Abstract
Species, as well as individuals within species, have unique susceptibilities to prion infection that are likely based on sequence differences in cellular prion protein (PrPC). Species barriers to transmission also reflect PrPC sequence differences. Defining the structure-activity relationship of PrPC/PrPSc with respect to infectivity/susceptibility will benefit disease understanding and assessment of transmission risks. Here, nanopore analysis is employed to investigate genotypes of sheep PrPC corresponding to differential susceptibilities to scrapie infection. Under non-denaturing conditions scrapie resistant (ARR) and susceptible (VRQ) genotypes display similar, type I (bumping) predominant event profiles, suggesting a conserved folding pattern. Under increasingly denaturing conditions both proteins shift to type II (intercalation/translocation) events but with different sensitivities to unfolding. Specifically, when pre-incubated in 2M Gdn-HCl, the VRQ variant had more of type II events as compared with the ARR protein, suggesting a more flexible unfolding pattern. Addition of PrPSc-specific polyclonal antibody (YML) to the ARR variant, pre-incubated in 2M Gdn-HCl, reduced the number of type II events with no clear intercalation/translocation peak, whereas for VRQ, type II events above blockades of 90 pA bound YML. A second PrPSc-specific antibody (SN6b) to a different cryptic epitope reduced type II events for VRQ but not the ARR variant. Collectively, the event patterns associated with sequential denaturation, as well as interactions with PrPSc-specific antibodies, support unique patterns and/or propensities of misfolding between the genotypes. Overall, nanopore analysis identifies intermediate conformations that occur during the unfolding pathways of ARR and VRQ genotypes and may help to understand the correlation of structural properties that induce protein misfolding.
Introduction
Prion diseases are fatal neurodegenerative diseases that are based upon a conformational change of the cellular prion protein (PrPC) into a pathological conformation (PrPSc).Citation1,Citation2 The PrPSc isoform, through its ability to induce the misfolding of PrPC into PrPSc, represents an infectious entity.Citation3,Citation4 Prion diseases represent the first, and arguably the only, example of an infectious disease that is mediated exclusively by a protein.Citation5 Prion diseases, also known as transmissible spongiform encephalopathies (TSE), include Kuru, Creutzfeldt-Jakob disease (CJD), Gerstmann-Sträussler-Scheinker syndrome (GSS), and fatal familial insomnia (FFI) in humans; bovine spongiform encephalopathy (BSE) in cattle; scrapie in sheep; and chronic wasting disease (CWD) in deer and elk.Citation6-Citation12 Within humans and animals prion disease can be initiated from either an internal or external locus of origin.Citation13
External sources of prion infection include contact with environmental prions, consumption of prion-infected animals/animal products, exposure to prion-infected medical equipment/reagents or direct contact with a prion-infected animal.Citation4,Citation14 Susceptibility to prion infection from external sources has a genetic component as different sequences of the prion protein gene (PRNP) determine the susceptibility of particular species, as well as the susceptibilities of individuals within a species, to prion infection.Citation15-Citation17 For example, cattle are highly susceptible to prion infection whereas rabbits are generally regarded as being prion resistant (rabbits are not resistant to prions).Citation18,Citation19 Further, natural sequence variations of PrPC within species contribute to disease susceptibility.Citation16-Citation18 For example, during the UK mad-cow outbreak of the 1980s in which millions of people consumed prion-infected beef, incidence of human prion disease was limited almost exclusively to individuals having methionine at codon 129 of a homozygous genotype.Citation20,Citation21
Prion diseases originating from an internal locus of infection (in which there is no exposure to external prions) include spontaneous forms of disease (in which there is no identified genetic basis) and familial prion diseases (in which there is a defined genetic basis for disease).Citation13 Given the unique etiology of the prion diseases, there has been considerable interest in defining the rules that govern disease susceptibility. This includes efforts to rationalize the structural basis of the unique disease-susceptibilities associated with different natural PrPC sequence variants. For example, molecular dynamic simulation studies on the T183A mutant PrPC, which is associated with rapid and spontaneous prion disease in humans, shows increased flexibility and instability as a consequence of the mutation.Citation22 It has also been speculated that the rapid proliferation of CWD within wild cervid populations reflects structural contributions of a rigid loop region of PrPC that predisposes for protein misfolding.Citation23
While understanding the structural basis for prion infectivity and susceptibility is of obvious interest for deciphering the mechanisms of this novel paradigm of infection, there is also considerable practical importance to unraveling this complex structure-activity relationship. Currently the unabated spread of CWD through wild cervids of North America is having dramatic impact on the health and viability of these herds. A secondary consequence of the propagation of prion diseases in these far-ranging animals is the opportunity for exposure of other species to CWD prions either through direct contact with infected cervids, exposure to environmental contamination or consumption of prion-infected meat. It is of critical importance to understanding which, if any, of these species, or genetic sub-populations within these species, is at greatest risk for infection.
