Abstract
A diagnostics of infectious diseases can be done by the immunologic methods or by the amplification of nucleic acid specific to contagious agent using polymerase chain reaction. However, in transmissible spongiform encephalopathies, the infectious agent, prion protein (PrPSc), has the same sequence of nucleic acids as a naturally occurring protein. The other issue with the diagnosing based on the PrPSc detection is that the pathological form of prion protein is abundant only at late stages of the disease in a brain. Therefore, the diagnostics of prion protein caused diseases represent a sort of challenges as that hosts can incubate infectious prion proteins for many months or even years. Therefore, new in vivo assays for detection of prion proteins and for diagnosis of their relation to neurodegenerative diseases are summarized. Their applicability and future prospects in this field are discussed with particular aim at using quantum dots as fluorescent labels.
Introduction
Alzheimer disease (AD) is characterized by the formation of senile plaques composed of the amyloid-β (Aβ) peptide and neurofibrillary tangles composed of hyperphosphorylated Tau. However, it is the accumulation of Aβ in the brain that appears to be critical for the pathogenesis of AD. The prion protein (PrP, ) is involved in neurodegeneration via its conversion from the normal cellular form, PrPC, to the infectious form, PrPSc, which is the causative agent of the transmissible spongiform encephalopathies (TSEs), including Creutzfeldt-Jakob disease (CJD).Citation2-Citation4 While there is an established role for PrPC in TSEs, the physiological role of PrPC has still not been fully elucidated. Roles in metal homeostasis, neuroprotective signaling, lymphocyte activation, neurite growth, synaptogenesis, cellular signaling, cell viability, and cellular response to oxidative stress have all been proposed.Citation5-Citation10 There are many neuropathological similarities and genetic links between AD and prion diseases. The coexistence of AD pathology in CJD has been reportedCitation11 and PrPC has been shown to co-localize with Aβ in plaques. These PrPC-Aβ plaques have been found in most CJD patients with associated AD-type pathology and it has been proposed that PrPC may promote Aβ plaque formation.Citation12,Citation13 A genetic correlation between PrPC and AD has also been reported.Citation14,Citation15 A systematic meta-analysis of AD genetic association studies revealed that the gene encoding PrPC (PRNP) is a potential AD susceptibility gene and the Met/Val 129 polymorphism in PRNP has been determined as a risk factor for early-onset AD.Citation16
Figure 1. Three-dimensional (3D) structure of human PrP. The structure of the α-helical form of rPrP(90–231) resembles that of PrPC. rPrP(90–231) is viewed from the interface where PrPSc is thought to bind to PrPC. The color scheme is as follows: α-helices A (residues 144–157), B (172–193), and C (200–227) in pink; disulphide between Cys-179 and Cys-214 in yellow; conserved hydrophobic region composed of residues 113–126 in red; loops in gray; residues 129–134 in green encompassing strand S1 and residues 159–165 in blue encompassing strand S2; the arrows span residues 129–131 and 161–163, as these show a closer resemblance to β-sheet (155).Citation1 The data source was the internet proteomic database Expasy (www.expasy.org). For data processing software Matlab version 7.9.0 (The MathWorks, Inc.) was used.
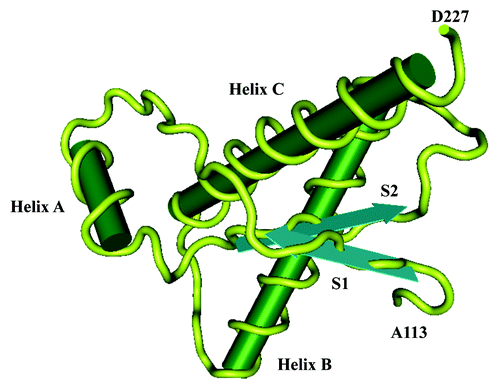
Current Diagnostics of Prion Protein Related Diseases
Diagnostics of infectious diseases is a relatively well-established area. It can be done either by the immunologic methods or by the amplification of a nucleic acid specific to infectious agent using polymerase chain reaction (PCR).Citation17 However, in TSEs, the infectious agent, PrP, has the same sequence of nucleic acids as a naturally occurring protein. The other issue with the diagnosing based on the PrPSc detection is that the pathological form of PrP is abundant only at late stages of the disease in a brain. Therefore, the diagnostics of PrP caused diseases represent a sort of challengesCitation18 as that hosts can incubate infectious prion proteins for many months or even years. During this period, they exhibit no overt clinical symptoms. Incubation period for some human prion diseases can be as long as 40 years.Citation19 The incubation time of the first human transmissible spongiform encephalopathy “Kuru” described by Prof. Gajdusek in the late 1950s was estimated within the range from 21 to 40 years.Citation20,Citation21
Immunological detection of PrPSc post mortem
Generally, the majority of analytical diagnostic methods rely on the proteolytic removal of endogenous PrPC prior to detection of PrPSc (). PrPSc is relatively resistant toward proteolytic degradation whereas PrPC is entirely digested by Proteinase K. Identical treatment leads to removal of a variable number of N-terminal amino acids in the case of PrPSc. This results in the appearance of three distinct bands, corresponding to di-, mono-, and un-glycosylated forms of PrP, upon Western Blotting. Several techniques have been developed to detect PrPSc in brain tissues, including Western Blot or other immunoblot methods.Citation22-Citation24 Quantitative Western Blot analysis revealed the highest expression of PrPC in cerebellum, obex, and spinal cord. Intermediate levels were detected in thymus, intestine, nervous, heart, and spleen; lower levels were found in lung, muscle, kidney, lymph node, skin, pancreas, and liver.Citation25 Western Blotting coupled with gel electrophoresis is one of the immunodetection methods successfully used for detection of PrPSc in tissue extracts.Citation26,Citation27 After denaturation of the tissue extract by heating with sodium dodecyl sulfate, the sample is analyzed by PAGE and the denatured protein is transferred to a solid support and detected with an enzyme-labeled antibody, often of goat, rabbit and mouse. The specificity of Western Blotting is based on the fact that proteolysis with proteinase K characteristically alters the molecular mass (app. five kDa) of the PrPc, due to the partial degradation of the N-terminal part of the protein.Citation28 Immunohistochemical analysis detected intense cellular-specific PrPC staining in neurons, thymocytes and lymphocytes. PrPC was also detected in the enteric wall, pancreatic islets of Langerhans, myocardium, pulmonary alveolar sacs, renal glomeruli, and dermal epithelial cells.Citation25
Another useful approach is the in situ detection of PrP by immunohistochemistry (IHC). In most IHC procedures, the brain tissue sections are treated to destroy PrPC with formic acid rather than with protease, since formic acid also enhances the PrPSc immunoreactivity.Citation29 A suitable IHC procedure was developed using brain tissue from hamsters that had been inoculated with the transmissible mink encephalopathy agent. Tissue samples were fixed in PLP (periodate, lysine, paraformaldehyde) that contained paraformaldehyde at a concentration of 0.125%. Before the application of IHC technique, tissue sections were deparaffinized and treated with formic acid simultaneously to enhance PrPSc immunoreactivity and to degrade PrPC. Primary antibody was obtained from a rabbit immunized to PrPSc extracted from brains of mice with experimentally induced scrapie. Brains from 21 sheeps with histopathologically confirmed scrapie were examined by IHC. In all of these brains, PrPSc was widely distributed throughout the entire brain.
In the case of human diseases, diagnosis is based almost exclusively on clinical examination. The disease is then considered as probable depending on the extent to which the clinical symptoms fit the standard guidelines. However, in the case of animals, detection of the only disease-specific analyte, PrPSc, can be commercially done.Citation30 From the clinical point of view, the most sensitive and specific method for diagnosing TSE is unquestionably experimental infection of laboratory animals. The animal is injected with a homogenate prepared from the suspicious tissue and the appearance of clinical signs is followed. The disease progress is then confirmed after dissection using classic techniques, such as histology, immunohistology, or Western Blot.Citation31 The animal infection is too laborious and time-consuming to be used for routine high-throughput screening.Citation28 Recently, new post-mortem tests have been introduced enabling rapid screening of the suspicious samples. Currently, 5 commercial tests are approved by the European Commission for BSE detection (Prionics-Check Western test, Enfer test, CEA/Biorad test, Prionics-Check LIA test, and Conformational-dependent immunoassay). All these tests are based on the immunodetection of the pathological PrPSc isoform, whereas four of them use proteolysis to distinguish PrPC from misfolded PrPSc 17. It has to be noted that none of these tests is able to identify infected animal at the pre-symptomatic stage and therefore the risk of the infectious agents entering the food chain is not completely eliminated.
