These findings imply that even the mildest forms of the [PSI+], [URE3] and [PIN+] prions impart > 1% growth/survival detriment on their hosts. Our estimate of outcrossing suggests that Saccharomyces cerevisiae is far more sexual than previously thought and would therefore be more responsive to the adaptive effects of natural selection compared with a strictly asexual yeast. Further, given its large effective population size, a growth/survival detriment of > 1% for yeast prions should strongly select against prion-infected strains in wild populations of Saccharomyces cerevisiae.
Prions are known to cause a variety of fatal neurodegenerative diseases in humans, livestock and wildlife. A number of prion proteins have been characterized in the budding yeast Saccharomyces cerevisiae, but their effects on yeast fitness remain controversial. The most commonly studied variants of the yeast prions, [PSI+], [URE3] and [PIN+] appear relatively harmless, though the identification of toxic and lethal variants of these prionsCitation1 indicates that laboratory growth conditions artificially select for mild prion variants. Some claim that prions are beneficial for yeast,Citation2-Citation6 and have been naturally selected to promote evolvability.Citation6 However, our recent work demonstrated that even the mildest forms of [PSI+], [URE3] and [PIN+] are deleterious diseases that slow cell growth or impair survival by greater than 1%.Citation7 We suggest that the detrimental nature of infectious prions hinders their spread in the wild and contributes to their rarity in natural yeast isolates.
While budding yeast predominantly reproduce mitotically, nutrient deprivation induces meiosis in diploids, with each cell producing four haploid spores. Spores are either mating type a or α, and mating partners must have different mating types. Therefore mating can occur between spores with opposite mating types within an ascus (intratetrad) or with a spore originating from a different diploid parent (outcrossing). Alternatively, if germinated spores do not mate immediately, cells can undergo a mating-type switch (homothallism) which produces a mixture of a and α cells from the same clone that can subsequently mate. Outcrossing is the predominant mode of prion transmission because with homothallism and intratetrad mating, spores within a single tetrad will likely be all infected or all uninfected.
The frequency of prion infections in the wild is affected by the rate of transmission, spontaneous loss or gain of the prion state and the fitness effects that prions impart on their host (). If yeast prions provide a benefit for their hosts as suggested by others, then natural selection would act to increase their frequency in natural populations. Based on this assumption, and the fact that they spread easily through mating, we would expect prions to be common in nature. However, surveys from wild and industrial Saccharomyces isolates found that most yeast prions are extremely rare in natural populations.Citation4,Citation8-Citation11 A recent study found [PSI+] in < 1% and [PIN+] in ~6% of 700 wild isolates surveyed.Citation4 Furthermore, attempts to detect prions in several other yeast species (Saccharomyces paradoxus, Saccharomyces bayanus, Naumovozyma castellii, Saccharomyces dairensis, Saccharomyces exiguus, Saccharomyces unisporus, Saccharomyces kluyveri and Pichia methanolica) were unsuccessful.Citation9
Figure 1. The equilibrium frequency of prions in wild populations is determined by spread, growth slowing effects and loss/gain of the prion state.
