Abstract
The discovery of tunnelling nanotubes (TNTs) and their proposed role in long intercellular transport of organelles, bacteria, and viruses have led us to examine their potential role during prion spreading. We have recently shown that these membrane bridges can form between neuronal cells, as well as between dendritic cells and primary neurons and that both endogenous and exogenous PrPSc appear to traffic through these structures between infected and non-infected cells. Furthermore, prion infection can be efficiently transmitted from infected dendritic cells to primary neurons only in co-culture conditions permissive for TNT formation. Therefore, we propose a role for TNTs during prion spreading from the periphery to the central nervous system (CNS). Here, we discuss some of the key steps where TNTs might play a role during prion neuroinvasion.
Prion diseases, or transmissible spongiform encephalopathies (TSEs), are fatal neurodegenerative disorders that have been found in a number of species, including scrapie in sheep, bovine spongiform encephalopathy in cattle (BSE), Chronic wasting disease in deer and Creutzfeldt-Jacob, the Gerstmann-Straüssler-Scheinker syndrome, fatal familial insomnia and kuru in humans (reviewed in ref. Citation1). Human TSEs can be sporadic, genetic or acquired by infection. A new variant of Creutzfeldt-Jakob disease (termed vCJD) was reported from the UK in 1996.Citation2 The majority of vCJD cases diagnosed to date resulted from a peripheral exposure via the consumption of BSE-contaminated food. Pathological features of TSE diseases can include gliosis, neuronal cell loss and spongiform changes, but the common feature of all members of this group of diseases is the build-up of an aberrant form of the host cellular protein PrPC, named PrPSc (from scrapie). The normal cellular isoform, PrPC, is an endogenous glycosylphosphatidyl inositol (GPI)-anchored protein present in numerous tissues in mammals, including neurons and lymphoid cells. While the exact function of PrPC remains unclear, evidence suggest putative roles in neuroprotection, cell adhesion and signal transduction (reviewed in refs. Citation3 and Citation4). According to the ‘protein-only hypothesis,’ the causative agents of prion diseases are proteinaceous infectious particles (‘prions’), which are composed essentially of misfolded PrPC, or PrPSc.Citation5,Citation6 Prions replicate through a molecular mechanism in which abnormally folded PrPSc acts as a catalyst and serves as a template to convert normal PrPC molecules into PrPSc.Citation5,Citation6 PrPSc differs from PrPC in the conformation of its polypeptide chain, which is enriched in β-sheets and is protease resistant. Although the conversion process is believed to have a predominant role in the pathogenesis of prion diseases, the cellular and molecular basis for the pathogenic conversion of PrP are still unknown.
Another important question is how PrPSc spreads to and within the brain. After oral exposure, PrPSc accumulates into lymphoid tissues, such as the spleen, lymph nodes or Peyer's patches, prior to neuroinvasion.Citation7–Citation9 The exact mechanisms and specific cells involved in the spreading from the gastrointestinal track to the lymphoid system and to the peripheral nervous system (PNS), leading to neuroinvasion of the CNS remain to be elucidated. However, a range of evidence suggests that the accumulation of PrPSc within lymphoid tissues is necessary for efficient neuroinvasion.Citation9–Citation11 In particular it has been shown that PrPSc accumulates first within follicular dendritic cells (FDCs)Citation12 and macrophages.Citation13 FDCs are stromal-differentiated cells in the germinal centres of activated lymphoid follicles. A number of studies have demonstrated that FDCs play a critical role during spreading of infection since their absence greatly impaires the neuroinvasion process.Citation8,Citation11,Citation14,Citation15 However, because FDCs are immobile cells, it is not clear how they acquire PrPSc and how it spreads from the FDCs to the PNS. FDCs and nerve synapses occupy different anatomical sitesCitation16,Citation17 and therefore the lack of physical contact between the gut and FDCs and between FDCs and the nerve periphery imply the presence of intermediate mechanisms for the transport of PrPSc. Dendritic cells (DCs) have been proposed to play a critical role in the transport of PrPSc from the gut to FDCs.Citation18 DCs function as sentinels for incoming pathogens. Bone-marrow dendritic cells (BMDCs) are migratory cells that are able to transport proteins within Peyer's patches and into mesenteric lymph nodes.Citation19 Interestingly, mucosal dendritic cells which play a role in the transport of intestinal antigen for presentation to Peyer's patches and to mesenteric lymph nodes, can also extend trans-epithelial dendrites to directly sample bacteria in the gut.Citation20,Citation21 However, the transport of PrPSc from FDCs to the PNS remains controversial and evidence for a direct role of DCs during this process has been debated.Citation22,Citation23 Several mechanisms have been proposed for the intercellular transfer of PrPSc, including cell-cell contact, transfer via exosomes or by GPI-painting.Citation24–Citation26 For example, similar to other types of pathogens such as HIV-1, which was proposed to follow the “exosomal” pathway to be released from the cells,Citation27 it has been shown that the supernatant of prion infected cells contain large amount of PrPSc in membranous vesicles known as exosomes.Citation25,Citation28 Thus, it was suggested that exosomes might be a way to spread prion infection in vivo.Citation25,Citation28 Recently, a different type of vesicles known as plasma membrane-derived microvesicles, were also described as a potential spreading mechanism during neuroinvasion.Citation29