Immunological detection of PrPSc in peripheral tissues
The infectivity studies have shown that prion proteins occurred in low amounts in the peripheral tissues, such as lymphoid organs and blood,Citation32 already at early stages of the disease, e.g. during the pre-symptomatic period. Some commercial assays (TeSeETM CJD ELISA and TeSeETM, ) are available to detect PrPSc in cerebral and lymphoid tissues of TSE patients. These two assays have been compared by Ugnon-Cafe et al.Citation50 using samples from 54 vCJD affected patients and 51 controls. Authors concluded that these tools were rapid and robust for routine in vitro human TSE diagnosis and characterization. CJD could also be diagnosed during the patient’s lifetime by detection of PrPSc in the tonsil. A pilot study was undertaken to look at the feasibility of testing for vCJD in deceased donors using tonsillar tissue. Obtaining tonsillar tissue in the immediate post-mortem period was limited by the presence of rigor mortis. Tonsillar tissue was suitable for routine analysis for the occurrence of prion protein associated with vCJD in the deceased tissue donors. In spite of the fact that palatine and lingual tonsil tissues could be obtained in pairs, it was possible, in the majority of cases, to set aside an intact sample for confirmatory testing if required.Citation51 A method using Western Blot assay with precipitation of streptomycin sulfate has been also reported improving the PrPSc detection sensitivity and providing the potential for specific, rapid and flexible determination of low PrPSc levels in the specimens not only from the central nervous system, but also from peripheral organs or fluids.Citation52
Table 1. Summary of Limits of Detection (LODs) for prion proteins measured by various methods
A new in vitro amplification technology, designated “real-time quaking-induced conversion (RT-QUIC),” has been described for the detection of tabnormal PrP (PrPSc) form in easily accessible specimens, such as cerebrospinal fluid. RT-QUIC method can be applied to other prion diseases, including scrapie, chronic wasting disease (CWD) and bovine spongiform encephalopathy (BSE), and is able to quantify PrP seeding activity when combined with an end-point dilution of samples.Citation34 Solid-state matrix can be used for capturing and concentrating disease-associated PrP. Coupling of this method with direct immunodetection of surface-bound material enabled to distinguish 10−10 dilution of exogenous vCJD prion-protein-infected brain from 10−6 dilution of normal brain (mean chemiluminescent signal, 1.3 × 105 for vCJD vs. 9.9 × 104 for normal brain; showing an assay sensitivity for vCJD of 71.4% and a specificity of 100%).Citation53
Non-immunological detection of PrPSc
In order to avoid the use of antibodies as mentioned in previous paragraph, several spectroscopic methods, such as multispectral UV fluoroscopy,Citation54 fluorescence correlation spectroscopy,Citation55,Citation56 magnetic resonance spectroscopyCitation57-Citation59 or Fourier-transformed infrared spectroscopy (FTIR)Citation60,Citation61 have been employed. The main disadvantages of these methods include requirements of expensive and sophisticated equipment as well as skilled operator. Recently, Raman scattering spectroscopy using gold nanorods 3D-supercrystals was used for PrP detection.Citation62 Last but not least, sensitive mass spectrometry based method of the PrP quantification in a variety of mammalian species has been presented.Citation36
On the other hand, protein misfolding cyclic amplification (PMCA) has a great potential and it is certainly the most promising approach from the viewpoint of a blood test development. It mimics pathological processes and is similar to the polymerase chain reaction; PrPSc is incubated in the presence of PrPC excess to initiate conversion to PrPSc aggregates which are subsequently dispersed by sonication to encourage the formation of new aggregates. The quantity of formed PrPSc depends on the number of expansion/sonication cycles performed.Citation63-Citation66 Detection limits are summarized in .
In vivo diagnostics
Neurodegenerative diseases can be definitively confirmed by post mortem histopathologic examination of senile plaques (SPs) and neurofibrillary tangles (NFTs) in the brain using stains and dyes that identify these microscopic structures. Advances in in vivo detection of β-amyloid formation and aggregation may further facilitate the drug development for the disease by providing critical information on plaque burden in the living brain. Currently, development of specific molecular imaging agents for direct mapping of Aβ aggregates in the living brain is a research topic being actively investigated. Several research groups have launched programs to successfully identify the biomarkers for imaging Aβ plaques in the brain.Citation67-Citation69
Even though computer tomography (CT) is not sensitive or specific in the evaluation of the disease, it may show atrophy at the advanced stage of the disease. Magnetic resonance imaging (MRI) is far more sensitive, especially with the addition of fluid attenuation inversion recovery (FLAIR) and diffusion weighted imaging (DWI). MRI of patient’s brain with prion diseases may show mild to moderate generalized atrophy at the early stage of disease and dramatic diffuse atrophy after a relatively short duration of disease; however, this finding is not consistent.Citation70
The detection of individual SPs and NFTs in vivo by positron-emission tomography (PET) or single-photon emission CT (SPECT) should improve diagnosis and also accelerate discovery of effective therapeutic agents. About a decade ago, the first successful Aβ plaque-specific PET imaging study was conducted in a living human subject clinically diagnosed with probable AD using the 11C-labeled radiopharmaceutical Pittsburgh Compound B (PiB; [11C]-2-4′-[methylaminophenyl]-6-hydroxybenzothiazole).Citation71 Since then many PET/SPECT imaging probes have been suggested, e.g. (18F)-2-(1-[2-{N-(2-fluoroethyl)-N-methylamino} naphthalene-6-yl]ethylidene)malononitrile (FDDNP), (11C)4-N-methylamino-4′-hydroxystilbene (SB-13),Citation72 (11C)2-(2-[2-dimethylaminothiazol-5-yl]ethenyl)-6-(2-[fluoro]ethoxy)benzoxazole (BF-227),Citation73 and (11C)-2-(6-[methylamino]pyridin-3-yl)-1,3-benzothiazol-6-ol (AZD2184).Citation74 It is clear that this type of PrP diagnostics related diseases has a great potential to be further developed and new imaging assays are still being looked for. Some of them could be based on quantum dots (QDs).
QDs as Alternative for Prion Detection
In the 1970s and early 1980s, understanding the photophysical properties of semiconductor structures was important for a broad range of computer and electronic applications. It was hypothesized that the physical properties of structures in an intermediate size range (between single atoms and bulk) could be tuned by alteration of size and shape. For electronics and computer applications, such a system allows to synthesize a large set of nanometer sized building blocks for constructing faster and smaller computer chips or more efficient light-emitting devices. It was soon realized that these nanometer sized structures called quantum dots (QDs) could have significantly much more applications. Nowadays a wide range of QDs utilizations are found in the area of biology, biochemistry and biomedicine. Besides the applications as simple sensors,Citation75-Citation77 the main function of QDs based on their exceptional fluorescent properties in the biochemical and biomedical research area is their usage as unique fluorescent labels.Citation78-Citation80 The utilization of QDs for various purposes is shown in .
Figure 2. Applications of QDs in chemical, biomedical and diagnostic area. QDs (the large green crystal) can be applied for biosensing, biomarker mapping, real time cell growth monitoring, drug delivering, gene delivering, detection and diagnostics, molecular imaging, and targeted therapy.