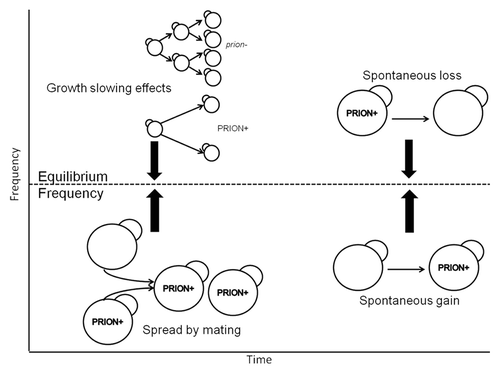
The rarity of yeast prions in the wild could reflect negative fitness consequences, high rates of prion loss, infrequent transmission or a combination of these. To quantify transmission rates in the wild, we used the naturally occurring 2 μ DNA plasmid as a model for infectious element spread among natural isolates. Like yeast prions, 2 μ plasmids are exclusively intracellular,Citation12 and thus spread solely through outcross mating. We used the known rate of spontaneous loss and gain of 2 μ DNA,Citation12 the known growth detriment of the plasmid (1−3%Citation12,Citation13) and its frequency in nature (38/70)Citation8 to estimate outcrossing rates for yeast. Our model projected that yeast outcross once per 100 cellular divisions,Citation7 an estimate that is much higher than previous reports for S. cerevisiae and S. paradoxus.Citation14,Citation15 Genomic-based methods utilizing recombination as a proxy for mating produced estimates of one outcross per 50,000 mitotic divisions for S. cerevisiaeCitation15 and one per 100,000 divisions for S. paradoxus.Citation14 Recombination-based methods for estimating outcrossing rely heavily on the detectability of heterozygotes. In highly clonal populations, occasional outcrossed mating may not be detected with genetic methods because spatially proximate mating partners are highly relatedCitation16 or because mitotic recombination has resulted in a loss of heterozygosity.Citation17 Furthermore, the power to detect recombination (and therefore mating) is reduced for populations with low diversity and low rates of recombination,Citation18 a potential problem for some yeast populations.Citation17
In addition to estimating the overall outcrossing frequency, we were able to use genetic heterozygosity as an indicator of the proportion of matings that are outcrossed (vs. intratetrad or homothallic). A previous study,Citation17 that we earlier failed to cite, showed that wild S. cerevisiae isolates are largely heterozygous, and thus that mating is commonly of the outcross type, thereby, pre-confirming our findings in this regard. We used nucleotide sequences at RNQ1 (coding for Rnq1p, the protein that forms the [PIN+] prion) to estimate population heterozygosity because the high polymorphism at this locusCitation10 facilitated the detection of heterozygotes.Citation7 Our results indicated that 23–46% of matings are outcrossed, but this is a minimum as it does not take mitotic recombination into effect. Our findings are consistent with other experimentally derived mating frequencies. Murphy and Zehyl (2010) found that yeast grown in dense cultures outcross 10–25% of the time, while spores in physically adjacent asci outcross with frequencies ranging from 10–50%.Citation16 Similar rates of outcrossing were reported for a geographically isolated population of S. cerevisiae in New Zealand, where ~20% outcrossing was observed among genetically subdivided lineages.Citation19
Our estimate of outcrossing frequency equates to the transmission rate for infectious prions in wild yeast. Using this information we were able to subsequently estimate the fitness effects of [PSI+], [URE3] and [PIN+], again assuming that the observed frequency of prions in the wild reflects transmission rate, spontaneous loss or gain of the prion state (derived experimentally for [PSI+]Citation20 and for [URE3]Citation21,Citation22) and any fitness effects afforded to the host (). With our estimate of 1% outcrossing and the observed prion frequencies of 1%, 1% and 16% for [PSI+], [URE3] and [PIN+]Citation4,Citation8 respectively, we showed that yeast prions slow cellular growth by at least 1% ().
Figure 2. Relative growth rate of [PRION+] vs. [prion-] cells for varying frequencies of mating, prion loss and equilibrium prion prevalence. For all calculations, the probability of spontaneous prion gain = 1.6 × 10−6. Experimentally determined values of each variable (prevalence = 0.01, mating = 0.01, loss = 0.00001) are indicated by *. Horizontal lines indicate relative growth rate = 1, where the fitness effects of the prion are neutral. Relative growth rate > 1 indicates a survival advantage for [PRION+] cells, while relative growth rate < 1 indicates growth detriment for [PRION+] cells.
![Figure 2. Relative growth rate of [PRION+] vs. [prion-] cells for varying frequencies of mating, prion loss and equilibrium prion prevalence. For all calculations, the probability of spontaneous prion gain = 1.6 × 10−6. Experimentally determined values of each variable (prevalence = 0.01, mating = 0.01, loss = 0.00001) are indicated by *. Horizontal lines indicate relative growth rate = 1, where the fitness effects of the prion are neutral. Relative growth rate > 1 indicates a survival advantage for [PRION+] cells, while relative growth rate < 1 indicates growth detriment for [PRION+] cells.](/cms/asset/85ae8822-08fb-473d-98df-3dd79e08dbd9/kprn_a_10924845_f0002.gif)
Masel and Griswold (2009) used theoretical modeling to extrapolate the selective forces acting against [PSI+] and in agreement with our data found that [PSI+] is usually deleterious.Citation23 Their results however, suggest that the detriment of [PSI+] is 100 times lower than our estimate of 1%. This difference is likely due to their assumption that an outcrossing frequency of 0.001% as estimated for S. paradoxus,Citation14 was generalizeable for S. cerevisiae. Recent genomic surveys have shown that the population structure of S. paradoxus differs dramatically from S. cerevisiae, with the latter species showing extensive mixing of lineages among subpopulations.Citation24 Thus, mating rates estimated for S. paradoxus may not hold true for S. cerevisiae,Citation17 and likely resulted in a substantial underestimate of [PSI+] detriment with theoretical models. Indeed our own analyses showed that estimates of prion growth detriment are extremely sensitive to changes in mating frequency ().
