Abstract
Prion protein, PrPC, is a glycoprotein that is expressed on the cell surface beginning with the early stages of embryonic stem cell differentiation. Previously, we showed that ectopic expression of PrPC in human embryonic stem cells (hESCs) triggered differentiation toward endodermal, mesodermal, and ectodermal lineages, whereas silencing of PrPC suppressed differentiation toward ectodermal but not endodermal or mesodermal lineages. Considering that PrPC might be involved in controlling the balance between cells of different lineages, the current study was designed to test whether PrPC controls differentiation of hESCs into cells of neuron-, oligodendrocyte-, and astrocyte-committed lineages. PrPC was silenced in hESCs cultured under three sets of conditions that were previously shown to induce hESCs differentiation into predominantly neuron-, oligodendrocyte-, and astrocyte-committed lineages. We found that silencing of PrPC suppressed differentiation toward all three lineages. Similar results were observed in all three protocols, arguing that the effect of PrPC was independent of differentiation conditions employed. Moreover, switching PrPC expression during a differentiation time course revealed that silencing PrPC expression during the very initial stage that corresponds to embryonic bodies has a more significant impact than silencing at later stages of differentiation. The current work illustrates that PrPC controls differentiation of hESCs toward neuron-, oligodendrocyte-, and astrocyte-committed lineages and is likely involved at the stage of uncommitted neural progenitor cells rather than lineage-committed neural progenitors.
Abbreviations
PrPC | = | normal, cellular isoform of the prion protein |
ESCs | = | embryonic stem cells |
hESCs | = | human ESCs |
Syn | = | synapsin I |
TH | = | tyrosine hydroxylase |
Olig1 | = | a marker of oligodendrocyte-committed lineages |
GFAP | = | glial fibrillary acidic protein |
Tet | = | tetracycline |
TetR | = | tetracycline repressor |
Lenti-TetR | = | lentiviral vector expressing tetracycline repressor |
Lenti-ShPrPC | = | lentiviral vector expressing short hairpin RNA against PrPC |
Lenti-ShScram | = | lentiviral vector expressing scrambled shRNA |
hES+TetR+ShPrPC | = | hESCs transfected with Lenti-TetR and Lenti-ShPrPC |
hES+TetR+ShScram | = | hESCs transfected with Lenti-TetR and Lenti-ShScram |
bFGF | = | basic fibroblast growth factor |
MEFs | = | mouse embryonic fibroblasts |
NIM | = | neural induction medium |
NPM | = | neural proliferation medium |
NDM | = | neuronal differentiation medium |
MEF-CM | = | mouse embryonic feeder-conditioned medium |
GRM | = | glial restrictive medium |
EFG | = | epidermal growth factor |
RA | = | retinoic acid |
EBs | = | embryoid bodies |
CNTF | = | ciliary neurotrophic factor |
Introduction
Conversion of the cellular prion protein (PrP) into self-propagating, β-sheet rich, aggregated state, PrPSc, underlies several lethal transmissible neurodegenerative diseases in mammals, including Creutzfeldt-Jakob disease and bovine spongiform encephalopathy.Citation1 The normal, cellular isoform of the prion protein, PrPC, is a sialoglycoprotein that is attached to the cell membrane via a C-terminal glycosylphosphatidyl-inositol anchor and has two N-linked glycans.Citation2,3 While PrPC is expressed in various tissues and organs, the highest level of expression has been found in cells of the central nervous system and in spermatogenetic cells.Citation4-6
The spectrum of proposed biological functions of PrPC has been expanding rapidly over the last decade. While several independent studies did not observed any deleterious effects in PrPC knock out mice,Citation7,8 deficiency in one of the two genes encoding PrPC in zebrafish led to an early developmental arrests.Citation9 To date, the list of possible PrPC functions includes those related to its Cu2+-binding properties such as Cu2+ sensing, Cu2+ transporting or acting as superoxide dismutase,Citation10–13 or activities not directly linked to Cu2+-binding such as signal transduction,Citation14 neuroprotectionCitation15–19 or regulation of the cell cycle.Citation20,21 Yet another set of PrPC activities that has been under rapid expansion in recent years are likely related to PrPC N-linked glycosylation and sialylation. They include neurotrophic activities,Citation19,22,23 cell adhesion,Citation9,Citation23-25 and cell proliferation and differentiation.Citation19,21,Citation26-31 The proportion of di- vs. mono-, and unglycosylated PrPC glycoforms was found to increase in the course of neuronal differentiation, as well as upon an increase in the density of cells cultured in vitro.Citation32,33 The studies that used PrPC knock out and overexpresser mice revealed that PrPC expression in multipotent neural precursor cells correlates with differentiation of precursors into mature neurons during neural development and adult neurogenesis.Citation26 Cross-talk between PrPC and the neural cell adhesion molecule NCAM was pointed out as critical for differentiation of neural stem cells into neurons.Citation34 In support of the hypothesis that PrPC is involved in differentiation of neural precursor cells,Citation26 PrPC was found on growing axons during development and along fiber bundles that contain elongating axons in the adult brain.Citation22,35 Moreover, PrPC was also found to induce polarization, synapse development and neuritogenesis in embryonic neuron cultures.Citation18,36 Recently, ablation of PrPC expression was found to cause chronic demyelinating polyneuropathy suggesting that PrPC is required for myelin maintenance.Citation37