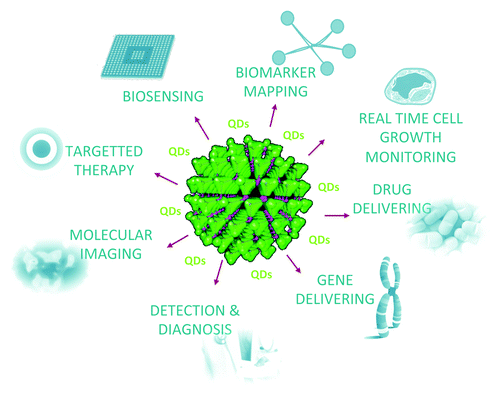
The most important characterization of QDs dealing with their optical properties is usually provided by UV-VIS and photoluminescence spectroscopy which offer fast, non-destructive and contactless option. Besides monitoring the excitation, absorption and emission spectra which are usually applied for the calculation of quantum yield, band gap studies can be also performed by optical diffuse reflectance spectra measurement. The size of QDs can be calculated from absorption edges using Henglein empirical curve, which relates the wavelength of the absorption threshold to the diameter of QDs.Citation81 In particular cases, FTIR spectroscopic measurements are also necessary.Citation82 Raman spectroscopy, as one of the best non-destructive techniques, can be employed for QDs characterization as well, since it allows to probe the active optical phonon modes and to explore the confined electronic structure of QDs.Citation83,Citation84
As mentioned before, the optical properties (fluorescence emission) of QDs can be fine-tuned by the QDs size which is a key parameter that determines the spectral position and purity of photoluminescence. QDs size and morphology (shape and structure) are generally calculated using conventional techniques like scanning electron microscopy (SEM), transmission electron microscopy (TEM), and dynamic light scattering (DLS) studies. For these measurements, QDs are usually transformed to powder form either by simple drying of QDs solution or by precipitation (e.g. with ethanol), centrifugation and final drying. Besides these techniques, field flow fractionation, which belongs to high resolution liquid chromatography-like elution methods for separating and sizing, can be also successfully employed for QDs size distribution analysis.Citation85 The structure of QDs is usually analyzed by X-ray diffraction (XRD)Citation86 and the elemental composition of QDs can be studied by energy dispersive X-ray analysis (known as EDS, EDX, or EDAX).Citation82 Other techniques, like inductively coupled plasma atomic emission spectrometry (ICP-AES), were used for analysis of QDs as well, namely for the metal ions content in QDs.Citation87 Recently, electrochemical methods were also applied to study the QDs behavior.Citation88-Citation90
Biocompatibility
To use QDs in biology, it is extremely important to deal with the biocompatibility and toxicity. However, minimum studies have examined the toxicity of these nanomaterials.Citation91-Citation93 Moreover, their toxicity evaluation is highly complicated, due to the diversity of materials. In sharp contrast to conventional hazardous materials, the attention has to be paid to the nanoparticle-specific problems, including the fact that the surface of nanomaterials is very active due to the large surface area and surface-to-volume ratio. In addition, it is necessary to exclude the effect of solubility and possible contamination which could also decrease the validity of any toxicity testing.Citation92 In 2006, Hardman reviewed the toxicity of QDs as a function of physicochemical and environmental factors.Citation94 QDs size, charge, concentration, outer coating bioactivity (capping material, functional groups) as well as oxidative, photolytic, and mechanical stability have each been shown as determining factors of QDs toxicity.
To improve the biocompatibility, passivation or capping of QDs with a layer of ZnS or CdS is needed. ZnS or CdS improve the fluorescence quantum yield of the QDs and protect them against photo-oxidation, which is important for minimizing cytotoxicity and enhancing photostability. ZnS shell has larger band gap energy than CdSe, eliminating the core’s surface defect states. ZnS shell has also similar bond length to CdSe, minimizing the crystal-lattice strain and allowing the epitaxial growth. Even with advances in synthesis, obtaining biomedically useful QDs is still problematic due to differences in optical properties from batch to batch. From one synthesis to the next, QDs with different quantum yields and fluorescence spectra may be produced. Moreover, further functionalization is needed for incorporation of required chemical species.
Various surface modification techniques were developed to ensure the specific bio-conjugation (). This is usually achieved by modifying QDs with proteins, peptides, nucleic acids, or other biomolecules that mediate specific interactions with living systems. Surface engineering is thus crucial not only for tuning the fundamental properties of nanomaterials and for rendering them stable and soluble in different environments, but also for creating nanoparticle–biomolecule hybrids capable of participating in biological processes. Such hybrids should combine useful properties of both materials involved, i.e. the optical properties of nanocrystals and biological functions of ligands attached.Citation78 One of these strategies utilizes the biotin-avidin (respectively streptavidin and neutravidin) interaction known for its very high specificity. Modification of QDs by the streptavidin proved a very successful method evaluated in numerous publications.Citation78,Citation95-Citation98 Due to this success, streptavidin-QDs are nowadays also commercially available. In addition, biotin-functionalized QDs were developed to exploit the same interaction ().
Figure 3. (A) Differential pulse voltammograms of prion protein (PrP, 500 µg/mL) with additions of QDs in various volumes (460 µg/mL, quantified according to concentration of cadmium[II]). Electrochemical measurements were performed with AUTOLAB Analyzer (EcoChemie) connected to VA-Stand 663 (Metrohm), using a standard cell with three electrodes. The working electrode was a hanging mercury drop electrode (HMDE) with a drop area of 0.4 mm2. The reference electrode was an Ag/AgCl/3M KCl electrode and the auxiliary electrode was a graphite electrode. Acetate buffer (0.2 M, pH 5) was used as the supporting electrolyte. For smoothing and baseline correction the software GPES 4.9 supplied by EcoChemie was employed. The amount of QDs was measured using DPV. Differential pulse voltammetric measurements were performed under the following parameters: start potential -1.5 V; end potential 0 V; modulation time 0.057 s, time interval 0.2 s, step potential of 1.05 mV/s, modulation amplitude of 250 mV, Eads = 0 V. All experiments were performed at room temperature (20 °C). The DPV samples analyzed were deoxygenated prior to measurements by purging with argon (99.999%) saturated with water for 120 s. (B) In vivo images of 10 µl (460 µg/mL) of CdTe QDs with prion protein (PrP, 500 µg/mL) applied 1 cm under skin of chicken thigh. The image of chicken leg without any injection was subtracted. The fluorescence imaging was performed by In vivo Xtreme system by Carestream. This instrument is equipped with 400 W xenon light source and 28 excitation filters (410–760 nm). The emitted light is captured by 4MP CCD camera. In this experiment, 410 nm was used as an excitation wavelength and the emission was measured at 535 nm. The exposition time was 5 s. The other parameters were set as follows: Bin, 2 × 2; field of view, 12 cm; fStop, 1.1. (C) Scheme of complex of QDs and antibody with prion protein and the possible using of such prion protein sensing in brain tissue.
![Figure 3. (A) Differential pulse voltammograms of prion protein (PrP, 500 µg/mL) with additions of QDs in various volumes (460 µg/mL, quantified according to concentration of cadmium[II]). Electrochemical measurements were performed with AUTOLAB Analyzer (EcoChemie) connected to VA-Stand 663 (Metrohm), using a standard cell with three electrodes. The working electrode was a hanging mercury drop electrode (HMDE) with a drop area of 0.4 mm2. The reference electrode was an Ag/AgCl/3M KCl electrode and the auxiliary electrode was a graphite electrode. Acetate buffer (0.2 M, pH 5) was used as the supporting electrolyte. For smoothing and baseline correction the software GPES 4.9 supplied by EcoChemie was employed. The amount of QDs was measured using DPV. Differential pulse voltammetric measurements were performed under the following parameters: start potential -1.5 V; end potential 0 V; modulation time 0.057 s, time interval 0.2 s, step potential of 1.05 mV/s, modulation amplitude of 250 mV, Eads = 0 V. All experiments were performed at room temperature (20 °C). The DPV samples analyzed were deoxygenated prior to measurements by purging with argon (99.999%) saturated with water for 120 s. (B) In vivo images of 10 µl (460 µg/mL) of CdTe QDs with prion protein (PrP, 500 µg/mL) applied 1 cm under skin of chicken thigh. The image of chicken leg without any injection was subtracted. The fluorescence imaging was performed by In vivo Xtreme system by Carestream. This instrument is equipped with 400 W xenon light source and 28 excitation filters (410–760 nm). The emitted light is captured by 4MP CCD camera. In this experiment, 410 nm was used as an excitation wavelength and the emission was measured at 535 nm. The exposition time was 5 s. The other parameters were set as follows: Bin, 2 × 2; field of view, 12 cm; fStop, 1.1. (C) Scheme of complex of QDs and antibody with prion protein and the possible using of such prion protein sensing in brain tissue.](/cms/asset/63d9a8df-1069-4606-88ee-b4f083fcde28/kprn_a_10926524_f0003.gif)
In vitro applications
The conditions of in vitro experiments are artificial and simulate what might happen in vivo. In the last decade, surface engineering and biofunctionalization techniques have transformed semiconductor nanocrystals into complex cellular probes capable of interaction with biomolecules and direct participation in biological processes. In 1998, two seminal scientific papers demonstrated for the first time that semiconductor nanoparticles could be made water-soluble and used as biological imaging probes.Citation99,Citation100 One approach utilized encapsulation of QDs in silica shell in order to produce QDs for a single-excitation dual-color cell staining.Citation99 When derived with trimethoxysilylpropyl urea and acetate groups, green QDs preferentially labeled the cell nucleus, and when modified with biotin, red QDs labeled F-actin filaments pretreated with phalloidin-biotin and streptavidin. The second paper was the first to demonstrate the ligand-exchange approach to QDs water solubilization.Citation100 Subsequent conjugation of transferrin produced QDs probes that were endocytosed by live HeLa cells, resulting in the punctate cell staining, while IgG bioconjugates were used in an aggregation-based immunoassay. Since then, many surface engineering techniques for QDs solubilization and biofunctionalization have been developed, enabling the application of QDs probes with specific design. Such probes have found their use in a variety of in vitro applications, such as histological evaluation of cells and tissue specimens, single molecule detection and real-time tracking, long-term live-cell imaging, and study of intracellular processes. Moreover, QDs can be employed as optical labels that probe dynamic biological processes, such as biocatalysed reactions or structurally induced biomolecular transformations using fluorescence resonance energy transfer (FRET) or electron-transfer quenching as photophysical probing mechanisms ().