Some have proposed that prions promote cell survival.Citation5,Citation6,Citation25 This hypothesis is mainly based on claims that [PSI+] can be an advantage under some circumstance in some strains,Citation3,Citation4 and is induced in the presence of certain cellular stressors.Citation26 Furthermore, ure2 cells (void of Ure2p, the protein monomer of [URE3]) were more tolerant of certain alkali metals ions compared with wild type,Citation27 even though [URE3] slows the growth of most strains on the usual laboratory medium.Citation28 The reported resistance of [PSI+] cells to heat and high ethanol stressCitation3 was not confirmed by a later report,Citation6 and the benefits reported by the later report,Citation6 were also not confirmed by others using identical strains.Citation29 The reports of [PSI+] induction by stress conditions used a non-natural Sup35 prion domain known to have an elevated frequency of generating [PSI+].Citation26 In our recent workCitation7 we tested whether the stress conditions reported to induce [PSI+] with the artificial prion domain would do so with the native Sup35p prion domain.Citation7 We have been unable to observe toxicity-based [PSI+] induction, suggesting that cells are not adapted to form prions in response to stress.
Despite the lack of experimental evidence that [PSI+], [URE3] and [PIN+] are beneficial, an adaptive role for yeast prions is often proposed in the literature. Evolutionary conservation of yeast prion forming ability is often cited as evidence that they provide a fitness advantage.Citation2,Citation5,Citation30 A few experimental studies have shown that overexpression of prion domains (domains necessary and sufficient for amyloidization) and occasionally full length proteins from various Ascomycetes will induce prion formation by that domain/protein in S. cerevisiae cells.Citation9,Citation31-Citation33 These cross-species inductions are interpreted as indicators of evolutionary conservation of prion-forming ability in yeast. However, this inference fails to acknowledge the artificiality of induction conditions (overexpression of prion domains and the use of inter-species fusion proteins); such conditions would not be encountered by yeast in natural environments. Furthermore, S. cerevisiae proteins can be induced to form prions from other species, but prion forming ability has not been assessed in the majority of yeasts.Citation9,Citation33 In addition, the Ure2p proteins of Kluyveromyces lactisCitation34 and Saccharomyces castellii,Citation35 are unable to form [URE3]. The K. lactis report from Safadi et al. (2011) is particularly important since this yeast is closely related to S. cerevisiae and the authors showed that in the K. lactis host, the K. lactis Ure2p could not form [URE3].Citation34 Ure2p of Candida albicans can form the [URE3] prion in Candida glabrata,Citation36 but Candida glabrata Ure2p is unable to form [URE3] in either its own cells or in S. cerevisiae cells.Citation36,Citation37 Thus, prion-forming ability of Ure2p is generally not conserved. Further, the existence of a phenomenon in many related species does not provide evidence that it benefits the organisms. Most vertebrates suffer from broken legs and arthritis, but this hardly qualifies these phenomena as helping the animal. Of course, even a broken leg can (rarely) be beneficial as discussed elsewhere.Citation7
Molecular studies of yeast prion proteins also claim that prion-forming domain sequences have been conserved by evolution. Jensen et al. (2001) reported evolutionary constraint for the Sup35 N and M-domains based on relatively low rates of non-synonymous compared with synonymous substitution for this region.Citation38 Compared with a more recent study,Citation11 the number of Sup35 polymorphisms reported by Jensen et al. (2001) is relatively low, likely due to a limited number and narrow range of strains analyzed. Additionally, functional constraint for Sup35 N and M domains cannot be solely attributed to prion formation, as these domains also play an active role in mRNA degradation.Citation39 Likewise, the prion domain of Ure2p prevents degradation of the protein in vivoand may affect interactions with other proteins.Citation40 Further, since prion-forming ability does not depend on sequence, but mostly on amino acid content,Citation41 even if prion-forming ability were selected and conserved, it would not necessarily result in conservation of amino acid sequence.