In 2004, Rustom and colleagues discovered a new mechanism of long distance intercellular communication in mammalian cells, called tunnelling nanotubes (TNTs).Citation30 TNTs are transient, long, actin-rich projections that allow for long-distance intercellular communication (reviewed in refs. Citation31–Citation33). TNT-like structures have been described to form in vitro between numerous cell types, including neuronal and immune cells.Citation30,Citation34,Citation35 These studies demonstrated that TNT-like structures formed bridges or channels between distant cells that can be used to transfer material between cells, including Lysotracker positive or endosomal vesicles, calcium fluxes, bacteria or viruses through their cytoplasms or along the surface of the nanotubes.Citation31–Citation33 Interestingly, a model GPI-anchored protein, GFP-GPI, was found to move at the surface of these tubesCitation34 and while studying the neuritic transport of prions in neuronal cells, Magalhães and colleagues noticed a strong correlation between internalized PrP-res and Lysotracker positive vesicles in neurites,Citation36 suggesting that PrP-res might also be able to transfer through TNTs during prion cell-cell spreading.
The results from the studies mentioned above and random observations of TNT-like structures in neuronal model cell cultures first led us to study whether these structures could in fact provide an efficient mechanism for prion cell-cell spreading.Citation37 We initially characterized TNT-like structures in the mouse catecholaminergic neuronal cell line, Cath.a-Differentiated cells (CAD cells) a well-recognized neuronal cell model for prion infection.Citation38 Under our culturing conditions, over 40% of the CAD cells could efficiently form actin-rich TNT-like structures between differentially labelled cell populations. In CAD cells, these nanotubes were very heterogeneous, both in length and in diameters. Indeed, TNT-like structures had lengths ranging from 10 to 80 µm and while over 70% of the nanotubes had diameters smaller than 200 nm, the remaining TNT-like structures had larger diameters (200 to 800 nm). We demonstrated that vesicles of lysosomal origins, a fluorescent form of PrP (GFP-PrP), infectious Alexa-PrPSc, as well as both endogenous and exogenous PrPSc could traffic within TNTs between neuronal cells (). The lysosomal and GFP-PrP vesicles observed to move through TNTs had a directed movement with a speed in the range of actin-mediated motors,Citation37 consistent with previous studies suggesting the involvement of an actomyosin-dependent transport.Citation39 Interestingly, active transfer of endogenous PrPSc, lysosomal or GFP-PrP vesicles occurred through TNTs with larger diameters, suggesting distinct roles for the different TNT-like structures observed.Citation37 These results do not seem to be specific to CAD cells since the transfer of GFP-PrP throught TNTs was observed in different types of transfected cells, including HEK293 cells (unpublished data). Furthermore, these results were in agreement with previous observations by Onfelt and colleagues showing the presence of a fluorescent GPI model protein (GFP-GPI) in TNTs formed between EBV-transformed human B cellsCitation34 suggesting that different GPI-anchored proteins can be transferred along the surface and inside vesicles within TNTs. In order to determine the relevance of this type of intercellular communication in the case of prion diseases, it was necessary to evaluate the trafficking of the pathological form of PrP (PrPSc) within TNTs, by analyzing the transfer of endogenous PrPSc between chronically infected ScCAD cells and non-infected CAD cells. By immunofluorescence after guanidium treatment, endogenous PrPSc was found inside TNTs and in the cytoplasm of recipient non-infected CAD cells. Similar to exogenous PrPSc, endogenous PrPSc particles were not present in non-infected CAD cells not in contact with ScCAD cells after overnight co-cultures, thus excluding exosomal transfer or protein shedding.Citation37 Similarly, no transfer was observed between cells in direct contact with one another or upon treatment with latrunculin, which inhibits TNT formation. Strikingly, the transfer of endogenous PrPSc was visible only when TNTs were present, demonstrating that in vitro, PrPSc can efficiently exploit TNTs to spread between cells of neuronal origin. These data suggested that TNTs could be a mechanism for prion spreading within the cells of the CNS.