Several studies illustrated that the involvement of PrPC in stem cell proliferation and differentiation is broader then just guiding differentiation of neural stem cells. PrPC was found on the surface of hematopoietic stem cells and proposed to be important for their self-renewal.Citation27 Moreover, studies conducted using PrPC knock out mice revealed that PrPC is important for regeneration of adult muscle tissue and guides the proliferation and differentiation of myogenic precursor cells.Citation38 Extremely high levels of Prnp (prion protein gene) mRNA were found in Sertoli cells that support development and high proliferating activity of spermatogonial stem cells.Citation6
In part due to limitations in usage of human embryonic stem cells, the vast majority of previous work on elucidating the role of PrPC in stem cell differentiation was limited to animal models or animal-derived cell lines. To test whether PrPC plays a role in early human embryogenesis, our previous studies examined the effects of recombinant PrP or the impact of PrPC suppression or overexpression on human embryonic stem cells (hESCs) during spontaneous differentiation.Citation21,28 hESCs are pluripotent cells with high self-renewal and proliferation activities that can be differentiated into any cell type of the three germ layers and subsequently any tissue.Citation39 The developmental sequence of hESCs embryonic bodies (EB) resembles the process of human embryogenesis.Citation40 Using a panel of lentiviral vectors we previously found that PrPC is involved in key cellular activities that determine the status of hESCs: (1) it regulates the cell cycle, (2) it controls the cellular switch between self-renewal and differentiation, and (3) contributes to regulating a differentiation balance between the lineages of the three germ layers, endoderm, mesoderm and ectoderm.Citation21 In particular, we found that silencing of PrPC under spontaneous differentiation conditions selectively suppressed differentiation toward ectodermal progenitors without affecting differentiation toward endodermal and mesodermal progenitors.
The current study asks the question whether PrPC selectively controls differentiation of hESCs into cells of three neural lineages: neuronal cells, astrocytes and oligodendrocytes. Using three protocols for differentiating hESCs into predominantly neuronal, oligodendrocyte and astrocyte lineages, we found that suppression of PrPC expression delays differentiation of all three lineages. Switching PrPC expression on and off during early differentiation revealed that PrPC expression during the very early stages that correspond to embryonic bodies has a more significant impact than silencing PrPC at the later stages. The extent of differentiation delay was found to be roughly proportional to the total length during which the expression of PrPC was suppressed. Together with previous studies, this work illustrates that PrPC is involved in controlling a balance between differentiation toward three germ layers, but not within neural lineages. The current work also suggests that PrPC is involved at the early stages of neural differentiation, perhaps, at the stage of uncommitted neural progenitor cells rather than lineage-committed progenitors.
Results
Silencing of PrPC delays differentiation of neuronal, oligodendrocytic, and astrocytic lineages
To examine the effect of PrPC on differentiation of hESCs toward three neural lineages, we employed an hESC line expressing inducible short hairpin RNA that suppresses PrPC expression under control of a tetracycline repressor (designated as Sup). As controls, an hESC line that expresses only the tetracycline repressor (designated as C1) or an hESC line that expresses inducible scrambled short hairpin RNA under control of the tetracycline repressor (designated as C2) were used. Three experimental protocols that were previously shown to induce differentiation of hESCs into predominantly neuronal cells, oligodendrocytes and astrocytes were employed.Citation41–43 In all three protocols, PrPC expression was suppressed starting from day zero and the suppression was continued until the experimental end-point, day 40.
As judged from cell shape and growing patterns, PrPC silencing delayed differentiation of hESCs conducted according to all three protocols. Notable differences in cell morphology between control lines and hESCs with suppressed PrPC expression were observed by the day 30 regardless of the differentiation protocol employed (, , and ). By the day 40, most of hESCs with silenced PrPC remained aggregated and lacked well-defined cell shapes, whereas cells in control groups acquired well-defined shape, a sign of differentiation (, , and ). By the day 40 control lines subjected to neuro-, oligodendrocyte- or astrocyte-specific differentiation protocols expressed tyrosine hydroxylase (TH, a marker of neuronal cells), Olig1, (a marker of oligodendrocyte-committed lineages) or glial fibrillary acidic protein (GFAP, a marker of astrocyte-commited lineages) across cell populations, respectively (, , and ). hESC lines with silenced PrPC expressed substantially lower amounts of protein markers specific for neuronal, oligodendrocytic or astrocytic lineages than in corresponding control cell groups (, , and ).
Figure 1. Suppression of PrPC delays differentiation into neuronal cells. (A) Phase-contrast images of hESCs with suppressed PrPC-expression (Sup) and in two control lines (C1 and C2) taken at 10th, 20th, 30th, and 40th day of differentiation. (B) Immunostaining for PrPC (red) and TH (green) on 40th day of differentiation. Hoechst 33342 was used for staining of nuclei (blue). Scale bar = 50 μm. (C) Quantification and statistical analyses of PrPC- and TH-positive cells on 40th day of differentiation. The data represent a mean ± SD from three independent experiments for each cell line. Statistical significance was determined by Student's t test: *, P < 0.05.