Though prion-like domains can be found in a variety of budding yeast,Citation42 this cannot be taken as direct evidence for evolutionary constraint of prions in fungi,Citation43 particularly given their non-prion functions.Citation39 Moreover, prions documented in wild yeast isolates are likely the most benign, and thus easily detected, variants. Lethal and toxic prions are generally not detectable in the laboratory under standard growth conditions because they abolish or severely inhibit cell growth.Citation1 Still, even a 1% growth detriment for benign prions could have substantial selection effects in wild populations. Neutral theory of evolution predicts that alleles are deleterious when s > 1/(2N) (where s is the selection coefficient against an allele and N is the number of breeding individuals; calculated with N from,Citation23 s > 10−8 would be deleterious in yeast).Citation44 Their large population size would therefore make yeast sensitive to modest fitness effects from prions.Citation23
Our finding that Saccharomyces cerevisiae is far more sexual than previously thought has far reaching implications for the evolutionary dynamics of this species. Sex increases genetic variability, promotes adaptation and allows natural selection to proceed more efficiently.Citation45 Through recombination, sex can bring together multiple beneficial mutations in a single generation, whereas a purely clonal population would have to acquire successive beneficial mutations along a single lineage to incur the same fitness effects.Citation46 The novel gene combinations created by sex increase fitness variation among individuals, which in turn increases the efficaciousness of natural selection. When grown in glucose limiting media at elevated temperatures (37°C), sexual yeast had higher relative fitness over 300 generations as compared with isogenic asexual yeast. The fitness advantage for sexual yeast was not observed in benign growth conditions (30°C in glucose limiting media), where both asexual and sexual yeast are sufficiently adapted, precluding fitness variation necessary for natural selection.Citation47 In nature, wild yeast are likely to encounter extreme growth conditions such as freeze-thaw cycles, elevated temperatures, nutrient deprivation, etc. Thus in the wild we would expect sexual yeasts to adapt more efficiently than their asexual counterparts.
The loss or acquisition of the prion state is associated with phenotypic changes in the cell. For example, [PIN+]-infected cells gain the ability to induce other prions,Citation48,Citation49 and [URE3]-infected cells inherit a defect in nitrogen catabolite repression.Citation50 [PSI+]-infected cells have reduced translational termination,Citation51 which allows some genes with internal stop codons and nonsense mutations to be translated.Citation52,Citation53 Despite speculation that the transient and heritable phenotypic changes observed in prion-infected laboratory strains could promote evolvability,Citation5,Citation6 selection for evolvability has never been documented in yeastCitation15,Citation24 and is generally not feasible for sexual populations. First of all, most novel mutations are deleterious.Citation54 Indeed, the Saccharomyces cerevisiae genome as a whole is under purifying selection,Citation24 an indication that there is pressure to reduce accumulation of deleterious alleles. Selection for evolvability is well documented in asexual organisms, because modifiers that increase mutation rate can hitchhike to higher frequencies when they are linked to a positively selected locus.Citation55-Citation58 However, with 1% outcrossing, Saccharomyces cerevisiae is by and large sexual. We would expect recombination to break down associations between mutation modifiers and beneficial allelesCitation59 which will rapidly result in the loss of modifiers from sexual populations.Citation60 Thus selection for evolvability is unlikely to explain phenotypic variation associated with prions. A more straight forward explanation is that prion mediated disruption of intracellular protein levels changes the cellular environment.Citation61
Given the complexities of disease transmission in wild yeast populations, it is difficult to predict if negative selection is sufficient to counteract spread by mating and decrease prion prevalence over time. This is especially true for Saccharomyces cerevisiae, given ecologically-driven differences in mating propensity observed for isolates in different environments. Magwene et al. (2011) found that outcrossing rates ranged from 36–52% for domesticated yeast (e.g., vineyard, clinical strains) as compared with 2% for strains isolated in natural environments (e.g., tree bark, fruit). Domestication could increase contact rates among different yeast strains and create mating opportunities that would otherwise be rare for isolates in low-density natural environments.Citation17 Our own data showed that 14/15 [PIN+] strains originated from domesticated environments.Citation7 Additionally, 12/15 [PIN+] strains were heterozygous, a likely indication that prions behave as sexually transmitted diseases in yeast. Taken together, these findings suggest that prion prevalence for yeast in human-associated environments is higher than for undomesticated yeast due to increased sexual transmission in highly outcrossed, domesticated strains.