Interestingly, DCs were shown to form networks of TNTs both in vitroCitation40 and in vivo.Citation41 In an elegant study, Watkins and Salter demonstrated that DCs could propagate calcium flux upon cell stimulation to other cells hundreds of microns away through TNTs, both between DCs and between DCs and THP-1 monocytes.Citation40 These data suggested the possibility that DCs could form tubular connections with neuronal cells in order to transport PrPSc to the PNS via TNTs. Using BMDCs in co-cultures with both cerebellar granular neurons (CGNs) and primary hippocampal neurons, we showed that BMDCs could form networks of TNTs with both types of neurons. Furthermore, these TNTs appeared to be functional, allowing for the transport of Lysotracker positive vesicles and infectious Alexa-PrPSc between loaded BMDCs and primary neurons, suggesting that DCs could transfer the infectious prion agent to primary neuronal cultures through TNTs. By using filters and conditions unfavorable for other mechanisms of transport, we found that moRK13 cells,Citation28 as well as CGNs (unpublished data), could be infected by co-cultures with BMDCs loaded with infectious brain homogenate.Citation37 Overall, these data indicate that TNTs could be an efficient mechanism of prion transmission between immune cells and neuronal cells, as well as between neuronal cultures. Since DCs can interact with peripheral neurons,Citation42 we propose that TNTs could be involved in the process of neuroinvasion at multiple stages, from the peripheral site of entry to the PNS by neuroimmune interactions with DCs, allowing neurons to retrogradely transport prions to the CNS, and within the CNS ().
Recently, it was demonstrated that the distance between FDCs and the neighbouring PNS was critical for prion neuroinvasion.Citation43 Indeed, in the spleen of CD19−/− mice, FDC networks were found to be 50% closer to the nerve fibers compared to wild-type mice.Citation43 The authors suggested that the increase in prion spreading efficiency in these mice was directly dependent on the reduction in the distance between the FDC networks and the PNS in these mice. These results would be consistent with a mechanism of transfer such as exosomes release. However, shortening the distance between FDCs and the PNS would also reduce the route of transport that mobile cells would have to travel and increase the chances for transfer of prions to the PNS, resulting in an increase in prion spreading efficiency. While the importance of FDCs in prion replication during the spreading to the CNS seems to be clear,Citation11,Citation14,Citation15 their specific role in the transfer of prions and their possible interactions with other mobile cells are much more debated.Citation22,Citation23 In order to bridge the gap between FDCs and the PNS, a role for DCs as possible carriers of PrPSc has been postulated. Aucouturier and colleagues have previously shown, using RAG-1−/− mice, which are deficient in FDCs, that CD11c+ DCs infected with 139A were able to carry prion infection to the CNS, without accumulation and replication in lymphoid organs,Citation22 thus suggesting that DCs are able to transport prions from the periphery to nerve cells. Recently, however, another study using TNFR1−/− mice, deficient for FDCs, suggested that DCs were unlikely candidates in the transport of prion to the PNS.Citation23 In this study, the authors showed that in TNFR1−/− mice, ME7 or 139A infected DCs were inefficient in transferring infection to the PNS. The authors suggested that the differences between the results obtained with RAG-1−/− mice and TNFR1−/− mice could be due to the differences in the levels of innervation of the spleen in RAG-1−/−mice compared to TNFR1−/− mice. They suggested that in RAG-1−/− mice, DCs could spread prion infection because their spleens are highly innervated, compared to TNFR1−/− or wild-type mice, therefore increasing the propensity of DCs to encounter nerve cells and tranfer the prion agents. Because of the reduction in the levels of innervation in the spleens of wild-type mice, the authors concluded that DCs are unlikely candidate for the transport of prions directly to the PNS [see (1) in ]. However, since these studies are using mice deficient for FDCs, it remains unclear what type of interactions might occur between FDCs and DCs, and how DCs might be able to transport prions from FDCs to the PNS in wild-type mice [see (2) in ]. Indeed, both studies show that under the right circumstances, DCs can interact with nerve cells, similar to what was recently shown in infected miceCitation42 and in agreement with our findings that DCs can form TNTs with neurons.Citation37 Within this scenario, it is clear that to determine the specific role of DCs during the spreading of prions from the gut to the PNS, the transfer mechanisms between DCs and other cell types, especially FDCs and peripheral neurons, need to be better characterized.