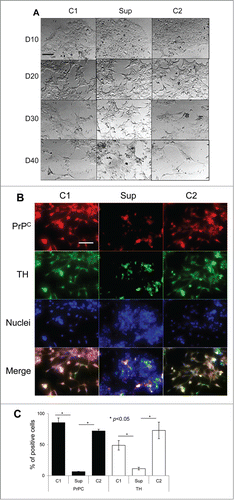
Figure 2. Suppression of PrPC delays differentiation into oligodendrocytes. (A) Phase-contrast images of hESCs with suppressed PrPC-expression (Sup) and in two control lines (C1 and C2) taken at 10th, 20th, 30th, and 40th day of differentiation. (B) Immunostaining for PrPC (red) and Olig1 (green) on 40th day of differentiation. Hoechst 33342 was used for staining of nuclei (blue). Scale bar = 50 μm. (C) Quantification and statistical analyses of PrPC- and Olig 1-positive cells on 40th day of differentiation. The data represent a mean ± SD from three independent experiments for each cell line. Statistical significance was determined by Student's t test: *, P < 0.05.
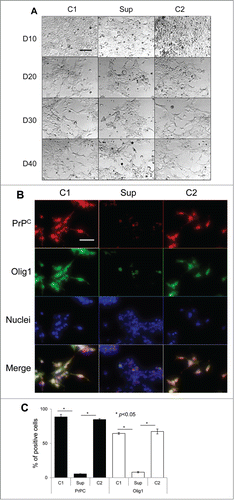
Figure 3. Suppression of PrPC delays differentiation into astrocytes. (A) Phase-contrast images of hESCs with suppressed PrPC-expression (Sup) and in two control lines (C1 and C2) taken at 10th, 20th, 30th, and 40th day of differentiation. (B) Immunostaining for PrPC (red) and GFAP (green) on 40th day of differentiation. Hoechst 33342 was used for staining of nuclei (blue). Scale bar = 50 μm. (C) Quantification and statistical analyses of PrPC- and GFAP-positive cells on 40th day of differentiation. The data represent a mean ± SD from three independent experiments for each cell line. Statistical significance was determined by Student's t test: *, P < 0.05.
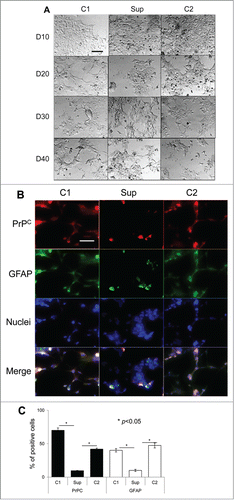
Western blotting for synapsin I (Syn, a neuronal marker), Olig1 or GFAP revealed that neuron-, oligodendrocyte- or astrocyte-specific differentiation protocols had a noticeable impact on the outcomes of differentiation, although the resulting cell populations were found to be heterogeneous in cultures produced according to three protocols (). For instance, cell differentiated according to the neuronal protocol expressed Syn, but also oligodendrocyte- and barely detectible astrocyte-specific markers (). Cells differentiated according to the oligodendrocytic protocol expressed Olig1 and to a lesser extent Syn and GFAP. Cell treated according to astrocyte-specific protocols expressed Olig1 and GFAP, but barely detectible amounts of Syn (). Nevertheless, regardless of the differentiation protocol, hESC lines with silenced PrPC displayed substantially lower levels of neuron-, oligodendrocyte- or astrocyte-specific markers in comparison to the corresponding control lines (). hESC with silenced PrPC as expected also showed the lowest level of PrPC expression relative to the control cell lines (). In summary, the current experiments revealed that suppression of PrPC expression blocked or substantially delayed differentiation of hESCc cells into three neuronal lineages (neuronal cells, oligodendrocytes and astrocytes); the effect of PrPC was observed regardless of the differentiation protocol employed.
Figure 4. western blotting analysis of expression of PrPC and three marker proteins. Western blotting (A) and quantification with statistical analyses (B) of the expression level of PrPC, Syn, Olig 1, and GFAP in hESCs with suppressed PrPC expression (Sup) and in two control lines (C1 and C2) cultured for 40 d under lineage-preferred differentiation conditions in the presence of tetracycline. β-actin was used as a loading control in (A). In (B) the expression level of each protein was normalized relative to that of β-actin in each differentiation protocol. The data represent a mean ± SD from three independent experiments. Statistical significance was determined by Student's t test: *, P < 0.05.
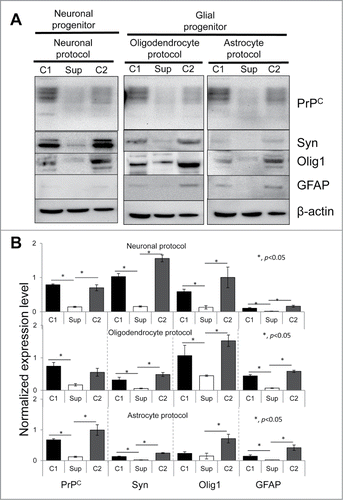
The effect of PrPC silencing at different time points on differentiation of hESCs
To test whether it is a specific time point of turning PrPC expression on and off or the total duration of PrPC silencing that has the most profound impact on delaying hESC differentiation, PrPC was silenced at different time points during spontaneous differentiation as shown in . The differentiation status was determined at the day 15 by analyzing the expression of protein markers Syn, Olig1 and GFAP, specific for neuronal, oligodendrocytic and astrocytic lineages, respectively (). Previously we showed that under spontaneous differentiation conditions hESCs cells gave rise to neuronal cells, oligodendrocytes and astrocytes.Citation21
Figure 5. The effect of PrPC silencing at different time points on differentiation of hESCs into neuron-, oligodendrocyte-, and astrocyte-specific lineages. (A) Schematic representation of eight experimental formats, where PrPC was temporarily silenced for 5, 10, or 15 d. hES+TetR+ShPrPC (Sup) cells were cultured under spontaneous differentiating conditions either in the absence (gray arrows, PrPC expression is on) or presence of 1 μg/ml tetracycline (white arrows, PrPC expression is silenced). Western blotting (B) and normalized expression level (C) of PrPC, Syn (synapsin I), Olig 1 and GFAP in hESCs after 15 d of culturing according to eight experimental formats described in panel A. β-actin was used as a loading control. In (C), the expression level of each protein was normalized relative to that of β-actin in each differentiation format. The data represent a mean ± SD from three independent experiments.