Recent achievements in merging nanoparticle encapsulation and bioconjugation steps and design of pre-functionalized surface coatings promise to provide more compact, stable, and biocompatible nanoparticles with controlled density and orientation of ligands attached. Amphiphilic polymers with a maleic anhydride backbone are being actively explored for this purpose. In organic anhydrous solvents, such polymers encapsulate trioctylphosphine-coated QDs and introduce reactive anhydride groups on the surface. In basic aqueous buffers, anhydride rings are quickly hydrolyzed, yielding negatively charged carboxylic acid groups and rendering QDs water soluble.Citation101 More importantly, anhydride groups are highly reactive toward amine-containing molecules, thus allowing covalent conjugation of various biomolecules to the polymer chains without the need for post-encapsulation modification.Citation102,Citation103
The choice of bioconjugation approach depends on the availability of ligands with suitable functional groups and on specific application requirements. However, common design criteria involve preserved QDs photo-physical properties and ligand biofunctionality, controlled ligand orientation and binding stoichiometry, compact probe size, and good stability in physiological environment. As these criteria can be satisfied in only few specific cases, improvement of existing bioconjugation techniques and design of novel application specific water solubilization and bioconjugation approaches remain an active area of research. With the development of stable and biofunctional QDs probes, these materials will become the nanoscience building blocks with flexible properties that could be further optimized for specific applications, including biomedical imaging, detection, and nano-therapeutics.Citation104
Electrochemical detection as a sensitive technique in combination with QDs has been already used for numerous applications. However, QDs have not been sufficiently analyzed by voltammetry with respect to employment these easy to use and repeatable methods for QDs characterization and quantification. Therefore, CdTe QDs were characterized using cyclic and differential pulse voltammetry. The obtained peaks were identified and detection limit (3 S/N) was estimated down to 100 fg/mL. Based on convincing results, new way how to study the stability and quantify QDs was suggested. The approach was further utilized for testing of QDs stability.Citation5 Interaction of PrP with CdTe QDs by voltammetry seems to be a new and extremely sensitive tool for sensing of these proteins. Primarily, fluorescent and electrochemical properties of QDs were characterized. Further, electrochemical study of their interactions was performed to find the most suitable conditions for sensitive detection of PrP (). Detection limit (3 S/N) was estimated as 1 fg in 5 µL.Citation105 This makes labeling of proteins with QDs of great importance due to easy applicability and possibility to use in miniaturized devices. Based on our results, it can be concluded that QDs-PrP complex is stable and can be quantified in extremely low amounts. The stability of complex in vivo was also proven (). This should open new possibilities how to determine the presence of these proteins in a tissue (), on surgical equipment and on other types of materials which could be contagious ().
Figure 4. Sensing of prion protein complex with quantum dots on the surface of a scalpel. (A) photo of a scalpel, (B) fluorescent image of scalpel, (C) fluorescent image of scalpel with prion protein-QDs complex, and (D) detail of fluorescent image of scalpel with prion protein-QDs complex. QD were prepared according to Duan et al.Citation106 Cadmium chloride solution (CdCl2, 0.04 M, 4 ml) was diluted to 42 ml with ultrapure water, and then trisodium citrate dihydrate (100 mg), Na2TeO3 (0.01 M, 4 ml), MPA (119 mg), and NaBH4 (50 mg) were added successively under magnetic stirring. The molar ratio of Cd2+/MPA/Te was 1:7:0.25. Ten ml of the resulting CdTe precursor was put into a Teflon vessel. CdTe QDs were prepared at 95 °C for various times according to desired color (10 min, green; 30 min, yellow; 120 min, red) under microwave irradiation (400 W, Multiwave3000, Anton-Paar GmbH). After microwave irradiation, the mixture was cooled to 50 °C and the CdTe QDs sample was obtained. Purification of CdTe QDs was performed using isopropanol condensing. The CdTe QDs was mixed with isopropanol in ratio 1:2 and then centrifuged for 10 min at 25 000 rpm (Eppendorf centrifuge 5417R). Precipitate (pure CdTe QDs) was than resuspended to initial volume of Tris Buffer pH 8.5. The fluorescence imaging was performed by In vivo Xtreme system by Carestream. This instrument is equipped with 400 W xenon light source and 28 excitation filters (410–760 nm). The emitted light is captured by 4MP CCD camera. In this experiment, 410 nm was used as an excitation wavelength and the emission was measured at 535 nm. The exposition time was 5 s. The other parameters were set as follows: Bin, 2 × 2; field of view, 12 cm; fStop, 1.1.
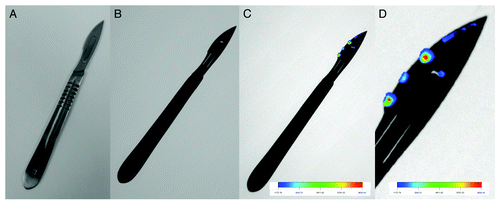
In vivo applications
Even though some attempts for optical imaging of prion based diseases have been made, these were mostly in in vitro mode.Citation107,Citation108 A new and exciting direction of research using QDs is their application as contrast agents for in vivo imaging.Citation109-Citation112 The characterization and analysis of biomolecules and biological systems in the context of intact organisms is known as in vivo research. The in vivo approach involves experiments performed in the large system of an experimental animal body. Organic fluorophores and chemiluminescence probes are currently the most commonly used optical probes for animal imaging. However, the lack of available probes that emit in NIR emission range (>650 nm) cause a significant limitation of these optical contrast agents. The NIR emitting window is appealing for biological optical imaging because of low tissue absorption and scattering effects in this emission range. The range of NIR optical window for animal imaging is typically set at 650–900 nm. Since the optical properties of QDs can be tuned by size and composition, it is possible to prepare series of NIR-emitting QDs for animal imaging.Citation113 CdTe, CdTeSe, InPAs, PbS, and PbSe have been successfully synthesized with NIR emission.Citation114,Citation115 For most in vivo imaging investigations , QDs are usually directly injected into the living animal intravenously or subcutaneously and thereby are delivered into the bloodstream.
Future Prospects
QDs, tiny light-emitting nanocrystals, have emerged as a new promising class of fluorescent probes for biomolecular and cellular imaging. In comparison with organic dyes and fluorescent proteins, QDs possess unique optical and electronic properties such as size-tunable light emission, improved signal brightness, resistance against photobleaching, and simultaneous excitation of multiple fluorescence colors. The biomedical applications of nanoparticles are rooted in the advanced functional design and have been realized in preclinical experimental diagnosis. As compared with the imaging in vitro, QDs imaging in vivo faces different challenges, caused by the increase of complexity of multicellular organism. There are four main kinds of in vivo imaging applications with QDs: (1) biodistribution of QDs, (2) vascular imaging, (3) tracking of QDs, and (4) tumor imaging (). Utilization of nanomaterials, particularly nanoparticles for the in vivo monitoring of brain diseases is one of near future appealing applications.Citation116 The incorporation of contrast agents into the cells can be done by either phagocytosis or conjugation of the contrast agent to the cell surface via antibody interaction with the receptor. In vivo imaging of amyloid plaques may be useful for the evaluation and diagnosis of AD patients ().
Disclosure of Potential Conflicts of Interest
No potential conflicts of interest were disclosed.
Acknowledgments
Financial support from CEITEC CZ.1.05/1.1.00/02.0068, DOC CEITEC.01/2012, Grant Agency of the Czech Republic under the contract GACR 102/13-20303P and IGA TP1/2013 (EA 3b) is highly acknowledged. The author Sobrova P is “Holder of Brno PhD Talent Financial Aid”.