Our interpretation of the rare occurrence of yeast prions in nature is that they are deleterious, and this negative selection outpaces prion transmission in spite of substantial rates of outcrossing in Saccharomyces cerevisiae Citation7. Though they have been extensively studied in the laboratory, few studies address prion disease dynamics in natural populations. Ecological factors such as mating clearly influence prion transmission rates and it would be interesting to determine if there are additional environmental variables that can influence the spread of prions in the wild.
Acknowledgments
We thank Frank Shewmaker and Dmitry Kryndushkin (Uniformed Services University for the Health Sciences, Bethesda, MD) for useful comments. This work was supported in part by the Intramural Program of the National Institute of Diabetes and Digestive and Kidney Diseases.
Disclosure of Potential Conflicts of Interest
No potential conflict of interest was disclosed.
References
- McGlinchey RP, Kryndushkin D, Wickner RB. Suicidal [PSI+] is a lethal yeast prion. Proc Natl Acad Sci U S A 2011; 108:5337 - 41; http://dx.doi.org/10.1073/pnas.1102762108; PMID: 21402947
- True HL, Berlin I, Lindquist SL. Epigenetic regulation of translation reveals hidden genetic variation to produce complex traits. Nature 2004; 431:184 - 7; http://dx.doi.org/10.1038/nature02885; PMID: 15311209
- Eaglestone SS, Cox BS, Tuite MF. Translation termination efficiency can be regulated in Saccharomyces cerevisiae by environmental stress through a prion-mediated mechanism. EMBO J 1999; 18:1974 - 81; http://dx.doi.org/10.1093/emboj/18.7.1974; PMID: 10202160
- Halfmann R, Jarosz DF, Jones SK, Chang A, Lancaster AK, Lindquist S. Prions are a common mechanism for phenotypic inheritance in wild yeasts. Nature 2012; 482:363 - 8; http://dx.doi.org/10.1038/nature10875; PMID: 22337056
- Shorter J, Lindquist S. Prions as adaptive conduits of memory and inheritance. Nat Rev Genet 2005; 6:435 - 50; http://dx.doi.org/10.1038/nrg1616; PMID: 15931169
- True HL, Lindquist SL. A yeast prion provides a mechanism for genetic variation and phenotypic diversity. Nature 2000; 407:477 - 83; http://dx.doi.org/10.1038/35035005; PMID: 11028992
- Kelly AC, Shewmaker FP, Kryndushkin D, Wickner RB. Sex, prions, and plasmids in yeast. Proceedings of the National Academy of Sciences 2012.
- Nakayashiki T, Kurtzman CP, Edskes HK, Wickner RB. Yeast prions [URE3] and [PSI+] are diseases. Proc Natl Acad Sci U S A 2005; 102:10575 - 80; http://dx.doi.org/10.1073/pnas.0504882102; PMID: 16024723
- Chernoff YO, Galkin AP, Lewitin E, Chernova TA, Newnam GP, Belenkiy SM. Evolutionary conservation of prion-forming abilities of the yeast Sup35 protein. Mol Microbiol 2000; 35:865 - 76; http://dx.doi.org/10.1046/j.1365-2958.2000.01761.x; PMID: 10692163
- Resende CG, Outeiro TF, Sands L, Lindquist S, Tuite MF. Prion protein gene polymorphisms in Saccharomyces cerevisiae. Mol Microbiol 2003; 49:1005 - 17; http://dx.doi.org/10.1046/j.1365-2958.2003.03608.x; PMID: 12890024
- Bateman DA, Wickner RB. [PSI+] Prion transmission barriers protect Saccharomyces cerevisiae from infection: intraspecies ‘species barriers’. Genetics 2012; 190:569 - 79; http://dx.doi.org/10.1534/genetics.111.136655; PMID: 22095075