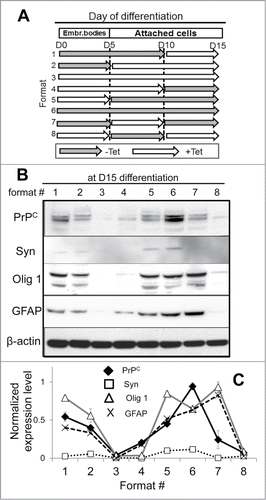
In the absence of PrPC silencing, all three markers and PrPC were detected by day 15, confirming on-going differentiation (format 6, ). Expression of Syn was relatively weak, as its expression typically increases at the later stages of neuronal differentiation. Comparison of the eight formats revealed that regardless of the specific time point at which PrPC was turned on or off, the extent to which differentiation was suppressed was roughly proportional to the length of time during which PrPC was silenced. Indeed, PrPC silencing for 5 d (formats 1, 5 and 7) showed relatively minor effects on expression of Olig1 and GFAP regardless of whether PrPC was silenced at the beginning, in the middle or at the end of the 15-d experiment (). PrPC silencing for 10 d (formats 2, 4, and 8) showed more substantial effects than silencing for 5 d. Notably, in the experiment where PrPC was continuously silenced during the last 10 d of experiment (format 2), the negative effect on differentiation was minimal in comparison to the formats 4 and 8 (). In fact, the negative effect of PrPC silencing for 10 d in format 2 was similar to those observed in formats # 1, 5 and 7, where PrPC was silenced for 5 d. This result illustrates that PrPC expression during the very initial stages might be more important than at the later stages. None of the three markers could be detected in the format where PrPC was silenced for the whole duration of the experiment (format 3, ), confirming again that PrPC is important for differentiation of cells in all three lineages.
Careful comparison within each group where PrPC was silencing for 5 or 10 d revealed relatively minor variations between the ratios of neuron-, oligodendrocyte- or astrocyte-specific markers (). This suggests that PrPC expression might have a somewhat limited impact on tuning the ratio between neuronal cells, oligodendrocytes and astrocytes at different time-points of spontaneous differentiation. However, temporal silencing of PrPC during different stages of differentiation was not able to alter drastically the cell fate of hESCs.
Discussion
In previous studies, we showed that ectopic expression of PrPC in hESCs under self-renewal conditions triggered differentiation toward lineages of three germ layers.Citation21 Silencing of PrPC in hESCs cultured under spontaneous differentiation conditions suppressed differentiation of cells toward ectodermal lineages, but did not affect differentiation of endodermal and mesodermal lineages, thereby changing the balance between the lineages of three germ layers.Citation21 Because all three protein markers used to examine ectodermal differentiation in previous studies were neuron-specific (growth-associated protein 43, synaptophysin and TH), it remained unclear whether PrPC was involved only in differentiation of neuronal cells or also in cells of other ectodermal lineages, such as oligodendrocyte- or astrocyte-committed lineages. The question of whether PrPC is expressed in oligodendrocytes, astrocytes or their immediate precursors remains controversial. The studies that examined different mouse brains areas including neurogenetic regions did not find PrPC expression in astrocytes or oligodendrocytes,Citation26,44 whereas several other studies that employed different animal models demonstrated expression of Prnp mRNA or PrPC in astrocytes or oligodendrocytes.Citation45-48
In the current study, we showed that silencing of PrPC in hESCs suppressed differentiation toward neuron-, oligodendrocyte-, and astrocyte-committed lineages (). Similar results were observed in three different experimental protocols, arguing that the effect of PrPC was independent of differentiation conditions employed. Notably, while each protocol was developed for differentiating hESCs into three different cell types, the resulting cell populations were found to be heterogeneous in each condition and consisted of oligodendrocytes, astrocytes and neuronal cells. Notably, PrPC silencing suppressed differentiation toward all three lineages under each differentiation condition.
Figure 6. A schematic model illustrating PrPC effects on self-renewal and differentiation of hESCs into neural lineages. Expression of PrPC in pluripotent hESCs triggers their differentiation into neuron-, oligodendrocyte- and astrocyte-committed lineages. PrPC guides differentiation at the very early stage, presumably the stage of uncommitted progenitor, via a yet unknown signaling pathway or through inhibiting cell-renewal.