References
- Prusiner SB. Prions. Proc Natl Acad Sci U S A 1998; 95:13363 - 83; http://dx.doi.org/10.1073/pnas.95.23.13363; PMID: 9811807
- Hegde RS, Tremblay P, Groth D, DeArmond SJ, Prusiner SB, Lingappa VR. Transmissible and genetic prion diseases share a common pathway of neurodegeneration. Nature 1999; 402:822 - 6; http://dx.doi.org/10.1038/45574; PMID: 10617204
- Prusiner SB. Prion diseases and the BSE crisis. Science 1997; 278:245 - 51; http://dx.doi.org/10.1126/science.278.5336.245; PMID: 9323196
- Inge-Vechtomov SG, Zhouravleva GA, Chernoff YO. Biological roles of prion domains. Prion 2007; 1:228 - 35; http://dx.doi.org/10.4161/pri.1.4.5059; PMID: 19172114
- Sobrova P, Ryvolova M, Hynek D, Adam V, Hubalek J, Kizek R. Electrochemical Behaviour of Native and Denatured beta-Sheet Breaker Prion Protein. Int J Electrochem Sci 2012; 7:928 - 42
- Kozlowski H, Luczkowski M, Remelli M, Valensin D. Copper, zinc and iron in neurodegenerative diseases (Alzheimer's, Parkinson's and prion diseases). Coord Chem Rev 2012; 256:2129 - 41; http://dx.doi.org/10.1016/j.ccr.2012.03.013
- Viles JH. Metal ions and amyloid fiber formation in neurodegenerative diseases. Copper, zinc and iron in Alzheimer's, Parkinson's and prion diseases. Coord Chem Rev 2012; 256:2271 - 84; http://dx.doi.org/10.1016/j.ccr.2012.05.003
- Kessels HW, Nguyen LN, Nabavi S, Malinow R. The prion protein as a receptor for amyloid-beta. Nature 2010; 466:E3 - 4, discussion E4-5; http://dx.doi.org/10.1038/nature09217; PMID: 20703260
- Laurén J, Gimbel DA, Nygaard HB, Gilbert JW, Strittmatter SM. Cellular prion protein mediates impairment of synaptic plasticity by amyloid-beta oligomers. Nature 2009; 457:1128 - 32; http://dx.doi.org/10.1038/nature07761; PMID: 19242475
- Fowler DM, Kelly JW. Functional amyloidogenesis and cytotoxicity-insights into biology and pathology. PLoS Biol 2012; 10:e1001459; http://dx.doi.org/10.1371/journal.pbio.1001459; PMID: 23300381
- Hainfellner JA, Wanschitz J, Jellinger K, Liberski PP, Gullotta F, Budka H. Coexistence of Alzheimer-type neuropathology in Creutzfeldt-Jakob disease. Acta Neuropathol 1998; 96:116 - 22; http://dx.doi.org/10.1007/s004010050870; PMID: 9705125
- Peden AH, Ironside JW. Molecular pathology in neurodegenerative diseases. Curr Drug Targets 2012; 13:1548 - 59; http://dx.doi.org/10.2174/138945012803530134; PMID: 22974397
- van Harten AC, Kester MI, Visser PJ, Blankenstein MA, Pijnenburg YAL, van der Flier WM, Scheltens P. Tau and p-tau as CSF biomarkers in dementia: a meta-analysis. Clin Chem Lab Med 2011; 49:353 - 66; http://dx.doi.org/10.1515/CCLM.2011.086; PMID: 21342021
- Jellinger KA. Interaction between pathogenic proteins in neurodegenerative disorders. J Cell Mol Med 2012; 16:1166 - 83; http://dx.doi.org/10.1111/j.1582-4934.2011.01507.x; PMID: 22176890
- Revesz T, Ghiso J, Lashley T, Plant G, Rostagno A, Frangione B, Holton JL. Cerebral amyloid angiopathies: a pathologic, biochemical, and genetic view. J Neuropathol Exp Neurol 2003; 62:885 - 98; PMID: 14533778
- Kellett KAB, Hooper NM. Prion protein and Alzheimer disease. Prion 2009; 3:190 - 4; http://dx.doi.org/10.4161/pri.3.4.9980; PMID: 19887909
- Parchi P, Strammiello R, Giese A, Kretzschmar H. Phenotypic variability of sporadic human prion disease and its molecular basis: past, present, and future. Acta Neuropathol 2011; 121:91 - 112; http://dx.doi.org/10.1007/s00401-010-0779-6; PMID: 21107851
- Soto C. Diagnosing prion diseases: needs, challenges and hopes. Nat Rev Microbiol 2004; 2:809 - 19; http://dx.doi.org/10.1038/nrmicro1003; PMID: 15378045
- Huzarewich RL, Siemens CG, Booth SA. Application of “omics” to prion biomarker discovery. J Biomed Biotechnol 2010; 2010:613504; http://dx.doi.org/10.1155/2010/613504; PMID: 20224650
- Goldfarb LG, Cervenakova L, Gajdusek DC. Genetic studies in relation to kuru: an overview. Curr Mol Med 2004; 4:375 - 84; http://dx.doi.org/10.2174/1566524043360627; PMID: 15354868
- Liberski PP. Kuru and D. Carleton Gajdusek: a close encounter. Folia Neuropathol 2009; 47:114 - 37; PMID: 19618335
- Brown DR, Wong BS, Hafiz F, Clive C, Haswell SJ, Jones IM. Normal prion protein has an activity like that of superoxide dismutase. Biochem J 1999; 344:1 - 5; http://dx.doi.org/10.1042/0264-6021:3440001; PMID: 10548526
- Safar J, Wille H, Itri V, Groth D, Serban H, Torchia M, Cohen FE, Prusiner SB. Eight prion strains have PrP(Sc) molecules with different conformations. Nat Med 1998; 4:1157 - 65; http://dx.doi.org/10.1038/2654; PMID: 9771749
- Wadsworth JDF, Joiner S, Hill AF, Campbell TA, Desbruslais M, Luthert PJ, Collinge J. Tissue distribution of protease resistant prion protein in variant Creutzfeldt-Jakob disease using a highly sensitive immunoblotting assay. Lancet 2001; 358:171 - 80; http://dx.doi.org/10.1016/S0140-6736(01)05403-4; PMID: 11476832
- Peralta OA, Eyestone WH. Quantitative and qualitative analysis of cellular prion protein (PrP(C)) expression in bovine somatic tissues. Prion 2009; 3:161 - 70; http://dx.doi.org/10.4161/pri.3.3.9772; PMID: 19806026
- Nunnally BK. It's a mad, mad, mad, mad cow: a review of analytical methodology for detecting BSE/TSE. Trac-Trends Anal Chem 2002; 21:82 - 9; http://dx.doi.org/10.1016/S0165-9936(01)00134-0
- Aguzzi A, Heikenwalder M, Miele G. Progress and problems in the biology, diagnostics, and therapeutics of prion diseases. J Clin Invest 2004; 114:153 - 60; PMID: 15254579
- Grassi J, Maillet S, Simon S, Morel N. Progress and limits of TSE diagnostic tools. Vet Res 2008; 39:33; http://dx.doi.org/10.1051/vetres:2008009; PMID: 18284910
- Miller JM, Jenny AL, Taylor WD, Marsh RF, Rubenstein R, Race RE. Immunohistochemical detection of prion protein in sheep with scrapie. J Vet Diagn Invest 1993; 5:309 - 16; http://dx.doi.org/10.1177/104063879300500301; PMID: 8104039
- Zsolnai A, Anton I, Kühn C, Fésüs L. Detection of single-nucleotide polymorphisms coding for three ovine prion protein variants by primer extension assay and capillary electrophoresis. Electrophoresis 2003; 24:634 - 8; http://dx.doi.org/10.1002/elps.200390074; PMID: 12601731
- Groschup MH, Weiland F, Straub OC. Diagnosis of bovine spongiform encephalopathy and scrapie - Possibilities and limitations of the diagnosis. Tierarztl Umsch 1994; 49:137 - 42
- Lacroux C, Bougard D, Litaise C, Simmons H, Corbiere F, Dernis D, Tardivel R, Morel N, Simon S, Lugan S, et al. Impact of leucocyte depletion and prion reduction filters on TSE blood borne transmission. PLoS One 2012; 7:e42019; http://dx.doi.org/10.1371/journal.pone.0042019; PMID: 22860049
- Zhuang HL, Zhen SJ, Wang J, Huang CZ. Sensitive detection of prion protein through long range resonance energy transfer between graphene oxide and molecular aptamer beacon. Anal Methods 2013; 5:208 - 12; http://dx.doi.org/10.1039/c2ay26156a
- Atarashi R, Sano K, Satoh K, Nishida N. Real-time quaking-induced conversion: a highly sensitive assay for prion detection. Prion 2011; 5:150 - 3; http://dx.doi.org/10.4161/pri.5.3.16893; PMID: 21778820
- Azam G, Shibata T, Kabashima T, Kai M. Sensitive chemiluminescence detection of prion protein on a membrane by using a peroxidase-labeled dextran probe. Anal Sci 2011; 27:715 - 20; http://dx.doi.org/10.2116/analsci.27.715; PMID: 21747179
- Silva CJ, Onisko BC, Dynin I, Erickson ML, Requena JR, Carter JM. Utility of mass spectrometry in the diagnosis of prion diseases. Anal Chem 2011; 83:1609 - 15; http://dx.doi.org/10.1021/ac102527w; PMID: 21288014