- Futcher AB, Cox BS. Maintenance of the 2 microns circle plasmid in populations of Saccharomyces cerevisiae. J Bacteriol 1983; 154:612 - 22; PMID: 6341357
- Mead DJ, Gardner DCJ, Oliver SG. The yeast 2 micron plasmid: strategies for the survival of a selfish DNA. Mol Gen Genet 1986; 205:417 - 21; http://dx.doi.org/10.1007/BF00338076; PMID: 3550381
- Tsai IJ, Bensasson D, Burt A, Koufopanou V. Population genomics of the wild yeast Saccharomyces paradoxus: Quantifying the life cycle. Proc Natl Acad Sci U S A 2008; 105:4957 - 62; http://dx.doi.org/10.1073/pnas.0707314105; PMID: 18344325
- Ruderfer DM, Pratt SC, Seidel HS, Kruglyak L. Population genomic analysis of outcrossing and recombination in yeast. Nat Genet 2006; 38:1077 - 81; http://dx.doi.org/10.1038/ng1859; PMID: 16892060
- Murphy HA, Zeyl CW. Yeast sex: surprisingly high rates of outcrossing between asci. PLoS One 2010; 5:e10461; http://dx.doi.org/10.1371/journal.pone.0010461; PMID: 20463964
- Magwene PM, Kayıkçı Ö, Granek JA, Reininga JM, Scholl Z, Murray D. Outcrossing, mitotic recombination, and life-history trade-offs shape genome evolution in Saccharomyces cerevisiae. Proc Natl Acad Sci U S A 2011; 108:1987 - 92; http://dx.doi.org/10.1073/pnas.1012544108; PMID: 21245305
- Posada D, Crandall KA. Evaluation of methods for detecting recombination from DNA sequences: computer simulations. Proc Natl Acad Sci U S A 2001; 98:13757 - 62; http://dx.doi.org/10.1073/pnas.241370698; PMID: 11717435
- Goddard MR, Anfang N, Tang R, Gardner RC, Jun C. A distinct population of Saccharomyces cerevisiae in New Zealand: evidence for local dispersal by insects and human-aided global dispersal in oak barrels. Environ Microbiol 2010; 12:63 - 73; http://dx.doi.org/10.1111/j.1462-2920.2009.02035.x; PMID: 19691498
- Liebman SW, Bagriantsev SN, Derkatch IL. Biochemical and genetic methods for characterization of [PIN+] prions in yeast. Methods 2006; 39:23 - 34; http://dx.doi.org/10.1016/j.ymeth.2006.04.010; PMID: 16793281
- Wickner RB. [URE3] as an altered URE2 protein: evidence for a prion analog in Saccharomyces cerevisiae. Science 1994; 264:566 - 9; http://dx.doi.org/10.1126/science.7909170; PMID: 7909170
- Bradley ME, Edskes HK, Hong JY, Wickner RB, Liebman SW. Interactions among prions and prion “strains” in yeast. Proc Natl Acad Sci U S A 2002; 99:Suppl 4 16392 - 9; http://dx.doi.org/10.1073/pnas.152330699; PMID: 12149514
- Masel J, Griswold CK. The strength of selection against the yeast prion [PSI+]. [PSI+] Genetics 2009; 181:1057 - 63; http://dx.doi.org/10.1534/genetics.108.100297; PMID: 19153253
- Liti G, Carter DM, Moses AM, Warringer J, Parts L, James SA, et al. Population genomics of domestic and wild yeasts. Nature 2009; 458:337 - 41; http://dx.doi.org/10.1038/nature07743; PMID: 19212322
- Uptain SM, Lindquist S. Prions as protein-based genetic elements. Annu Rev Microbiol 2002; 56:703 - 41; http://dx.doi.org/10.1146/annurev.micro.56.013002.100603; PMID: 12142498
- Tyedmers J, Madariaga ML, Lindquist S. Prion switching in response to environmental stress. PLoS Biol 2008; 6:e294; http://dx.doi.org/10.1371/journal.pbio.0060294; PMID: 19067491
- Withee JL, Sen R, Cyert MS. Ion tolerance of Saccharomyces cerevisiae lacking the Ca2+/CaM-dependent phosphatase (calcineurin) is improved by mutations in URE2 or PMA1. Genetics 1998; 149:865 - 78; PMID: 9611198