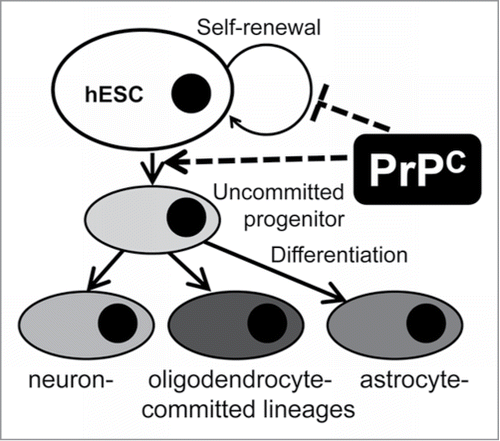
While the current work suggests that PrPC deficiency might lead to significant delay or even arrested of neural development in human, it is currently difficult to project these results on development of human embryo in part because such conclusions are not well supported by previous studies that employed animal models. Previous studies using PrPC knock out mice revealed only subtle phenotypes such as minor neurophysiological or behavioral abnormalities,Citation49-51 but no obvious problems in development.Citation7,8 Several studies pointed out that PrPC might be essential only under circumstances when animals are subjected to stress conditions, such as regeneration of adult muscle tissues after damageCitation38 or self-renewal of hematopoetic cells after irradiation.Citation27 It is difficult to comprehend the extent to which PrPC is essential for neurodevelopment in part because of some differences in biochemistry and physiology between humans and other mammals. A number of functional activities of PrPC and in particular those involved in neurotrophic activities,Citation19,22,23 cell adhesion,Citation9,Citation23–25 cell proliferation and differentiationCitation19,21,Citation26-31 are likely to depend on sialylation of PrPC N-linked glycans. Due to an irreversible mutation in the gene encoding human N-acetylneuraminic acid hydroxylase, different kinds of sialyc acid residues are synthesized in humans compared with the rest of mammalian species: N-acetyl neuraminic acid (Neu5Ac) is produced in human, while N-glycolylneuraminic acid (Neu5Gc) is in all other mammals.Citation52 Among other factors, the difference in structures of sialic acid residues might account for the differences in the spectrum of PrPC biological activities in humans and other mammals. Notably, early developmental arrests were described in PrP knock out models of zebrafishCitation9 supporting the role of PrPC in early development in at least one taxonomic class.
The recent article by Miranda et al. provides comprehensive review of the studies on the role of PrPC in stem cell pluripotency, self-renewal and differentiation,Citation53 therefore we will only discuss the results that are the most relevant to the current finding. During early embryogenesis, PrPC expression was found to be largely limited to cells that undergo neural differentiation.Citation54,55 The fact that PrPC expression accompanies neuronal differentiation during embryo development was supported by work on in vitro models that employed neural precursors and ESCs.Citation26,28,33 Treatment of primary neuronal culture or embryonic neurons with recombinant PrP in α-helical conformation helped neuronal survival and stimulated neurite outgrowth.Citation36,56 The current finding that differentiation toward all three neural lineages was delayed, suggests that PrPC is involved at the early stages of differentiation, perhaps, at the stage of uncommitted neural progenitor cells rather than lineage-committed neural progenitors (). In support of this hypothesis, the experiment on silencing PrPC expression at different stages of early differentiation revealed that PrPC expression during the very early stage of embryonic bodies had a more significant impact than silencing at the later stages (). These results expands the previous view and suggests that not only does PrPC expression accompany cell fate restriction of multipotent stem cells toward neural lineages,Citation31 but is actively involved at the early stages of differentiation toward neural-committed lineages. While no deleterious effects were reported in PrPC knock out mice, a double knock out of PrPC and its paralog Shadoo was found to have lethal effect on mouse development at the E10.5 stage.Citation57 The current work is consistent with the results of the recent studies that illustrate a delay in differentiation toward oligodendrocytes in embryonic primary cell cultures isolated at P0-P2 stage from Prnp0/0 mouse pups relative to cultures from Prnp+/+ mouse pups.Citation58 Other studies reported faster astrocyte maturation in cultured embryonic astrocytes from PrPC -overexpressing mice compared with wild-type mice,Citation59 and a slower rate of astrocyte development in cultures from PrPC-null animals then wild-type mice.Citation60 These results are in accord with the current work that PrPC silencing delays differentiation of the astrocyte-committed lineage. We did not observe significant effect of PrPC silencing in changing the balance between neuron-, oligodendrocyte- or astrocyte-specific differentiation pathways. This is consistent with the idea that PrPC is involved in differentiation of cell of all three neural lineages at very early stages ().
How does PrPC regulate ESCs self-renewal and differentiation? Several plausible PrPC interacting partners and pathways have recently been described. Knocking out the Prnp gene or blocking PrPC with PrPC-specific antibodies was shown to downregulate the mRNA expression level of Nanog, a transcription factor which is involved in ESCs self-renewal and controlling pluripotency.Citation61 Knocking down PrPC expression by siRNA in mouse ESCs had a negative effect on transcription of the ectodermal marker Nestin.Citation62 This result is consistent with the current study that PrPC is involved at the stage of stem cell differentiation into neural progenitor cells. Other studies reported that the positive effect of PrPC on neuronal differentiation and neurite outgrowth could be mediated via interaction of PrPC with the stress-inducible protein STI1,Citation18,30 laminin,Citation63 or the neural cell adhesion molecule NCAM.Citation34 In addition, PrPC could be involved in changing the balance between self-renewal and differentiation via interaction with cdk2, which is known to participate in the cell cycle G1 to S phase transition.Citation64 Our previous studies showed that at the early stages of hESCs differentiation, PrPC silencing changed the structure of cell cycle by inhibiting the cell cycle G1 to S phase transitionCitation21 ().