There has been the greatest opportunity to study the structure-activity relationship of PrPC/PrPSc within the context of sheep scrapie which was first reported in 1732. Investigations of scrapie identified polymorphisms at codons 136, 154, and 171 in the PRNP gene that are linked to scrapie susceptibility.Citation24,Citation25 For example, a dominant-negative polymorphism at Q171R protects sheep from scrapie.Citation26 The most resistant homozygous genotype is ARR (A[136], R[154], R[171]) whereas VRQ (V[136], R[154], Q[171]) presents the greatest susceptibility.Citation27 An intermediate scrapie susceptibility is associated with the ARQ (A[136], R[154], Q[171]) variant.Citation26-Citation28 Polymorphisms in the PRNP gene may influence the conversion of PrPC to PrPSc through different folding patterns governed by thermodynamic stability.Citation29 For example, using scanning calorimetry within a wide range of pH’s revealed a random coil unfolding pattern for the ARR genotype compared with a β-sheet structure seen during unfolding of ARQ and VRQ PrPC.Citation30 Another study, utilizing circular dichroism and proteolytic digestion, revealed that VRQ cellular PrPC is intrinsically more compact than ARR PrPC.Citation31 Evidence from microsecond unfolding kinetics reveal that the susceptible variant for sheep (amino acids 94–233) may favor protein oligomerization.Citation32
Methods that provide opportunity to characterize conformation changes within individual protein molecules offer considerable advantage for defining complex folding/unfolding patterns. Single-molecule fluorescence resonance energy transfer (FRET), optical tweezers/optical traps and atomic force microscopy (AFM) are some of the most common methods to study individual molecules.Citation33-Citation35 Nanopore analysis also allows investigation of structural characteristics of individual peptides or proteins via characterization of their interactions with a biological pore.Citation36-Citation39 In nanopore analysis, single molecules are interrogated with the pore to give rise to distinct event parameters, blockade current (I) and blockade time (T).Citation36-Citation39 These parameters can be used to predict different conformations within the molecule of interest.Citation40,Citation41 A considerable advantage of nanopore analysis over other single-molecule based techniques is that it does not depend on immobilization of the molecule of interest to a solid matrix and therefore provides greater opportunity for unbiased observation of biologically relevant folding and unfolding events. It is also performed at low concentrations which mitigates against aggregation.Citation38,Citation39 Previously nanopore analysis has allowed detailed investigation of various aspects of the PrPC structure-activity relationship including the interaction of PrPC with metal ions and characterization of the consequences of disease-associated single amino acid mutations on interaction with a therapeutic PrPSc-antibody.Citation42-Citation44 Accordingly nanopore analysis was a logical approach to investigate genetic polymorphisms of ovine PrPC that are associated with differential susceptibilities to scrapie infection.
In this study nanopore analysis was employed to investigate unfolding patterns of scrapie resistant (ARR) and susceptible (VRQ) genotypes. Under non-denaturing conditions scrapie resistant (ARR) and susceptible (VRQ) genotypes display similar, type I (bumping) predominant event profiles, suggesting a conserved folding pattern. Under increasingly denaturing conditions, however, structural differences in the proteins are apparent. In particular, following pre-incubation of the proteins with 2M Gdn-HCl a difference in the number of type II events was observed for the VRQ isoform, indicating a more flexible unfolding pattern for this variant. The unique patterns of unfolding of these sequence isoforms under denaturing conditions was further supported by the unique patterns of complex formation that resulted from incubation of the isomers with two antibodies that recognize distinct epitopes that are buried in the native conformation.Citation45-Citation47 Collectively, the sensitivity of nanopore analysis to identify intermediate conformations that occur during the unfolding pathways of ARR and VRQ genotypes in sheep may help understand the correlation of the different structural properties that induce protein misfolding.
Results
Nanopore analysis of denaturation of ovine ARR and VRQ PrPC
In nanopore analysis an “event” is defined as the interaction of an individual molecule with the pore.Citation36-Citation39 Type I events have small blockade currents and times that represent a molecule bumping into the pore and diffusing away.Citation36 Type II events occur when a portion of the molecule locks into the vestibule of the pore and may diffuse back to the cis side (intercalation) or threads through the pore (translocation) to the trans side.Citation36,Citation37 Intercalation/translocation events have larger blockade currents and times as compared with type I events.Citation37 At present it is very difficult to distinguish between intercalation and translocation events for proteins. Therefore we refer to events having a blockade current of <40 pA as type I (bumping) and >40 pA as type II (intercalation/translocation). The conceptual differences between a type I and II events, as well as raw data representing these events, are presented in ().
Figure 1. Representation and documentation of Type I and Type II events through nanopore analysis. (A) Cartoon representation of the interaction between the pore and protein of interest that lead to Type I and Type II (intercalation or translocation events). (B) Raw data from the current project illustrating Type I and Type II events.