Overall, these in vitro data strongly point toward TNTs as one possible mechanism of prion spreading. The next step will be to identify these structures in vivo and to determine whether prion spreading in vivo is the result of passive mechanisms, such as exosome release, active intercellular transport along and within TNTs or whether prions will use any means available to reach their targets. Recently, TNT-like structures were imaged in a mouse cornea,Citation41 suggesting that while challenging, the visualization of in vivo trafficking of prions in lymphoid tissues such as in lymph nodes or in the spleen as well as in brain organotypic cultures might be possible and could be used to reveal the presence of TNTs.
The discovery of the existence of nanotubular membrane bridges in vitro has opened-up a new field of research. Channels, called plasmodesmata,Citation44 connecting plant cells have long been known to play crucial roles in the transport of nutrients, molecules and signals during development and some of their functions were recently compared with some of the recently proposed functions of TNTs.Citation45 Furthermore, in vivo long, actin-rich filopodia like structures were found to be crucial during development.Citation46–Citation49 For example, these structures exist in developing sea urchin embryos and were proposed to play a role in signalling and patterning during gastrulation.Citation47 Similar roles were proposed for thin filopodia-like structures observed during dorsal closure in drosophila.Citation49 In addition, TNT-like structures were observed in the mouse cornea between DCs and were shown to increase under inflammatory conditions.Citation41 The authors postulated that these TNT-like structures could play a role in Ag-specific signalling, especially as a response to eye inflammation. Therefore, the possibility that TNTs might play numerous roles during cell development, in the immune system and as conduits for the spreading of pathogens could lead to major changes in the way we view animal cell interactions. Specifically, understanding how pathogens usurp these cellular connections to spread could allow for the screening and the identification of new therapeutic inhibitors. To this aim, characterizing the basic mechanism of TNT formation within cell model systems will be necessary to improve the knowledge of TNTs in general, to analyze the transfer of pathogens more specifically, and to identify key molecules during this process. In the case of prions, whether they hijack nanotubes to spread between cells or whether prions increase the formation of filopodia and TNT-like structures similar to some virusesCitation33,Citation50 and/or the efficiency of transfer remain to be determined. Overall, in this specific field, the constant improvement of cell imaging techniques and the emergence of imaging tools to study prion spreadingCitation36,Citation37,Citation51–Citation53 could lead to exciting new insights both in the physiology of these intercellular connections and in the pathology of these devastating diseases.
Figures and Tables
Figure 1 Endogenous PrPSc transfer from ScCAD cells to CAD cells via TNTs. Endogenous PrPSc is found in punctate structures inside TNTs and in the cytoplasms of recipient cells. CAD cells were transfected with Cherry-PLAP (red) and co-cultured with ScCAD for 24 h. Cells were fixed, treated with Gnd and immunostained for PrP using SAF32 Ab (green). (A) Merge projection of Z-stacks obtained with a confocal Andor spinning-disk microscope. (B) Three-dimensional reconstruction of (A) using OsiriX software. (C) Zoom in on TNT-like structures. PrPSc is found in vesicular structures inside TNTs and in the cytoplasm of the recipient non-infected CAD cells (see blue arrow heads). Scale bar represents 10 µm.