- Schwimmer C, Masison DC. Antagonistic interactions between yeast [PSI(+)] and [URE3] prions and curing of [URE3] by Hsp70 protein chaperone Ssa1p but not by Ssa2p. Mol Cell Biol 2002; 22:3590 - 8; http://dx.doi.org/10.1128/MCB.22.11.3590-3598.2002; PMID: 11997496
- Namy O, Galopier A, Martini C, Matsufuji S, Fabret C, Rousset JP. Epigenetic control of polyamines by the prion [PSI+]. [PSI+] Nat Cell Biol 2008; 10:1069 - 75; http://dx.doi.org/10.1038/ncb1766; PMID: 19160487
- Sparrer HE, Santoso A, Szoka FC Jr., Weissman JS. Evidence for the prion hypothesis: induction of the yeast [PSI+] factor by in vitro- converted Sup35 protein. Science 2000; 289:595 - 9; http://dx.doi.org/10.1126/science.289.5479.595; PMID: 10915616
- Kushnirov VV, Kochneva-Pervukhova NV, Chechenova MB, Frolova NS, Ter-Avanesyan MD. Prion properties of the Sup35 protein of yeast Pichia methanolica. EMBO J 2000; 19:324 - 31; http://dx.doi.org/10.1093/emboj/19.3.324; PMID: 10654931
- Tanaka M, Chien P, Yonekura K, Weissman JS. Mechanism of cross-species prion transmission: an infectious conformation compatible with two highly divergent yeast prion proteins. Cell 2005; 121:49 - 62; http://dx.doi.org/10.1016/j.cell.2005.03.008; PMID: 15820678
- Nakayashiki T, Ebihara K, Bannai H, Nakamura Y. Yeast [PSI+] “prions” that are crosstransmissible and susceptible beyond a species barrier through a quasi-prion state. Mol Cell 2001; 7:1121 - 30; http://dx.doi.org/10.1016/S1097-2765(01)00259-3; PMID: 11430816
- Safadi RA, Talarek N, Jacques N, Aigle M. Yeast prions: could they be exaptations? The URE2/[URE3] system in Kluyveromyces lactis. FEMS Yeast Res 2011; 11:151 - 3; http://dx.doi.org/10.1111/j.1567-1364.2010.00700.x; PMID: 21235712
- Edskes HK, McCann LM, Hebert AM, Wickner RB. Prion variants and species barriers among Saccharomyces Ure2 proteins. Genetics 2009; 181:1159 - 67; http://dx.doi.org/10.1534/genetics.108.099929; PMID: 19124570
- Edskes HK, Wickner RB. The [URE3] prion in Candida. [Epub ahead of print] Eukaryot Cell 2013; 12:551 - 8; http://dx.doi.org/10.1128/EC.00015-13; PMID: 23397567
- Edskes HK, Engel A, McCann LM, Brachmann A, Tsai HF, Wickner RB. Prion-forming ability of Ure2 of yeasts is not evolutionarily conserved. Genetics 2011; 188:81 - 90; http://dx.doi.org/10.1534/genetics.111.127217; PMID: 21368275
- Jensen MA, True HL, Chernoff YO, Lindquist S. Molecular population genetics and evolution of a prion-like protein in Saccharomyces cerevisiae. Genetics 2001; 159:527 - 35; PMID: 11606530
- Hosoda N, Kobayashi T, Uchida N, Funakoshi Y, Kikuchi Y, Hoshino S, et al. Translation termination factor eRF3 mediates mRNA decay through the regulation of deadenylation. J Biol Chem 2003; 278:38287 - 91; http://dx.doi.org/10.1074/jbc.C300300200; PMID: 12923185
- Shewmaker F, Mull L, Nakayashiki T, Masison DC, Wickner RB. Ure2p function is enhanced by its prion domain in Saccharomyces cerevisiae. Genetics 2007; 176:1557 - 65; http://dx.doi.org/10.1534/genetics.107.074153; PMID: 17507672
- Ross ED, Baxa U, Wickner RB. Scrambled prion domains form prions and amyloid. Mol Cell Biol 2004; 24:7206 - 13; http://dx.doi.org/10.1128/MCB.24.16.7206-7213.2004; PMID: 15282319