- Onisko B, Dynin I, Requena JR, Silva CJ, Erickson M, Carter JM. Mass spectrometric detection of attomole amounts of the prion protein by nanoLC/MS/MS. J Am Soc Mass Spectrom 2007; 18:1070 - 9; http://dx.doi.org/10.1016/j.jasms.2007.03.009; PMID: 17446085
- Yang WC, Schmerr MJ, Jackman R, Bodemer W, Yeung ES. Capillary electrophoresis-based noncompetitive immunoassay for the prion protein using fluorescein-labeled protein A as a fluorescent probe. Anal Chem 2005; 77:4489 - 94; http://dx.doi.org/10.1021/ac050231u; PMID: 16013864
- Yang WC, Yeung ES, Schmerr MJ. Detection of prion protein using a capillary electrophoresis-based competitive immunoassay with laser-induced fluorescence detection and cyclodextrin-aided separation. Electrophoresis 2005; 26:1751 - 9; http://dx.doi.org/10.1002/elps.200410202; PMID: 15815999
- Chang BG, Gray P, Piltch M, Bulgin MS, Sorensen-Melson S, Miller MW, Davies P, Brown DR, Coughlin DR, Rubenstein R. Surround optical fiber immunoassay (SOFIA): an ultra-sensitive assay for prion protein detection. J Virol Methods 2009; 159:15 - 22; http://dx.doi.org/10.1016/j.jviromet.2009.02.019; PMID: 19442839
- Reuter T, Gilroyed BH, Alexander TW, Mitchell G, Balachandran A, Czub S, McAllister TA. Prion protein detection via direct immuno-quantitative real-time PCR. J Microbiol Methods 2009; 78:307 - 11; http://dx.doi.org/10.1016/j.mimet.2009.07.001; PMID: 19596031
- Dabaghian RH, Barnard G, McConnell I, Clewley JP. An immunoassay for the pathological form of the prion protein based on denaturation and time resolved fluorometry. J Virol Methods 2006; 132:85 - 91; http://dx.doi.org/10.1016/j.jviromet.2005.09.002; PMID: 16219367
- Brooks B, Brooks A, Wulff SS, Lewis RV. Identification of problems developing an ultrasensitive immunoassay for the ante mortem detection of the infectious isoform of the CWD-associated prion protein. J Immunoassay Immunochem 2009; 30:135 - 49; http://dx.doi.org/10.1080/15321810902782848; PMID: 19330640
- Colby DW, Zhang Q, Wang S, Groth D, Legname G, Riesner D, Prusiner SB. Prion detection by an amyloid seeding assay. Proc Natl Acad Sci U S A 2007; 104:20914 - 9; http://dx.doi.org/10.1073/pnas.0710152105; PMID: 18096717
- Chang BG, Cheng X, Yin SM, Pan T, Zhang HT, Wong PK, Kang SC, Xiao F, Yan HM, Li CY, et al. Test for detection of disease-associated prion aggregate in the blood of infected but asymptomatic animals. Clin Vaccine Immunol 2007; 14:36 - 43; http://dx.doi.org/10.1128/CVI.00341-06; PMID: 17079434
- Trieschmann L, Navarrete Santos A, Kaschig K, Torkler S, Maas E, Schätzl H, Böhm G. Ultra-sensitive detection of prion protein fibrils by flow cytometry in blood from cattle affected with bovine spongiform encephalopathy. BMC Biotechnol 2005; 5:26; http://dx.doi.org/10.1186/1472-6750-5-26; PMID: 16202155
- Xiao SJ, Hu PP, Li YF, Huang CZ, Huang T, Xiao GF. Aptamer-mediated turn-on fluorescence assay for prion protein based on guanine quenched fluophor. Talanta 2009; 79:1283 - 6; http://dx.doi.org/10.1016/j.talanta.2009.05.040; PMID: 19635360
- Henry J, Anand A, Chowdhury M, Coté G, Moreira R, Good T. Development of a nanoparticle-based surface-modified fluorescence assay for the detection of prion proteins. Anal Biochem 2004; 334:1 - 8; http://dx.doi.org/10.1016/j.ab.2004.07.008; PMID: 15464948
- Huang XX, Long YJ, Zhang HJ, Wang QL, Zhu R, Zheng HZ. Gold nanoparticles as a probe for prion determination via resonance light scattering method. Anal Sci 2012; 28:475 - 9; http://dx.doi.org/10.2116/analsci.28.475; PMID: 22687927
- Ugnon-Café S, Dorey A, Bilheude JM, Streichenberger N, Viennet G, Meyronet D, Maues de Paula A, Perret-Liaudet A, Quadrio I. Rapid screening and confirmatory methods for biochemical diagnosis of human prion disease. J Virol Methods 2011; 175:216 - 23; http://dx.doi.org/10.1016/j.jviromet.2011.05.016; PMID: 21619894
- Warwick RM, Armitage WJ, Chandrasekar A, Mallinson G, Poniatowski S, Clarkson A. A pilot to examine the logistical and feasibility issues in testing deceased tissue donors for vCJD using tonsil as the analyte. Cell Tissue Bank 2012; 13:53 - 61; http://dx.doi.org/10.1007/s10561-010-9228-y; PMID: 21046259
- Dong CF, Huang YX, An R, Chen JM, Wang XF, Shan B, Lei YJ, Han L, Zhang BY, Han J, et al. Sensitive detection of PrPSc by Western blot assay based on streptomycin sulphate precipitation. Zoonoses Public Health 2007; 54:328 - 36; http://dx.doi.org/10.1111/j.1863-2378.2007.01062.x; PMID: 17894644
- Edgeworth JA, Farmer M, Sicilia A, Tavares P, Beck J, Campbell T, Lowe J, Mead S, Rudge P, Collinge J, et al. Detection of prion infection in variant Creutzfeldt-Jakob disease: a blood-based assay. Lancet 2011; 377:487 - 93; http://dx.doi.org/10.1016/S0140-6736(10)62308-2; PMID: 21295339
- Rubenstein R, Gray PC, Wehlburg CM, Wagner JS, Tisone GC. Detection and discrimination of PrPSc by multi-spectral ultraviolet fluorescence. Biochem Biophys Res Commun 1998; 246:100 - 6; http://dx.doi.org/10.1006/bbrc.1998.8542; PMID: 9600075
- Birkmann E, Schäfer O, Weinmann N, Dumpitak C, Beekes M, Jackman R, Thorne L, Riesner D. Detection of prion particles in samples of BSE and scrapie by fluorescence correlation spectroscopy without proteinase K digestion. Biol Chem 2006; 387:95 - 102; http://dx.doi.org/10.1515/BC.2006.013; PMID: 16497169
- Fujii F, Horiuchi M, Ueno M, Sakata H, Nagao I, Tamura M, Kinjo M. Detection of prion protein immune complex for bovine spongiform encephalopathy diagnosis using fluorescence correlation spectroscopy and fluorescence cross-correlation spectroscopy. Anal Biochem 2007; 370:131 - 41; http://dx.doi.org/10.1016/j.ab.2007.07.018; PMID: 17825783
- Lodi R, Parchi P, Tonon C, Manners D, Capellari S, Strammiello R, Rinaldi R, Testa C, Malucelli E, Mostacci B, et al. Magnetic resonance diagnostic markers in clinically sporadic prion disease: a combined brain magnetic resonance imaging and spectroscopy study. Brain 2009; 132:2669 - 79; http://dx.doi.org/10.1093/brain/awp210; PMID: 19755520
- Galanaud D, Haik S, Linguraru MG, Ranjeva JP, Faucheux B, Kaphan E, Ayache N, Chiras J, Cozzone P, Dormont D, et al. Combined diffusion imaging and MR spectroscopy in the diagnosis of human prion diseases. AJNR Am J Neuroradiol 2010; 31:1311 - 8; http://dx.doi.org/10.3174/ajnr.A2069; PMID: 20430851
- Murray KL, Knight RSG, Summers D, Collie DAC, Will RG. The role of MRI brain scan in the diagnosis of human prion disease. J Neurol Neurosurg Psychiatry 2005; 76:608
- Lasch P, Schmitt J, Beekes M, Udelhoven T, Eiden M, Fabian H, Petrich W, Naumann D. Antemortem identification of bovine spongiform encephalopathy from serum using infrared spectroscopy. Anal Chem 2003; 75:6673 - 8; http://dx.doi.org/10.1021/ac030259a; PMID: 14640744
- Beekes M, Lasch P, Naumann D. Analytical applications of Fourier transform-infrared (FT-IR) spectroscopy in microbiology and prion research. Vet Microbiol 2007; 123:305 - 19; http://dx.doi.org/10.1016/j.vetmic.2007.04.010; PMID: 17540519
- Alvarez-Puebla RA, Agarwal A, Manna P, Khanal BP, Aldeanueva-Potel P, Carbó-Argibay E, Pazos-Pérez N, Vigderman L, Zubarev ER, Kotov NA, et al. Gold nanorods 3D-supercrystals as surface enhanced Raman scattering spectroscopy substrates for the rapid detection of scrambled prions. Proc Natl Acad Sci U S A 2011; 108:8157 - 61; http://dx.doi.org/10.1073/pnas.1016530108; PMID: 21536908
- Castilla J, Saa P, Morales R, Abid K, Maundrell K, Soto C. Protein misfolding cyclic amplification for diagnosis and prion propagation studies. In: Kheterpal I, Wetzel R, eds. Amyloid, Prions, and Other Protein Aggregates, Pt B. San Diego: Elsevier Academic Press Inc, 2006:3-21.