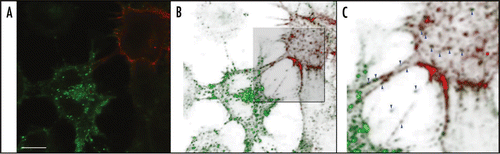
Figure 2 Transport of PrPSc via TNTs, an alternative spreading mechanism during neuroinvasion. Studies in our laboratory suggest that TNTs allow for the intracellular transport of PrPSc between dendritic cells and neurons and between neurons (see inset). The exact mechanism of transport remains to be determined. For instance, it is still not clear, whether PrPSc is strictly transported within endocytic vesicles, or whether it can slide along the surface or be transported as aggregosomes within the tubes. Similarly, the types of motors used, as well as the possible gated mechanisms to enter the recipient cells are not known. Because of the high propensity of DCs to form TNTs with different cell types, we propose that TNTs could play important roles in delivering PrPSc to the proper cell types along the neuroinvasion route. For instance, DCs could deliver PrPSc from the peripheral entry sites to FDCs in the secondary lymphoid tissues (2) or in a less efficient manner, they might occasionally directly transport PrPSc to the PNS (1). They could also bridge the immobile FDC networks and the PNS (3), since we have shown that DCs can form TNTs with nerve cells. Finally, once PrPSc has reached its final destination within the CNS, TNTs might play a final role in the spreading of PrPSc within the brain between neurons and possibly between neuronal cells and astrocytes (4).
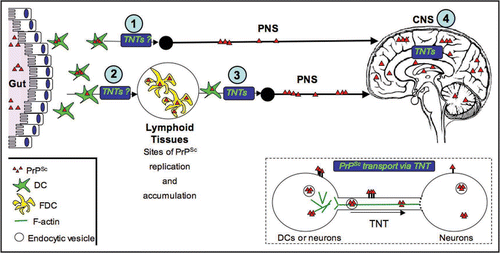
Acknowledgements
We thank Christelle Langevin and Benoit Vanderperre for critical reading of the manuscript and Anna Caputo who performed the experiment shown in . K.G. is supported by the Pasteur Foundation Fellowship Program. Support for this work was provided by EU grant (Strainbarrier [FP6 Contract No° 023183] (Food)) and Alliance Biosecure.
References
- Mabbott NA, MacPherson GG. Prions and their lethal journey to the brain. Nat Rev Microbiol 2006; 4:201 - 211
- Will RG, Ironside JW, Zeidler M, Cousens SN, Estibeiro K, Alperovitch A, et al. A new variant of Creutzfeldt-Jakob disease in the UK. Lancet 1996; 347:921 - 925
- Aguzzi A, Sigurdson C, Heikenwaelder M. Molecular mechanisms of prion pathogenesis. Annu Rev Pathol 2008; 3:11 - 40
- Westergard L, Christensen HM, Harris DA. The cellular prion protein (PrP(C)): its physiological function and role in disease. Biochim Biophys Acta 2007; 1772:629 - 644
- Prusiner SB. Novel proteinaceous infectious particles cause scrapie. Science 1982; 216:136 - 144
- Harris DA. Cellular biology of prion diseases. Clin Micr Rev 1999; 12:429 - 444
- Beekes M, McBride PA. Early accumulation of pathological PrP in the enteric nervous system and gut-associated lymphoid tissue of hamsters orally infected with scrapie. Neurosci Lett 2000; 278:181 - 184
- Mabbott NA, Young J, McConnell I, Bruce ME. Follicular dendritic cell dedifferentiation by treatment with an inhibitor of the lymphotoxin pathway dramatically reduces scrapie susceptibility. J Virol 2003; 77:6845 - 6854
- Prinz M, Huber G, Macpherson AJ, Heppner FL, Glatzel M, Eugster HP, et al. Oral prion infection requires normal numbers of Peyer's patches but not of enteric lymphocytes. Am J Pathol 2003; 162:1103 - 1111
- Fraser H, Dickinson AG. Pathogenesis of scrapie in the mouse: the role of the spleen. Nature 1970; 226:462 - 463
- Mohan J, Bruce ME, Mabbott NA. Follicular dendritic cell dedifferentiation reduces scrapie susceptibility following inoculation via the skin. Immunol 2005; 114:225 - 234