- Harrison LB, Yu Z, Stajich JE, Dietrich FS, Harrison PM. Evolution of budding yeast prion-determinant sequences across diverse fungi. J Mol Biol 2007; 368:273 - 82; http://dx.doi.org/10.1016/j.jmb.2007.01.070; PMID: 17320905
- Schwenk K. A utilitarian approach to evolutionary constraint. Zoology 1995; 98:251 - 62
- Wright S. Evolution in Mendelian populations. Genetics 1931; 16:97 - 159; PMID: 17246615
- Barton NH, Charlesworth B. Why sex and recombination?. Science 1998; 281:1986 - 90; http://dx.doi.org/10.1126/science.281.5385.1986; PMID: 9748151
- Muller HJ. Some genetic aspects of sex. Am Nat 1932; 66:118 - 38; http://dx.doi.org/10.1086/280418
- Goddard MR, Godfray HCJ, Burt A. Sex increases the efficacy of natural selection in experimental yeast populations. Nature 2005; 434:636 - 40; http://dx.doi.org/10.1038/nature03405; PMID: 15800622
- Derkatch IL, Bradley ME, Hong JY, Liebman SW. Prions affect the appearance of other prions: the story of [PIN(+)]. [PIN+] Cell 2001; 106:171 - 82; http://dx.doi.org/10.1016/S0092-8674(01)00427-5; PMID: 11511345
- Derkatch IL, Bradley ME, Zhou P, Chernoff YO, Liebman SW. Genetic and environmental factors affecting the de novo appearance of the [PSI+] prion in Saccharomyces cerevisiae. Genetics 1997; 147:507 - 19; PMID: 9335589
- Wickner RB. [URE3] as an altered URE2 protein: evidence for a prion analog in Saccharomyces cerevisiae. Science 1994; 264:566 - 9; http://dx.doi.org/10.1126/science.7909170; PMID: 7909170
- Liebman SW, Sherman F. Extrachromosomal psi+ determinant suppresses nonsense mutations in yeast. J Bacteriol 1979; 139:1068 - 71; PMID: 225301
- Cox BS, Tuite MF, McLaughlin CS. The psi factor of yeast: a problem in inheritance. Yeast 1988; 4:159 - 78; http://dx.doi.org/10.1002/yea.320040302; PMID: 3059716
- Firoozan M, Grant CM, Duarte JAB, Tuite MF. Quantitation of readthrough of termination codons in yeast using a novel gene fusion assay. Yeast 1991; 7:173 - 83; http://dx.doi.org/10.1002/yea.320070211; PMID: 1905859
- Taddei F, Radman M, Maynard-Smith J, Toupance B, Gouyon PH, Godelle B. Role of mutator alleles in adaptive evolution. Nature 1997; 387:700 - 2; http://dx.doi.org/10.1038/42696; PMID: 9192893
- Tröbner W, Piechocki R. Competition between isogenic mutS and mut+ populations of Escherichia coli K12 in continuously growing cultures. Mol Gen Genet 1984; 198:175 - 6; http://dx.doi.org/10.1007/BF00328719; PMID: 6394962
- Sniegowski PD, Gerrish PJ, Lenski RE. Evolution of high mutation rates in experimental populations of E. coli. Nature 1997; 387:703 - 5; http://dx.doi.org/10.1038/42701; PMID: 9192894
- Shaver AC, Dombrowski PG, Sweeney JY, Treis T, Zappala RM, Sniegowski PD. Fitness evolution and the rise of mutator alleles in experimental Escherichia coli populations. Genetics 2002; 162:557 - 66; PMID: 12399371
- Chao L, Cox EC. Competition between high and low mutating strains of Escherichia coli. Evolution 1983; 37:125 - 34; http://dx.doi.org/10.2307/2408181
- Leigh EG Jr.. Natural selection and mutability. Am Nat 1970; 104:301 - 5; http://dx.doi.org/10.1086/282663
- Raynes Y, Gazzara MR, Sniegowski PD. Mutator dynamics in sexual and asexual experimental populations of yeast. BMC Evol Biol 2011; 11:158; http://dx.doi.org/10.1186/1471-2148-11-158; PMID: 21649918
- Partridge L, Barton NH. Evolving evolvability. Nature 2000; 407:457 - 8; http://dx.doi.org/10.1038/35035173; PMID: 11028981