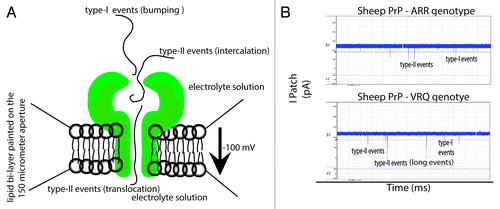
Under non-denaturing conditions proteins corresponding to the ARR or VRQ genotypes of ovine PrPC were added to the cis chamber after the formation of stable pores in a synthetic lipid membrane. The complete event parameters for these proteins are listed in () for the ARR and VRQ genotypes. For ARR genotype under non-denaturing conditions (, a), a single Gaussian distribution of type I events (<40 pA) with an average blockage current of –27 pA was recorded. For the VRQ genotype the peak for type I events is considerably broader (, b). In both cases, the profiles show a majority of bumping events but there are proportionally more for ARR compared with the VRQ genotype. Even though the N-terminal truncated ARR and VRQ proteins differ by a single amino acid at the C-terminal ([ARR: stop sequence RGAS]; [VRQ: stop sequence RGA]) they both show mostly type I events under native conditions. If this extra amino acid at the C-terminal altered the conformation of the protein, it would have been detected by a change in events with nanopore analysis. Upon pre-treatment with 1 or 2M Gdn-HCl (, c and d, , e and f) there is a significant increase in the proportion of intercalation/translocation events and a corresponding decrease in bumping events. In particular at 2M Gdn-HCl, there are 51% type II events for ARR (, e) but 63% for the VRQ phenotype (, f). Additionally, at 2M Gdn-HCl the full-length sheep prion protein (aa 25–231) of the ARR genotype also gave similar type I and II events as of ARR (94–234) (data not shown). Finally, pre-treatment of ARR in 3M Gdn-HCl (, g) shifted the peak centered for type II events from –77 pA to –58 pA whereas for VRQ pre-treatment in 3M Gdn-HCl (, h) shifted the Gaussian peak centered for the type II events to –73 pA. Collectively these results suggest a more flexible conformation for VRQ as compared with ARR. In another vein, a potential of 100 mV is sufficient to unfold proteins under denaturing conditions that favor the formation of an unfolded intermediate for the VRQ genotype. Subsequently, this intermediate may generate a more tractable protein.
Table 1. Event parameters for Ovine PrP(94–234), ARR genotype and Ovine PrP(94–233), VRQ genotype pre-treated with 0 M, 1 M, 2 M, and 3 M Gdn-HCla
Figure 2. Nanopore Analysis of Denaturation of Ovine ARR and VRQ PrPC. Current blockade histograms for E.coli expressed sheep PrPC for the two genotypes, ARR and VRQ. (A) under non-denaturing conditions (0 M Gdn-HCl): (a) OvPrP(94–234) ARR genotype, (b) OvPrP(94–233) VRQ genotype. (B) Under denaturing conditions (1 M Gdn-HCl): (c) OvPrP(94–234) ARR genotype, (d) OvPrP(94–233) VRQ genotype. (C) Under denaturing conditions (2 M Gdn-HCl): (e) OvPrP(94–234) ARR genotype, (f) OvPrP(94–233) VRQ genotype. (D) Under denaturing conditions (3 M Gdn-HCl): (g) OvPrP(94–234) ARR genotype, (h) OvPrP(94–233) VRQ genotype. The values are listed in .
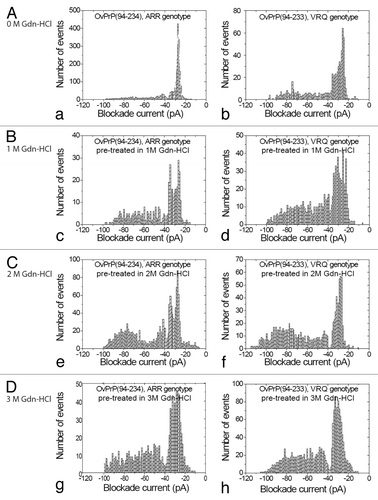
More detailed consideration of the nanopore results following pre-treatment of the ovine ARR and VRQ PrPC proteins with 2M Gdn-HCl reveals critical differences in the unfolding patterns of these two proteins. Specifically, the blockade time for the type II events for VRQ () is approximately double as compared with the ARR genotype (). This may indicate that the VRQ genotype generates a more flexible unfolded protein conformation that can bind to the pore more tightly compared with the unfolded ARR genotype. The type I events give almost similar blockade times ().
Figure 3. Blockade time for molecules interacting with the α-hemolysin pore in nanopore analysis for Ovine ARR and VRQ PrPC pre-treated in 2 M Gdn-HCl. Exponential curves depicting the blockade time for (A) bumping time (T1) for OvPrP(94–234) ARR genotype. (B) Intercalation/translocation time (T2) for OvPrP(94–234) ARR genotype. (C) bumping time (T1) for OvPrP(94–233) VRQ genotype. (D) Intercalation/translocation time (T2) for OvPrP(94–233) VRQ genotype.
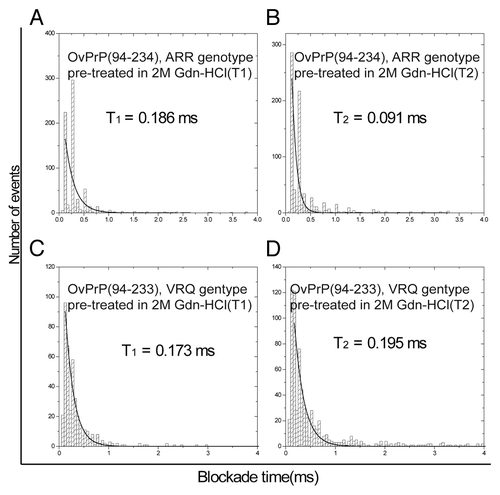
Binding of PrPSc-specific antibodies to unfolding intermediates of ovine ARR and VRQ PrPC
Within the context of developing a PrPSc-specific vaccine for prion diseases our lab developed and purified a number of antibodies that bind to regions (epitopes) of PrPC that are only exposed upon misfolding of the protein. These include polyclonal antibodies SN6b against the epitope QVYYRPVDQYSNQN as described in Hedlin et al.Citation47 as well as an YML antibody against the sequence SGYMLGSAMSRP.Citation45,Citation46 Previously we have reported on the utility of PrPSc-specific antibodies as tools to compliment nanopore analysis.Citation44 Here the two PrPSc-specific antibodies, targeting two distinct cryptic epitopes of ovine PrPC, are used in combination with nanopore analysis to investigate generation of folding intermediates of the two genotypes during denaturation.