Importance of PrPC for early neural differentiation might also play a critical role in prion pathogenesis. As supported by recent study, PrPC depletion due to PrPSc spread and replication in CNS is likely to have a negative impact on the ability of CNS to replace lost neurons via stimulating differentiation of adult stem cells during prion disease.Citation65 Consistent with this hypothesis, PrPC expression level in the mouse subventricular zone was found to correlate with differentiation of multipotent neural precursors during adult neurogenesis.Citation26
Materials and Methods
hESCs culturing
The protocol for using hESCs (H9, National Stem Cell Bank, Madison, WI, USA) was reviewed and approved by the University of Maryland, Baltimore Embryonic Stem Cell Research Oversight Committee and Institutional Review. The following H9 hESCs were employed in this study: i) hES+TetR is a hESC line transfected with a lentiviral vector expressing tetracycline repressor (referred to as C1); ii) hES+TetR+ShPrPC is a hESC line transfected with lentiviral vectors expressing tetracycline repressor and inducible short hairpin RNA for suppression of PrPC expression (referred to as Sup); iii) hES+TetR+ShScram is a hESC line transfected with lentiviral vectors expressing tetracycline repressor and inducible scrambled short hairpin RNA as a control for non-specific effects (referred to as C2). These hESCs lines were described in detail in a previous study.Citation21 H9 hESCs were maintained on mitomycin C (Sigma, St. Louis, MO, USA) -treated mouse embryonic fibroblasts (MEFs) (American Type Culture Collection, Manassas, VA, USA) feeder layers in DMEM/F12 (Invitrogen, Carlsbad, CA, USA) supplemented with 20% knockout serum replacement (Invitrogen), 0.1 mM β-mercaptoethanol (Sigma), 0.1 mM non-essential amino acids (Invitrogen), 50 U/ml penicillin G (Invitrogen), 50 μg/ml streptomycin (Invitrogen) and 4 ng/ml human recombinant basic fibroblast growth factor (bFGF, Invitrogen) at 37°C in 5% CO2. hESC colonies were subcultured on new feeder cells every 5–7 d.
Induced differentiation of hESCs
For differentiation into neuronal cells, the protocol described by Nat and coauthors was used as previously described.Citation43 Briefly, after culturing in feeder free conditions, hESC colonies were incubated in neural induction medium (NIM, DMEM:Neurobasal (1:1) supplemented with 1x N2 and 1x B27 supplements and 2mM Glutamax) for one day. Then, hESCs were cultured on non-adherent culture dishes in NIM until they form uniformed aggregates for 2–4 d. Floating aggregates were transferred to neural proliferation medium (NPM, DMEM:Neurobasal (1:1) supplemented with 0.5x N2 and 0.5x B27 supplements, 2mM Glutamax and 20 ng/ml FGF2) on day 5. At day 7, aggregates were plated on laminin-coated culture dishes in NPM and cultured for an additional 10 d. The medium was changed with neural differentiation medium (NDM, Neurobasal supplemented 1x B27 supplement, 2mM Glutamax, 10 ng/ml brain derived neurotrophic factor, BDNF) for additional 20 d.
For differentiation into oligodendrocytes, the protocol described by Sharp and coauthors was used as previously described.Citation42 Briefly, mouse embryonic feeder-conditioned medium (MEF-CM), glial restrictive medium (GRM, DMEM with 1x B27 supplement, 1x Insulin/Transferrin/Selenium Solution (Gibco) and Transition medium (MEF-CM:GRM = 1:1) were used. To induce differentiation into oligodendrocyte progenitor cells, hESCs were treated with collagenase IV (Sigma), and cell aggregates were distributed to low-attachment cell culture plates. After cultivation for two days with transition medium, cell aggregates were cultured with GRM+20 ng/ml epidermal growth factor (EFG, Sigma) supplemented with 10 μg/ml retinoic acid (RA, Sigma) for 10 d, then cultured with GRM supplemented with 20 ng/ml EGF medium for additional 15 d. Cell aggregates were transferred to Matrigel (BD Biosciences)-coated plates and cultured with GRM medium supplemented with 20 ng/ml EGF for additional 20 d while changing medium every other day.
For differentiation into astrocytes, the protocol described by Emdad and coauthors was used.Citation41 Briefly, cells were mechanically detached from culture plates in undifferentiated condition, and cell aggregates were cultured in low-attachment plates to generate EBs. At day six, EBs were cultured in Matrigel-coated plates using neurobasal medium with 2x N2 supplement, 10 ng/ml FGF2, 20 ng/ml EGF, 500 ng/ml Noggin for 3 d, then cultured in neurobasal medium with 2x N2 supplement, 10 ng/ml FGF2, 20 ng/ml EGF, 500 ng/ml Noggin, 20 ng/ml ciliary neurotrophic factor (CNTF, R&D Systems) for additional 3 d. Cell aggregates were then transferred and cultured with neurobasal medium supplemented with 2x N2, 10 ng/ml FGF2, 500 ng/ml Noggin, 20 ng/ml CNTF for 3 d, then in neurobasal medium supplement with 2x N2, 20 ng/ml CNTF for additional 3 d. Neural tube-like rosettes were detached mechanically at the day 15 of differentiation and transferred to Matrigel-coated plates in the same medium.