- Kitamoto T, Muramoto T, Mohri S, Doh-Ura K, Tateishi J. Abnormal isoform of prion protein accumulates in follicular dendritic cells in mice with Creutzfeldt-Jakob disease. J Virol 1991; 65:6292 - 6295
- Jeffrey M, McGovern G, Goodsir CM, Brown KL, Bruce ME. Sites of prion protein accumulation in scrapie-infected mouse spleen revealed by immunoelectron microscopy. J Pathol 2000; 191:323 - 332
- Mabbott NA, Mackay F, Minns F, Bruce ME. Temporary inactivation of follicular dendritic cells delays neuroinvasion of scrapie. Nature Med 2000; 6:719 - 720
- Montrasio F, Frigg R, Glatzel M, Klein MA, Mackay F, Aguzzi A, Weissmann C. Impaired prion replication in spleens of mice lacking functional follicular dendritic cells. Science 2000; 288:1257 - 1259
- Defaweux V, Dorban G, Demonceau C, Piret J, Jolois O, Thellin O, et al. Interfaces between dendritic cells, other immune cells, and nerve fibres in mouse Peyer's patches: potential sites for neuroinvasion in prion diseases. Microsc Res Tech 2005; 66:1 - 9
- Felten DL. Neurotransmitter signaling of cells of the immune system: important progress, major gaps. Brain Behav Immun 1991; 5:2 - 8
- Huang FP, Farquhar CF, Mabbott NA, Bruce ME, MacPherson GG. Migrating intestinal dendritic cells transport PrPSc from the gut. J Gen Virol 2002; 83:267 - 271
- Banchereau J, Briere F, Caux C, Davoust J, Lebecque S, Liu YJ, et al. Immunobiology of dendritic cells. Annu Rev Immunol 2000; 18:767 - 811
- Rescigno M, Urbano M, Valzasina B, Francolini M, Rotta G, Bonasio R, et al. Dendritic cells express tight junction proteins and penetrate gut epithelial monolayers to sample bacteria. Nat Immunol 2001; 2:361 - 367
- Niess JH, Brand S, Gu X, Landsman L, Jung S, McCormick BA, et al. CX3CR1-mediated dendritic cell access to the intestinal lumen and bacterial clearance. Science 2005; 307:254 - 258
- Aucouturier P, Geissmann F, Damotte D, Saborio GP, Meeker HC, Kascsak R, et al. Infected splenic dendritic cells are sufficient for prion transmission to the CNS in mouse scrapie. J Clin Invest 2001; 108:703 - 708
- Raymond CR, Mabbott NA. Assessing the involvement of migratory dendritic cells in the transfer of the scrapie agent from the immune to peripheral nervous systems. J Neuroimmunol 2007; 187:114 - 125
- Kanu N, Imokawa Y, Drechsel DN, Williamson RA, Birkett CR, Bostock CJ, Brockes JP. Transfer of scrapie prion infectivity by cell contact in culture. Curr Biol 2002; 12:523 - 530
- Fevrier B, Vilette D, Archer F, Loew D, Faigle W, Vidal M, et al. Cells release prions in association with exosomes. Proc Natl Acad Sci USA 2004; 101:9683 - 9688
- Baron GS, Magalhães AC, Prado MA, Caughey B. Mouse-adapted scrapie infection of SN56 cells: greater efficiency with microsome-associated versus purified PrP-res. J Virol 2006; 80:2106 - 2117
- Gould SJ, Booth AM, Hildreth JE. The Trojan exosome hypothesis. Proc Natl Acad Sci USA 2003; 100:10592 - 10597
- Vella LJ, Sharples RA, Lawson VA, Masters CL, Cappai R, Hill AF. Packaging of prions into exosomes is associated with a novel pathway of PrP processing. J Pathol 2007; 211:582 - 590
- Mattei V, Barenco MG, Tasciotti V, Garofalo T, Longo A, Boller K, et al. Paracrine diffusion of PrP(C) and propagation of prion infectivity by plasma membrane-derived microvesicles. PLoS ONE 2009; 4:5057
- Rustom A, Saffrich R, Markovic I, Walther P, Gerdes HH. Nanotubular highways for intercellular organelle transport. Science 2004; 303:1007 - 1010
- Gurke S, Barroso JF, Gerdes HH. The art of cellular communication: tunneling nanotubes bridge the divide. Histochem Cell Biol 2008; 129:539 - 550
- Davis DM, Sowinski S. Membrane nanotubes: dynamic long-distance connections between animal cells. Nat Rev Mol Cell Biol 2008; 9:431 - 436