For the ARR protein, addition of the YML antibody to the sample pre-treated with 2M Gdn-HCl (, a) reduced the number of type II events with no clear Gaussian peak. The complete events parameters are stated in . A peak with 56% type I events having a Gaussian distribution was present. In contrast the same antibody against the VRQ protein pre-treated with 2M Gdn-HCl (, b) removed all the events above 90 pA and a shift in the Gausian peak for the type II events to –62 pA was observed. Similarly, a second PrPSc-antibody, SN6b, against a different cryptic epitope of PrPC resulted in a reduction of the type II events for VRQ (, f) with only a modest reduction of the type II events for the ARR variant (, e). Additionally, a control experiment under native conditions for the VRQ protein did not show a significant change in the percentage of type I events upon addition of antibody SN6b as would be expected if the antibody did not bind to the native protein (data not shown). Collectively the unique patterns of complex formation with the two PrPSc-antibodies indicate unique pathways, or at least propensities, for misfolding of the ARR and VRQ variants of ovine PrPC. Histograms depicting the overlapping of events with and without antibodies for the ARR and VRQ genotypes are shown in (, c and d, , g and h) for the YML and SN6b antibodies, respectively.
Figure 4. Prion antibody interactions. Current blockade histograms for sheep PrPC genotypes, ARR and VRQ pre-treated in 2M Gdn-HCl with YML or SN6b antibodies. (A) YML antibody interactions: (a) OvPrP(94–234), ARR genotype pre-treated in 2 M Gdn-HCl with YML at a 1:1 ratio. (b) OvPrP(94–233), VRQ genotype pre-treated in 2 M Gdn-HCl with YML at a 1:1 ratio. (c) Overlapping histograms of OvPrP(94–234), ARR genotype pre-treated in 2 M Gdn-HCl with and without YML antibody. (d) Overlapping histograms of OvPrP(94–233), VRQ genotype pre-treated in 2 M Gdn-HCl with and without YML antibody. (B) SN6b antibody interactions: (e) OvPrP(94–234), ARR genotype pre-treated in 2 M Gdn-HCl with SN6b at a 1:1 ratio. (f) OvPrP(94–233), VRQ genotype pre-treated in 2 M Gdn-HCl with SN6b at a 1:1 ratio. (g) Overlapping histograms of OvPrP(94–234), ARR genotype pre-treated in 2 M Gdn-HCl with and without SN6b antibody. (h) Overlapping histograms of OvPrP(94–233), VRQ genotype pre-treated in 2 M Gdn-HCl with and without SN6b antibody. The values are listed in .
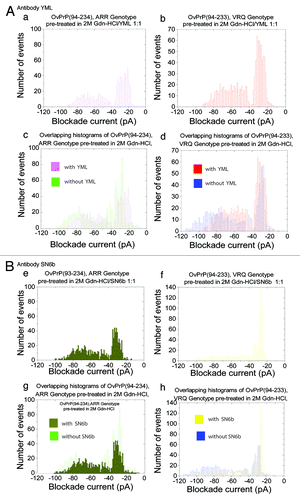
Table 2. Event parameters for OvPrP(94–234):ARR genotype and OvPrP(94–233), VRQ genotype in 2M Gdn-HCl with YML or SN6b complexa
Discussion
Previously, our group demonstrated the binding of SN6b with a mutant of the bovine prion T194A, which is a homolog of the human T183A.Citation44 Comparable results were also obtained for the polyclonal antibody YML with bovine T194A (data not shown). The dispose of two hydrogen bonds between helix 2 and the β-sheet generates a more flexible prion for the T183A mutation.Citation48 This may enhance the formation of other intermediate PrPC conformations that can lead PrPSc.Citation48 Similarly natural sequence variations within the ovine PRNP gene have important and well documented consequences on the susceptibility of sheep to scrapie infection.Citation26-Citation28 This provides an interesting and relevant example to investigate how subtle variations in the sequence influence structural characteristics of this protein, in particular with respect to propensity for unfolding. Intermediates that occur during the unfolding process may have different protein plasticity that may influence the transition from PrPC to PrPSc. To address the conformational changes that occur during protein denaturation we used nanopore analysis, a sensitive single molecule technique, to investigate unfolding events that occur with two sheep PrPC sequence variants corresponding to scrapie resistance (ARR) and susceptible (VRQ) proteins. In the absence of a denaturant proteins corresponding to the ARR and VRQ genotypes showed similar event profiles representing a predominance of type I events. This is consistent with similar investigations with circular dichroism (CD) that did not show any significant variations of secondary structure between these same ovine PrPC variants.Citation30 Under non-denaturing conditions, nanopore analysis does not demonstrate propensity for spontaneous misfolding of the VRQ genotype.