For spontaneous differentiation, mechanically dissociated and harvested hESCs cells were grown in suspension culture without MEFs using the same medium as described in hESCs culturing protocol, but without bFGF for 5 d in absence or presence of 1 μg/ml tetracycline. At the day 5 of differentiation, formed EBs were attached onto 0.1% gelatin-coated culture plates, then cultured for additional 5 d in the absence or presence of 1 μg/ml tetracycline, as indicated. At the day 10 of differentiation, the culture medium was changed, and cells were cultured in the absence or presence of 1 μg/ml tetracycline for additional 5 d as indicated. At the day 15 of differentiation, cells were harvested and cell lysates were used for western blotting analysis.
Immunostaining
Cells were washed with phosphate buffered saline (PBS), fixed with 4% paraformaldehyde (Sigma) for 15 min at room temperature (RT), washed again three times with PBS, then incubated with PBS-T (PBS + 0.25% triton X-100) for 10 min at RT followed by incubation with 1% bovine serum albumin (BSA) in PBS-T for 30 min at RT. After blocking with BSA, cells were stained in 1% BSA in PBS-T for one hour at RT with the following antibodies: mouse anti-TH (Millipore), rabbit anti-Olig1 (Sigma), rabbit anti-GFAP (Novus), mouse anti-PrP 3F4 (Signet), or humanized anti-PrP P (Signet). Double immunostaining was visualized using combination of goat anti-mouse secondary antibodies conjugated with Alexa 488 or Alexa 546 and goat anti-rabbit secondary antibodies conjugated with Alexa 488 or Alexa 546 (Invitrogen). For antibody P, donkey anti-human secondary antibody conjugated with Alexa 546 (Invitrogen) was used. Hoechst 33342 was used for staining the nucleus. After staining, cells were mounted with antifade mounting fluorescence medium (Dako). Microscopy was performed on an inverted microscope (Nikon Eclipse TE2000-U) with an illumination system X-cite 120 (EXFO Photonics Solutions). Digital images were acquired using a cooled 12-bit CoolSnap HQ CCD camera (Photometrics). Images were processed with WCIF ImageJ software (National Institute of Health, Bethesda, MD). The experiments were performed in triplicate; at least three fields of view were analyzed for each repeat for statistical analysis.
Western blots and data analysis
Cells were lysed in ProteoJET Mammalian Cell Lysis Reagent (Fermantas) with a protease inhibitor cocktail (Roche), and insoluble materials were precipitated by centrifugation at 16,000 X g for 15 min at 4°C. The supernatant was transferred to a new tube and protein concentrations were determined by absorbance spectroscopy. Twelve micrograms of each lysate was resolved by 12% sodium dodecyl sulfate-PAGE (SDS-PAGE), transferred to a polyvinylidene fluoride (PVDF) membrane (Millipore), and then immunoblotted with rabbit anti-Syn (Cell Signaling), rabbit anti-Olig1 (Sigma), rabbit anti-GFAP (Novus) or mouse anti-β-actin (Sigma) antibody. Binding of primary antibody was detected by incubating blots with horseradish-peroxidase-conjugated goat anti-mouse or anti-rabbit antibody, and blots were developed using chemiluminescence (Thermo Scientific).
To analyze expression level of specific proteins, densitometry analysis for each protein band and corresponding background was performed using WCIF ImageJ software, then background was subtracted. Then, the resulting net value representing the expression level of an individual protein was normalized to net value representing the expression level of a loading control (β-actin) in a corresponding sample. Three independent experiments were performed and the data represent a mean ± SD from three experiments. Statistical significance was determined by Student's t test: *, P < 0.05.
Acknowledgment
We thank Pamela Wright for editing the manuscript.
Funding
This work was supported by a Postdoctoral Fellowship Grant of Maryland Stem Cell Commission to YJL and NIH grant NS045585 to IVB.