- Saá P, Castilla J, Soto C. Ultra-efficient replication of infectious prions by automated protein misfolding cyclic amplification. J Biol Chem 2006; 281:35245 - 52; http://dx.doi.org/10.1074/jbc.M603964200; PMID: 16982620
- Saborio GP, Permanne B, Soto C. Sensitive detection of pathological prion protein by cyclic amplification of protein misfolding. Nature 2001; 411:810 - 3; http://dx.doi.org/10.1038/35081095; PMID: 11459061
- Soto C, Anderes L, Suardi S, Cardone F, Castilla J, Frossard MJ, Peano S, Saa P, Limido L, Carbonatto M, et al. Pre-symptomatic detection of prions by cyclic amplification of protein misfolding. FEBS Lett 2005; 579:638 - 42; http://dx.doi.org/10.1016/j.febslet.2004.12.035; PMID: 15670821
- Agdeppa ED, Kepe V, Liu J, Flores-Torres S, Satyamurthy N, Petric A, Cole GM, Small GW, Huang SC, Barrio JR. Binding characteristics of radiofluorinated 6-dialkylamino-2-naphthylethylidene derivatives as positron emission tomography imaging probes for beta-amyloid plaques in Alzheimer’s disease. J Neurosci 2001; 21:RC189; PMID: 11734604
- Cai LS, Chin FT, Pike VW, Toyama H, Liow JS, Zoghbi SS, Modell K, Briard E, Shetty HU, Sinclair K, et al. Synthesis and evaluation of two 18F-labeled 6-iodo-2-(4′-N,N-dimethylamino)phenylimidazo[1,2-a]pyridine derivatives as prospective radioligands for beta-amyloid in Alzheimer’s disease. J Med Chem 2004; 47:2208 - 18; http://dx.doi.org/10.1021/jm030477w; PMID: 15084119
- Klunk WE, Wang YM, Huang GF, Debnath ML, Holt DP, Shao L, Hamilton RL, Ikonomovic MD, DeKosky ST, Mathis CA. The binding of 2-(4′-methylaminophenyl)benzothiazole to postmortem brain homogenates is dominated by the amyloid component. J Neurosci 2003; 23:2086 - 92; PMID: 12657667
- Mastrianni JA. Prion diseases. Clin Neurosci Res 2004; 3:469 - 80; http://dx.doi.org/10.1016/j.cnr.2004.04.012
- Ono M, Saji H. Molecular approaches to the treatment, prophylaxis, and diagnosis of Alzheimer’s disease: novel PET/SPECT imaging probes for diagnosis of Alzheimer’s disease. J Pharmacol Sci 2012; 118:338 - 44; http://dx.doi.org/10.1254/jphs.11R08FM; PMID: 22382657
- Verhoeff NP, Wilson AA, Takeshita S, Trop L, Hussey D, Singh K, Kung HF, Kung MP, Houle S. In-vivo imaging of Alzheimer disease beta-amyloid with [11C]SB-13 PET. Am J Geriatr Psychiatry 2004; 12:584 - 95; PMID: 15545326
- Kudo Y, Okamura N, Furumoto S, Tashiro M, Furukawa K, Maruyama M, Itoh M, Iwata R, Yanai K, Arai H. 2-(2-[2-Dimethylaminothiazol-5-yl]ethenyl)-6- (2-[fluoro]ethoxy)benzoxazole: a novel PET agent for in vivo detection of dense amyloid plaques in Alzheimer’s disease patients. J Nucl Med 2007; 48:553 - 61; http://dx.doi.org/10.2967/jnumed.106.037556; PMID: 17401091
- Johnson AE, Jeppsson F, Sandell J, Wensbo D, Neelissen JAM, Juréus A, Ström P, Norman H, Farde L, Svensson SPS. AZD2184: a radioligand for sensitive detection of beta-amyloid deposits. J Neurochem 2009; 108:1177 - 86; http://dx.doi.org/10.1111/j.1471-4159.2008.05861.x; PMID: 19141073
- Zhang LJ, Xu CL, Li BX. Simple and sensitive detection method for chromium(VI) in water using glutathione-capped CdTe quantum dots as fluorescent probes. Mikrochim Acta 2009; 166:61 - 8; http://dx.doi.org/10.1007/s00604-009-0164-0
- Li T, Zhou YY, Sun JY, Tang DB, Guo SX, Ding XP. Ultrasensitive detection of mercury(II) ion using CdTe quantum dots in sol-gel-derived silica spheres coated with calix 6 arene as fluorescent probes. Mikrochim Acta 2011; 175:113 - 9; http://dx.doi.org/10.1007/s00604-011-0655-7
- Li YL, Zhou J, Liu CL, Li HB. Composite quantum dots detect Cd(II) in living cells in a fluorescence “turning on” mode. J Mater Chem 2012; 22:2507 - 11; http://dx.doi.org/10.1039/c1jm14317d
- Algar WR, Tavares AJ, Krull UJ. Beyond labels: a review of the application of quantum dots as integrated components of assays, bioprobes, and biosensors utilizing optical transduction. Anal Chim Acta 2010; 673:1 - 25; http://dx.doi.org/10.1016/j.aca.2010.05.026; PMID: 20630173
- Chen JA, Pei Y, Chen ZW, Cai JY. Quantum dot labeling based on near-field optical imaging of CD44 molecules. Micron 2010; 41:198 - 202; http://dx.doi.org/10.1016/j.micron.2009.11.002; PMID: 19959369
- Resch-Genger U, Grabolle M, Cavaliere-Jaricot S, Nitschke R, Nann T. Quantum dots versus organic dyes as fluorescent labels. Nat Methods 2008; 5:763 - 75; http://dx.doi.org/10.1038/nmeth.1248; PMID: 18756197
- Das P, Zhong WH, Claverie JP. Copolymer nanosphere encapsulated CdS quantum dots prepared by RAFT copolymerization: synthesis, characterization and mechanism of formation. Colloid Polym Sci 2011; 289:1519 - 33; http://dx.doi.org/10.1007/s00396-011-2466-0
- Kumar KS, Divya A, Reddy PS. Synthesis and characterization of Cr doped CdS nanoparticles stabilized with polyvinylpyrrolidone. Appl Surf Sci 2011; 257:9515 - 8; http://dx.doi.org/10.1016/j.apsusc.2011.06.048
- Gondal MA, Bagabas AA, Dastageer MA. Synthesis of w-CdS quantum dots and discovery of intense sub band emission owing to longitudinal optical phonons. J Nanopart Res 2011; 13:3835 - 42; http://dx.doi.org/10.1007/s11051-011-0318-y
- Gu Y, Kuskovsky IL, Fung J, Robinson R, Herman IP, Neumark GF, Zhou X, Guo SP, Tamargo MC. Determination of size and composition of optically active CdZnSe/ZnBeSe quantum dots. Appl Phys Lett 2003; 83:3779 - 81; http://dx.doi.org/10.1063/1.1623941
- Rameshwar T, Samal S, Lee S, Kim S, Cho J, Kim IS. Determination of the size of water-soluble nanoparticles and quantum dots by field-flow fractionation. J Nanosci Nanotechnol 2006; 6:2461 - 7; http://dx.doi.org/10.1166/jnn.2006.544; PMID: 17037856
- Lin ZH, Wang MQ, Wei LZ, Song XH, Xue YH, Yao X. Synthesis and characterization of ZnSe/ZnS core/shell nanocrystals by aqueous reflux route. J Alloy Comp 2011; 509:8356 - 9; http://dx.doi.org/10.1016/j.jallcom.2011.03.070
- Huang FH, Lan YL, Chen PF. Synthesis and characterization of water-soluble l-cysteine-modified ZnS nanocrystals doped with silver. J Mater Sci 2011; 46:5732 - 6; http://dx.doi.org/10.1007/s10853-011-5527-3
- Khene S, Moeno S, Nyokong T. Voltammetry and electrochemical impedance spectroscopy of gold electrodes modified with CdTe quantum dots and their conjugates with nickel tetraamino phthalocyanine. Polyhedron 2011; 30:2162 - 70; http://dx.doi.org/10.1016/j.poly.2011.06.002