Using step-wise gradients of a denaturing agent (Gdn-HCl) it is possible to observe trends and propensities for misfolding with each of the genotypes. At each of the extremes of denaturant that were considered (0 M and pre-incubation at 3 M Gdn-HCl) the proteins show almost identical event profiles, suggestive of structural similarity in the completely folded and completely unfolded states. It is unlikely that the additional amino acid serine (S) at the C-terminal of the ARR prion protein causes a conformational change because there is no difference in type II events as compared with the VRQ genotype under native and extreme denaturation (3 M Gdn-HCl). Under conditions representing partial denaturation, or relaxation of the protein structures, differences in the event profiles are apparent between the two genotypes which suggest unique patterns or propensities for unfolding. In particular a unique difference between ARR and VRQ was observed when protein was pre-treated at 2 M Gdn-HCl. The VRQ genotype seems to be more flexible when unfolding at a concentration of 2 M Gdn-HCl, where a certain number of protein-pore interaction events indicate threading into the pore having a larger current blockade than 100 pA. This is not seen in the ARR genotype. Under denatured conditions the VRQ genotype may favor the formation of a more stretchable protein that can perhaps now enter the stem region of the α-hemolysin pore and cause type II (intercalation/translocation) events with blockade current above 100 pA. Even though this may be a subtle change between the two proteins, it suggests two conformations one being more flexible (VRQ genotype) and perhaps easier to misfold into an intermediate that can promote misfolding. This conclusion was further supported through the use of two conformation-specific antibodies to the prion protein whose epitopes are unavailable for binding when PrPC is in its native conformation. The unique association of each of these antibodies with each of these sequence variants during the unfolding process supports the existence of unique unfolding intermediates for each polymorphism. Hypothetically these intermediates might represent early intermediates for prion seeding.
Materials and Methods
Plasmid constructs of ovine ARR and VRQ genotypes
For the ARR genotype, Ovine cDNA encoding amino acids (94–234) was amplified using PCR with forward, 5′-AGAATTCATA TGGGTCAAGG TGGTAGCCACA GTC-3′ and reverse 5′-CGCGGATCCC TAACTTGCCC CCCTTTGGTA ATAAGC-3′ primers. The PCR product was first sequentially digested with NdeI and BamHI-HF, and inserted into cloning vector pET-15b. The sequence was verified by DNA sequencing. The ovine ARR genotype construct encoded for a protein with polymorphisms at positions 136(A), 154(R) and 171(R), which was identical to the National Centre for Biotechnology Information (NCBI) accession number: DQ677230. The plasmid containing polymorphisms at positions 136(V), 154(R) and 171(Q) was a gift from PrioNet Prion Protein and Plasmid Production Facility. The Ovine PrP for the VRQ variant encoded amino acids (94–233).
Protein expression, preparation of inclusion bodies and purification
A 10 ml Luria-Bertani (LB) medium containing ampicilin (100 µg/ml) was inoculated with Escherichia coli BL 21(DE3) cells that were previously transformed with plasmids encoding the ARR or VRQ variants. This culture was grown overnight at 37 °C and transferred to 500 ml of LB containing ampicilin and grown until the optical density at 600 nm reached 0.6. Protein expression was induced (IPTG) at a final concentration of 1 mM, and the culture was grown for another 4 h. The cells were harvested by centrifugation at 10 000 × g for 15 min at 4 °C. The supernatant was discarded and the pellet was resuspended in 50 mM Tris containing 25% sucrose at pH 8.0. This was followed by lysis, sonication and solubilization of inclusion bodies in 6M Gdn-HCl containing 100 mM NaH2PO4 and 10 mM Tris at pH 8.0. The His-tagged prion protein was purified using a Ni-NTA agarose (R901-01; Life Technologies) with a bio-spin chromatography column (732-6008EDU; Bio-Rad). On column protein refolding was performed using a gradient of urea containing buffers. Finally, Ovine PrP was eluted with 0.25 M imidazole (Fig. S1).
α-Hemolysin pore and polyclonal antibodies
α-hemolysin needed for the formation of the pore was purchased from (H9395; Sigma-Aldrich) and resuspended in 1.0 M KCl 10 mM TRIS-HCl buffer, pH 7.8. The concentration of the working solution of α-hemolysin was 2 µg/ml. The YML polyclonal antibody was raised against an in silico optimized expansion of the YML core epitope with the sequence GYMLGSAMSRP, presented in a repeat motif (forward-back-back)4, and affinity purified from sheep serum. Polyclonal antibody SN6b, was raised against an immunogenic epitope QVYYRPVDQYSNQN that was represented in the form of a (forward-back-back)4, repeat sequence and was affinity purified from sheep serum.Citation45-Citation47
Nanopore analysis
Single molecule nanopore analysis was used as the primary technique to determine conformational changes in prion protein genotypes and prion-antibody interactions. For nanopore analysis, an Axopatch 200B amplifier (Axon Instruments) was connected to a CV203BU head-stage (Axon Instruments) and was used at 22 ± 1 °C. Experiments were conducted with an applied potential of –100 mV at a bandwidth of 10 KHz.Citation36-Citation39,Citation43 A perfusion unit made up of a black perfusion chamber containing a white perfusion cup that divided two chambers (cis and trans) by a 150 µm aperture was used for nanopore analysis. A lipid bilayer was formed by applying a synthetic lipid, (1, 2-diphytanoyl-sn-glycero-3-phosphocholine [Avanti Polar Lipids]) on the 150 µm aperture (). After insertion of a α-hemolysin pore, the protein was added to a final concentration of 0.02 mg/ml in the 1.5 ml cis chamber proximal to the aperture.Citation36 For experiments under denatured conditions the protein was pre-incubated in 1 M, 2 M or 3 M Gdn-HCl for 15 min at room temperature and added to the cis chamber. For prion-antibody interactions the protein was incubated with antibody for 1 h at room temperature and added to the chamber.Citation43,Citation44 The electrolyte solution for nanopore experiments was 1.0 M KCl in 10 mM TRIS-HCl buffer at pH 7.8. The data recording and collection was done as “fixed length events” stated in the pClamp 9.0 User’s Guide. The raw data obtained from each experiment were analyzed using the Clampfit 9.2 software program from Axon Instruments.Citation36 Histograms for blockade current were constructed by fitting the populations for blockade current distribution with a Gaussian function. A single exponential function was used to fit each blockade duration distribution.Citation36,Citation38,Citation43 Most experiments were done more than once and gave reproducible results. A detailed statistical analysis is not possible, as is frequently the case with nanopore analysis.