- Sherer NM, Mothes W. Cytonemes and tunneling nanotubules in cell-cell communication and viral pathogenesis. Trends Cell Biol 2008; 18:414 - 420
- Onfelt B, Nedvetzki S, Yanagi K, Davis DM. Cutting edge: Membrane nanotubes connect immune cells. J Immunol 2004; 173:1511 - 1513
- Sherer NM, Lehmann MJ, Jimenez-Soto LF, Horensavitz C, Pypaert M, Mothes W. Retroviruses can establish filopodial bridges for efficient cell-to-cell transmission. Nat Cell Biol 2007; 9:310 - 315
- Magalhães AC, Baron GS, Lee KS, Steele-Mortimer O, Dorward D, Prado MA, et al. Uptake and neuritic transport of scrapie prion protein coincident with infection of neuronal cells. J Neurosci 2005; 25:5207 - 5216
- Gousset K, Schiff E, Langevin C, Marijanovic Z, Caputo A, Browman DT, et al. Prions hijack tunnelling nanotubes for intercellular spread. Nat Cell Biol 2009; 11:328 - 336
- Mahal SP, Baker CA, Demczyk CA, Smith EW, Julius C, Weissmann C. Prion strain discrimination in cell culture: the cell panel assay. Proc Natl Acad Sci USA 2007; 104:20908 - 20913
- Gerdes HH, Carvalho RN. Intercellular transfer mediated by tunneling nanotubes. Curr Opin Cell Biol 2008; 20:470 - 475
- Watkins SC, Salter RD. Functional connectivity between immune cells mediated by tunneling nanotubules. Immunity 2005; 23:309 - 318
- Chinnery HR, Pearlman E, McMenamin PG. Cutting edge: membrane nanotubes in vivo: a feature of MHC class II+ cells in the mouse cornea. J Immunol 2008; 180:5779 - 5783
- Dorban G, Defaweux V, Demonceau C, Flandroy S, Van Lerberghe PB, Falisse-Poirrier N, et al. Interaction between dendritic cells and nerve fibres in lymphoid organs after oral scrapie exposure. Virchows Arch 2007; 451:1057 - 1065
- von Poser-Klein C, Flechsig E, Hoffmann T, Schwarz P, Harms H, Bujdoso R, et al. Alteration of B-cell subsets enhances neuroinvasion in mouse scrapie infection. J Virol 2008; 82:3791 - 3795
- Zambryski P, Crawford K. Plasmodesmata: gatekeepers for cell-to-cell transport of developmental signals in plants. Annu Rev Cell Dev Biol 2000; 16:393 - 421
- Gerdes HH, Bukoreshtliev NV, Barroso JF. Tunneling nanotubes: a new route for the exchange of components between animal cells. FEBS Lett 2007; 581:2194 - 2201
- Ramirez-Weber FA, Kornberg TB. Cytonemes: cellular processes that project to the principal signaling center in Drosophila imaginal discs. Cell 1999; 97:599 - 607
- Miller J, Fraser SE, McClay D. Dynamics of thin filopodia during sea urchin gastrulation. Development 1995; 121:2501 - 2511
- Salas-Vidal E, Lomeli H. Imaging filopodia dynamics in the mouse blastocyst. Dev Biol 2004; 265:75 - 89
- Millard TH, Martin P. Dynamic analysis of filopodial interactions during the zippering phase of Drosophila dorsal closure. Development 2008; 135:621 - 626
- Eugenin EA, Gaskill PJ, Berman JW. Tunneling nanotubes (TNT) are induced by HIV-infection of macrophages: a potential mechanism for intercellular HIV trafficking. Cell Immunol 2009; 254:142 - 148
- Rutishauser D, Mertz KD, Moos R, Brunner E, Rülicke T, Calella AM, Aguzzi A. The comprehensive native interactome of a fully functional tagged prion protein. PLoS ONE 2009; 4:4446
- Taguchi Y, Shi ZD, Ruddy B, Dorward DW, Greene L, Baron GS. Specific biarsenical labeling of cell surface proteins allows fluorescent- and biotin-tagging of amyloid precursor protein and prion proteins. Mol Biol Cell 2009; 20:233 - 244
- Marijanovic Z, Caputo A, Campana V, Zurzolo C. Identification of the intracellular site of prion conversion. PLoS Pathogen 2009; 5:1000426