- Li YF, Han M, Bai HY, Wu Y, Dai ZH, Bao JC. A sensitive electrochemical aptasensor based on water soluble CdSe quantum dots (QDs) for thrombin determination. Electrochim Acta 2011; 56:7058 - 63; http://dx.doi.org/10.1016/j.electacta.2011.05.119
- Wang QS, Yang L, Fang TT, Wu S, Liu P, Min XM, Li X. Interactions between CdSe/CdS quantum dots and DNA through spectroscopic and electrochemical methods. Appl Surf Sci 2011; 257:9747 - 51; http://dx.doi.org/10.1016/j.apsusc.2011.05.123
- Ghaderi S, Ramesh B, Seifalian AM. Fluorescence nanoparticles “quantum dots” as drug delivery system and their toxicity: a review. J Drug Target 2011; 19:475 - 86; http://dx.doi.org/10.3109/1061186X.2010.526227; PMID: 20964619
- Hoshino A, Hanada S, Yamamoto K. Toxicity of nanocrystal quantum dots: the relevance of surface modifications. Arch Toxicol 2011; 85:707 - 20; http://dx.doi.org/10.1007/s00204-011-0695-0; PMID: 21445587
- Pelley JL, Daar AS, Saner MA. State of academic knowledge on toxicity and biological fate of quantum dots. Toxicol Sci 2009; 112:276 - 96; http://dx.doi.org/10.1093/toxsci/kfp188; PMID: 19684286
- Hardman R. A toxicologic review of quantum dots: toxicity depends on physicochemical and environmental factors. Environ Health Perspect 2006; 114:165 - 72; http://dx.doi.org/10.1289/ehp.8284; PMID: 16451849
- Ryvolova M, Chomoucka J, Janu L, Drbohlavova J, Adam V, Hubalek J, Kizek R. Biotin-modified glutathione as a functionalized coating for bioconjugation of CdTe-based quantum dots. Electrophoresis 2011; 32:1619 - 22; PMID: 21607989
- Bottini M, Cerignoli F, Dawson MI, Magrini A, Rosato N, Mustelin T. Full-length single-walled carbon nanotubes decorated with streptavidin-conjugated quantum dots as multivalent intracellular fluorescent nanoprobes. Biomacromolecules 2006; 7:2259 - 63; http://dx.doi.org/10.1021/bm0602031; PMID: 16903668
- Shao J, You XG, Gao F, He R, Cui DX. Labeling of quantum dots with streptavidin and its bioactivity measurement. Chin J Anal Chem 2006; 34:1625 - 8
- Wu Y, Lopez GP, Sklar LA, Buranda T. Spectroscopic characterization of streptavidin functionalized quantum dots. Anal Biochem 2007; 364:193 - 203; http://dx.doi.org/10.1016/j.ab.2007.02.007; PMID: 17368555
- Bruchez M Jr., Moronne M, Gin P, Weiss S, Alivisatos AP. Semiconductor nanocrystals as fluorescent biological labels. Science 1998; 281:2013 - 6; http://dx.doi.org/10.1126/science.281.5385.2013; PMID: 9748157
- Chan WCW, Nie SM. Quantum dot bioconjugates for ultrasensitive nonisotopic detection. Science 1998; 281:2016 - 8; http://dx.doi.org/10.1126/science.281.5385.2016; PMID: 9748158
- Pellegrino T, Manna L, Kudera S, Liedl T, Koktysh D, Rogach AL, Keller S, Radler J, Natile G, Parak WJ. Hydrophobic nanocrystals coated with an amphiphilic polymer shell: A general route to water soluble nanocrystals. Nano Lett 2004; 4:703 - 7; http://dx.doi.org/10.1021/nl035172j
- Fernandez-Argüelles MT, Yakovlev A, Sperling RA, Luccardini C, Gaillard S, Medel AS, Mallet JM, Brochon JC, Feltz A, Oheim M, et al. Synthesis and characterization of polymer-coated quantum dots with integrated acceptor dyes as FRET-based nanoprobes. Nano Lett 2007; 7:2613 - 7; http://dx.doi.org/10.1021/nl070971d; PMID: 17691850
- Lin CAJ, Sperling RA, Li JK, Yang TY, Li PY, Zanella M, Chang WH, Parak WJ. Design of an amphiphilic polymer for nanoparticle coating and functionalization. Small 2008; 4:334 - 41; http://dx.doi.org/10.1002/smll.200700654; PMID: 18273855
- Alivisatos AP. Birth of a nanoscience building block. ACS Nano 2008; 2:1514 - 6; http://dx.doi.org/10.1021/nn800485f; PMID: 19206353
- Sobrova P, Ryvolova M. J. H, Adam V, Kizek R. Femtogram Electrochemical Sensing of Prion Proteins Using Quantum Dots. Anal Chim Acta 2013; Submitted
- Duan JL, Song LX, Zhan JH. One-Pot Synthesis of Highly Luminescent CdTe Quantum Dots by Microwave Irradiation Reduction and Their Hg(2+)-Sensitive Properties. Nano Res 2009; 2:61 - 8; http://dx.doi.org/10.1007/s12274-009-9004-0
- Dalal V, Bhattacharya M, Narang D, Sharma PK, Mukhopadhyay S. Nanoscale Fluorescence Imaging of Single Amyloid Fibrils. J Phys Chem Lett 2012; 3:1783 - 7; http://dx.doi.org/10.1021/jz300687f
- Zhou YW, Li CM, Liu Y, Huang CZ. Effective detection and cell imaging of prion protein with new prepared targetable yellow-emission silver nanoclusters. Analyst 2013; 138:873 - 8; http://dx.doi.org/10.1039/c2an36456e; PMID: 23223184
- Gao XH, Yang LL, Petros JA, Marshall FF, Simons JW, Nie SM. In vivo molecular and cellular imaging with quantum dots. Curr Opin Biotechnol 2005; 16:63 - 72; http://dx.doi.org/10.1016/j.copbio.2004.11.003; PMID: 15722017
- Michalet X, Pinaud FF, Bentolila LA, Tsay JM, Doose S, Li JJ, Sundaresan G, Wu AM, Gambhir SS, Weiss S. Quantum dots for live cells, in vivo imaging, and diagnostics. Science 2005; 307:538 - 44; http://dx.doi.org/10.1126/science.1104274; PMID: 15681376
- Smith AM, Duan HW, Mohs AM, Nie SM. Bioconjugated quantum dots for in vivo molecular and cellular imaging. Adv Drug Deliv Rev 2008; 60:1226 - 40; http://dx.doi.org/10.1016/j.addr.2008.03.015; PMID: 18495291
- Hu R, Zhang XB, Kong RM, Zhao XH, Jiang JH, Tan WH. Nucleic acid-functionalized nanomaterials for bioimaging applications. J Mater Chem 2011; 21:16323 - 34; http://dx.doi.org/10.1039/c1jm12588e
- Taniguchi S, Green M, Rizvi SB, Seifalian A. The one-pot synthesis of core/shell/shell CdTe/CdSe/ZnSe quantum dots in aqueous media for in vivo deep tissue imaging. J Mater Chem 2011; 21:2877 - 82; http://dx.doi.org/10.1039/c0jm03527k
- Ciarlo M, Russo P, Cesario A, Ramella S, Baio G, Neumaier CE, Paleari L. Use of the semiconductor nanotechnologies “quantum dots” for in vivo cancer imaging. Recent Pat Anticancer Drug Discov 2009; 4:207 - 15; http://dx.doi.org/10.2174/157489209789206841; PMID: 19715554
- Tavares AJ, Chong LR, Petryayeva E, Algar WR, Krull UJ. Quantum dots as contrast agents for in vivo tumor imaging: progress and issues. Anal Bioanal Chem 2011; 399:2331 - 42; http://dx.doi.org/10.1007/s00216-010-4010-3; PMID: 20658228
- Jetha NN, Semenchenko V, Wishart DS, Cashman NR, Marziali A. Nanopore Analysis of Wild-Type and Mutant Prion Protein (PrPC): Single Molecule Discrimination and PrPC Kinetics. PLoS ONE 2013; 8:1 - 10; http://dx.doi.org/10.1371/journal.pone.0054982