Abbreviations: | ||
PrPC | = | cellular prion protein |
PrPSc | = | scrapie prion protein |
OvPrP | = | sheep prion protein |
Additional material
Download Zip (183.1 KB)Discosure of Potential Conflicts of Interest
No potential conflict of interest was disclosed.
Acknowledgments
Thank you to Dr David S Wishart (Department of Computing Science and Biological Sciences, University of Alberta, Edmonton, Alberta, T6G 2E8, Canada) for the VRQ plasmid. We also sincerely thank Dr Hugh GG Townsend (DVM, MSc), research scientist and program manager (Vaccine and Infectious Disease Organization, University of Saskatchewan, Saskatoon, Saskatchewan, S7N 5E3, Canada).
Footnotes
This work was supported by PrioNet Canada, Saskatchewan Agriculture and Development, Alberta Prion Research Institute and NSERC.
References
- Prusiner SB. Novel proteinaceous infectious particles cause scrapie. Science 1982; 216:136 - 44; http://dx.doi.org/10.1126/science.6801762; PMID: 6801762
- Pan KM, Baldwin M, Nguyen J, Gasset M, Serban A, Groth D, Mehlhorn I, Huang Z, Fletterick RJ, Cohen FE, et al. Conversion of alpha-helices into beta-sheets features in the formation of the scrapie prion proteins. Proc Natl Acad Sci U S A 1993; 90:10962 - 6; http://dx.doi.org/10.1073/pnas.90.23.10962; PMID: 7902575
- Stöhr J, Weinmann N, Wille H, Kaimann T, Nagel-Steger L, Birkmann E, Panza G, Prusiner SB, Eigen M, Riesner D. Mechanisms of prion protein assembly into amyloid. Proc Natl Acad Sci U S A 2008; 105:2409 - 14; http://dx.doi.org/10.1073/pnas.0712036105; PMID: 18268326
- Aguzzi A, Calella AM. Prions: protein aggregation and infectious diseases. Physiol Rev 2009; 89:1105 - 52; http://dx.doi.org/10.1152/physrev.00006.2009; PMID: 19789378
- Marciniuk K, Taschuk R, Napper S. Evidence for prion-like mechanisms in several neurodegenerative diseases: potential implications for immunotherapy. Clin Dev Immunol 2013; 2013:473706; http://dx.doi.org/10.1155/2013/473706; PMID: 24228054
- Prusiner SB. The prion diseases. Brain Pathol 1998; 8:499 - 513; http://dx.doi.org/10.1111/j.1750-3639.1998.tb00171.x; PMID: 9669700
- Klatzo I, Gajdusek DC, Zigas V. Pathology of Kuru. Lab Invest 1959; 8:799 - 847; PMID: 13665963
- Roos R, Gajdusek DC, Gibbs CJ Jr.. The clinical characteristics of transmissible Creutzfeldt-Jakob disease. Brain 1973; 96:1 - 20; http://dx.doi.org/10.1093/brain/96.1.1; PMID: 4633062
- Goldfarb LG, Petersen RB, Tabaton M, Brown P, LeBlanc AC, Montagna P, Cortelli P, Julien J, Vital C, Pendelbury WW, et al. Fatal familial insomnia and familial Creutzfeldt-Jakob disease: disease phenotype determined by a DNA polymorphism. Science 1992; 258:806 - 8; http://dx.doi.org/10.1126/science.1439789; PMID: 1439789
- Wood JL, McGill IS, Done SH, Bradley R. Neuropathology of scrapie: a study of the distribution patterns of brain lesions in 222 cases of natural scrapie in sheep, 1982-1991. Vet Rec 1997; 140:167 - 74; http://dx.doi.org/10.1136/vr.140.7.167; PMID: 9055393
- Wells GAH, Scott AC, Johnson CT, Gunning RF, Hancock RD, Jeffrey M, Dawson M, Bradley R. A novel progressive spongiform encephalopathy in cattle. Vet Rec 1987; 121:419 - 20; http://dx.doi.org/10.1136/vr.121.18.419; PMID: 3424605
- Williams ES, Young S. Chronic wasting disease of captive mule deer: a spongiform encephalopathy. J Wildl Dis 1980; 16:89 - 98; http://dx.doi.org/10.7589/0090-3558-16.1.89; PMID: 7373730
- Collinge J. Prion diseases of humans and animals: their causes and molecular basis. Annu Rev Neurosci 2001; 24:519 - 50; http://dx.doi.org/10.1146/annurev.neuro.24.1.519; PMID: 11283320
- Kujala P, Raymond CR, Romeijn M, Godsave SF, van Kasteren SI, Wille H, Prusiner SB, Mabbott NA, Peters PJ. Prion uptake in the gut: identification of the first uptake and replication sites. PLoS Pathog 2011; 7:e1002449; http://dx.doi.org/10.1371/journal.ppat.1002449; PMID: 22216002
- Robinson SJ, Samuel MD, O’Rourke KI, Johnson CJ. The role of genetics in chronic wasting disease of North American cervids. Prion 2012; 6:153 - 62; http://dx.doi.org/10.4161/pri.19640; PMID: 22460693
- Scott MR, Peretz D, Nguyen HO, Dearmond SJ, Prusiner SB. Transmission barriers for bovine, ovine, and human prions in transgenic mice. J Virol 2005; 79:5259 - 71; http://dx.doi.org/10.1128/JVI.79.9.5259-5271.2005; PMID: 15827140
- Stewart P, Campbell L, Skogtvedt S, Griffin KA, Arnemo JM, Tryland M, Girling S, Miller MW, Tranulis MA, Goldmann W. Genetic predictions of prion disease susceptibility in carnivore species based on variability of the prion gene coding region. PLoS One 2012; 7:e50623; http://dx.doi.org/10.1371/journal.pone.0050623; PMID: 23236380
- Vidal E, Fernández-Borges N, Pintado B, Ordóñez M, Márquez M, Fondevila D, Torres JM, Pumarola M, Castilla J. Bovine spongiform encephalopathy induces misfolding of alleged prion-resistant species cellular prion protein without altering its pathobiological features. J Neurosci 2013; 33:7778 - 86; http://dx.doi.org/10.1523/JNEUROSCI.0244-13.2013; PMID: 23637170
- Chianini F, Fernández-Borges N, Vidal E, Gibbard L, Pintado B, de Castro J, Priola SA, Hamilton S, Eaton SL, Finlayson J, et al. Rabbits are not resistant to prion infection. Proc Natl Acad Sci U S A 2012; 109:5080 - 5; http://dx.doi.org/10.1073/pnas.1120076109; PMID: 22416127
- Béringue V, Le Dur A, Tixador P, Reine F, Lepourry L, Perret-Liaudet A, Haïk S, Vilotte JL, Fontés M, Laude H. Prominent and persistent extraneural infection in human PrP transgenic mice infected with variant CJD. PLoS One 2008; 3:e1419; http://dx.doi.org/10.1371/journal.pone.0001419; PMID: 18183299
- Lewis PA, Tattum MH, Jones S, Bhelt D, Batchelor M, Clarke AR, Collinge J, Jackson GS. Codon 129 polymorphism of the human prion protein influences the kinetics of amyloid formation. J Gen Virol 2006; 87:2443 - 9; http://dx.doi.org/10.1099/vir.0.81630-0; PMID: 16847141
- van der Kamp MW, Daggett V. Pathogenic mutations in the hydrophobic core of the human prion protein can promote structural instability and misfolding. J Mol Biol 2010; 404:732 - 48; http://dx.doi.org/10.1016/j.jmb.2010.09.060; PMID: 20932979
- Kyle LM, John TR, Schätzl HM, Lewis RV. Introducing a rigid loop structure from deer into mouse prion protein increases its propensity for misfolding in vitro. PLoS One 2013; 8:e66715; http://dx.doi.org/10.1371/journal.pone.0066715; PMID: 23825561
- Tranulis MA, Osland A, Bratberg B, Ulvund MJ. Prion protein gene polymorphisms in sheep with natural scrapie and healthy controls in Norway. J Gen Virol 1999; 80:1073 - 7; PMID: 10211978
- Elsen JM, Amigues Y, Schelcher F, Ducrocq V, Andreoletti O, Eychenne F, Khang JV, Poivey JP, Lantier F, Laplanche JL. Genetic susceptibility and transmission factors in scrapie: detailed analysis of an epidemic in a closed flock of Romanov. Arch Virol 1999; 144:431 - 45; http://dx.doi.org/10.1007/s007050050516; PMID: 10226611
- Goldmann W, Hunter N, Foster JD, Salbaum JM, Beyreuther K, Hope J. Two alleles of a neural protein gene linked to scrapie in sheep. Proc Natl Acad Sci U S A 1990; 87:2476 - 80; http://dx.doi.org/10.1073/pnas.87.7.2476; PMID: 1969635
- Belt PB, Muileman IH, Schreuder BE, Bos-de Ruijter J, Gielkens AL, Smits MA. Identification of five allelic variants of the sheep PrP gene and their association with natural scrapie. J Gen Virol 1995; 76:509 - 17; http://dx.doi.org/10.1099/0022-1317-76-3-509; PMID: 7897344
- Goldmann W, Hunter N, Smith G, Foster J, Hope J. PrP genotype and agent effects in scrapie: change in allelic interaction with different isolates of agent in sheep, a natural host of scrapie. J Gen Virol 1994; 75:989 - 95; http://dx.doi.org/10.1099/0022-1317-75-5-989; PMID: 7909834
- Swietnicki W, Petersen RB, Gambetti P, Surewicz WK. Familial mutations and the thermodynamic stability of the recombinant human prion protein. J Biol Chem 1998; 273:31048 - 52; http://dx.doi.org/10.1074/jbc.273.47.31048; PMID: 9813003
- Rezaei H, Choiset Y, Eghiaian F, Treguer E, Mentre P, Debey P, Grosclaude J, Haertle T. Amyloidogenic unfolding intermediates differentiate sheep prion protein variants. J Mol Biol 2002; 322:799 - 814; http://dx.doi.org/10.1016/S0022-2836(02)00856-2; PMID: 12270715
- Rezaei H, Marc D, Choiset Y, Takahashi M, Hui Bon Hoa G, Haertlé T, Grosclaude J, Debey P. High yield purification and physico-chemical properties of full-length recombinant allelic variants of sheep prion protein linked to scrapie susceptibility. Eur J Biochem 2000; 267:2833 - 9; http://dx.doi.org/10.1046/j.1432-1033.2000.01347.x; PMID: 10806380
- Chen KC, Xu M, Wedemeyer WJ, Roder H. Microsecond unfolding kinetics of sheep prion protein reveals an intermediate that correlates with susceptibility to classical scrapie. Biophys J 2011; 101:1221 - 30; http://dx.doi.org/10.1016/j.bpj.2011.07.024; PMID: 21889460
- Ha T, Enderle T, Ogletree DF, Chemla DS, Selvin PR, Weiss S. Probing the interaction between two single molecules: fluorescence resonance energy transfer between a single donor and a single acceptor. Proc Natl Acad Sci U S A 1996; 93:6264 - 8; http://dx.doi.org/10.1073/pnas.93.13.6264; PMID: 8692803
- Oddershede L, Flyvbjerg H, Berg-Sorensen K. Single-molecule experiment with optical tweezers: improved analysis of the diffusion of the lambda-receptor in E-coli's outer membrane. J Phys Condens Matter 2003; 15:S1737 - 46; http://dx.doi.org/10.1088/0953-8984/15/18/307
- Lee GU, Chrisey LA, Colton RJ. Direct measurement of the forces between complementary strands of DNA. Science 1994; 266:771 - 3; http://dx.doi.org/10.1126/science.7973628; PMID: 7973628
- Stefureac R, Long YT, Kraatz HB, Howard P, Lee JS. Transport of alpha-helical peptides through alpha-hemolysin and aerolysin pores. Biochemistry 2006; 45:9172 - 9; http://dx.doi.org/10.1021/bi0604835; PMID: 16866363
- Meng H, Detillieux D, Baran C, Krasniqi B, Christensen C, Madampage C, Stefureac RI, Lee JS. Nanopore analysis of tethered peptides. J Pept Sci 2010; 16:701 - 8; http://dx.doi.org/10.1002/psc.1289; PMID: 20814890
- Stefureac R, Waldner L, Howard P, Lee JS. Nanopore analysis of a small 86-residue protein. Small 2008; 4:59 - 63; http://dx.doi.org/10.1002/smll.200700402; PMID: 18058890
- Stefureac RI, Lee JS. Nanopore analysis of the folding of zinc fingers. Small 2008; 4:1646 - 50; http://dx.doi.org/10.1002/smll.200800585; PMID: 18819138
- Madampage C, Tavassoly O, Christensen C, Kumari M, Lee JS. Nanopore analysis: An emerging technique for studying the folding and misfolding of proteins. Prion 2012; 6:116 - 23; http://dx.doi.org/10.4161/pri.18665; PMID: 22421211
- Tavassoly O, Lee JS. Methamphetamine binds to α-synuclein and causes a conformational change which can be detected by nanopore analysis. FEBS Lett 2012; 586:3222 - 8; http://dx.doi.org/10.1016/j.febslet.2012.06.040; PMID: 22771474
- Stefureac RI, Madampage CA, Andrievskaia O, Lee JS. Nanopore analysis of the interaction of metal ions with prion proteins and peptides. Biochem Cell Biol 2010; 88:347 - 58; http://dx.doi.org/10.1139/O09-176; PMID: 20453935
- Madampage CA, Andrievskaia O, Lee JS. Nanopore detection of antibody prion interactions. Anal Biochem 2010; 396:36 - 41; http://dx.doi.org/10.1016/j.ab.2009.08.028; PMID: 19699704
- Madampage CA, Määttänen P, Marciniuk K, Brownlie R, Andrievskaia O, Potter A, Cashman NR, Lee JS, Napper S. Binding of bovine T194A PrP(C) by PrP(Sc)-specific antibodies: potential implications for immunotherapy of familial prion diseases. Prion 2013; 7:301 - 11; http://dx.doi.org/10.4161/pri.25148; PMID: 23787697
- Paramithiotis E, Pinard M, Lawton T, LaBoissiere S, Leathers VL, Zou WQ, Estey LA, Lamontagne J, Lehto MT, Kondejewski LH, et al. A prion protein epitope selective for the pathologically misfolded conformation. Nat Med 2003; 9:893 - 9; http://dx.doi.org/10.1038/nm883; PMID: 12778138
- Li L, Napper S, Cashman NR. Immunotherapy for prion diseases: opportunities and obstacles. Immunotherapy 2010; 2:269 - 82; http://dx.doi.org/10.2217/imt.10.3; PMID: 20635933
- Hedlin PD, Cashman NR, Li L, Gupta J, Babiuk LA, Potter AA, Griebel P, Napper S. Design and delivery of a cryptic PrP(C) epitope for induction of PrP(Sc)-specific antibody responses. Vaccine 2010; 28:981 - 8; http://dx.doi.org/10.1016/j.vaccine.2009.10.134; PMID: 19925901
- Riek R, Wider G, Billeter M, Hornemann S, Glockshuber R, Wüthrich K. Prion protein NMR structure and familial human spongiform encephalopathies. Proc Natl Acad Sci U S A 1998; 95:11667 - 72; http://dx.doi.org/10.1073/pnas.95.20.11667; PMID: 9751723