References
- Prusiner SB. Prion diseases and the BSE crisis. Science 1997; 278:245-51; PMID:9323196; http://dx.doi.org/10.1126/science.278.5336.245
- Stahl N, Borchelt DR, Hsiao K, Prusiner SB. Scrapie prion protein contains a phosphatidylinositol glycolipid. Cell 1987; 51:229-40; PMID:2444340; http://dx.doi.org/10.1016/0092-8674(87)90150-4
- Stahl N, Baldwin MA, Teplow DB, Hood L, Gibson BW, Burlingame AL, Prusiner SB. Structural studies of the scrapie prion protein using mass spectrometry and amino acid sequencing. Biochemistry 1993; 32:1991-2002; PMID:8448158; http://dx.doi.org/10.1021/bi00059a016
- Manson J, West JD, Thomson V, McBride P, Kaufman MH, Hope J. The prion protein gene: a role in mouse embryogenesis? Development 1992; 115:117-22; PMID:1353438
- Fujisawa M, Kanai Y, Nam SY, Maeda S, Nakamuta N, Kano K, Kurohmaru M, Hayashi Y. Expression of Prnp mRNA (prion protein gene) in mouse spermatogenic cells. J Reprod Dev 2004; 50:565-70; PMID:15514463; http://dx.doi.org/10.1262/jrd.50.565
- Johnston DS, Wright WW, Dicandeloro P, Wilson E, Kopf GS, Jelinsky SA. Stage-specific gene expression is a fundamental characteristic of rat spermatogenic cells and Sertoli cells. Proc Natl Acad Sci U S A 2008; 105:8315-20; PMID:18544648; http://dx.doi.org/10.1073/pnas.0709854105
- Büeler H, Fischer M, Lang Y, Bluethmann H, Lipp HP, DeArmond SJ, Prusiner SB, Aguet M, Weissmann C. Normal development and behaviour of mice lacking the neuronal cell-surface PrP protein. Nature 1992; 356:577-82; PMID:1373228; http://dx.doi.org/10.1038/356577a0
- Manson JC, Clarke AR, Hooper ML, Aitchison L, McConnell I, Hope J. 129/Ola mice carrying a null mutation in PrP that abolishes mRNA production are developmentally normal. Mol Neurobiol 1994; 8:121-7; PMID:7999308; http://dx.doi.org/10.1007/BF02780662
- Málaga-Trillo E, Solis GP, Schrock Y, Geiss C, Luncz L, Thomanetz V, Stuermer CAO. Regulation of embryonic cell adhesion by the prion protein. PLoS Biol 2009; 7:e55; PMID:19278297; http://dx.doi.org/10.1371/journal.pbio.1000055
- Pauly PC, Harris DA. Copper stimulates endocytosis of the prion protein. J Biol Chem 1998; 273:33107-10; PMID:9837873; http://dx.doi.org/10.1074/jbc.273.50.33107
- Brown DR, Wong BS, Hafiz F, Clive C, Haswell SJ, Jones IM. Normal prion protein has an activity like that of superoxide dismutase. Biochem J 1999; 344:1-5; PMID:10548526; http://dx.doi.org/10.1042/0264-6021:3440001
- Vassallo N, Herms J. Cellular prion protein function in copper homeostasis and redox signalling at the synapse. J Neurochem 2003; 86:538-44; PMID:12859667; http://dx.doi.org/10.1046/j.1471-4159.2003.01882.x
- Millhauser GL. Copper binding in the prion protein. Acc Chem Res 2004; 37:79-85; PMID:14967054; http://dx.doi.org/10.1021/ar0301678
- Mouillet-Richard S, Ermonval M, Chebassier C, Laplanche JL, Lehmann S, Launay JM, Kellermann O. Signal transduction through prion protein. Science 2000; 289:1925-8; PMID:10988071; http://dx.doi.org/10.1126/science.289.5486.1925
- Roucou X, Giannopoulos PN, Zhang Y, Jodoin J, Goodyer CG, LeBlanc A. Cellular prion protein inhibits proapoptotic Bax conformational change in human neurons and in breast carcinoma MCF-7 cells. Cell Death Differ 2005; 12:783-95; PMID:15846375; http://dx.doi.org/10.1038/sj.cdd.4401629
- Bounhar Y, Zhang Y, Goodyer CG, LeBlanc A. Prion protein protects human neurons against Bax-mediated apoptosis. J Biol Chem 2001; 276:39145-9; PMID:11522774; http://dx.doi.org/10.1074/jbc.C100443200
- Chiarini LB, Freitas ARO, Zanata SM, Brentani RR, Martins VR, Linden R. Cellular prion protein transduces neuroprotective signals. EMBO J 2002; 21:3317-26; PMID:12093733; http://dx.doi.org/10.1093/emboj/cdf324
- Lopes MH, Hajj GNM, Muras AG, Mancini GL, Castro RMPS, Ribeiro KCB, Brentani RR, Linden R, Martins VR. Interaction of cellular prion and stress-inducible protein 1 promotes neuritogenesis and neuroprotection by distinct signaling pathways. J Neurosci 2005; 25:11330-9; PMID:16339028; http://dx.doi.org/10.1523/JNEUROSCI.2313-05.2005
- Lima FR, Arantes CP, Muras AG, Nomizo R, Brentani RR, Martins VR. Cellular prion protein expression in astrocytes modulates neuronal survival and differentiation. J Neurochem 2007; 103:2164-76; PMID:17868300; http://dx.doi.org/10.1111/j.1471-4159.2007.04904.x
- Liang J, Pan Y, Zhang D, Guo C, Shi Y, Wang J, Chen Y, Wang X, Liu J, Guo X, et al. Cellular prion protein promotes proliferation and G1/S transition of human gastric cancer cells SGC7901 and AGS. FASEB J 2007; 21:2247-56; PMID:17409275; http://dx.doi.org/10.1096/fj.06-7799com
- Lee YJ, Baskakov IV. The cellular form of the prion protein is involved in controlling cell cycle dynamics, self-renewal, and the fate of human embryonic stem cell differentiation. J Neurochem 2013; 124:310-22; PMID:22860629; http://dx.doi.org/10.1111/j.1471-4159.2012.07913.x
- Chen S, Mangé A, Dong L, Lehmann S, Schachner M. Prion protein as trans-interacting partner for neurons is involved in neurite outgrowth and neuronal survival. Mol Cell Neurosci 2003; 22:227-33; PMID:12676532; http://dx.doi.org/10.1016/S1044-7431(02)00014-3
- Santuccione A, Sytnyk V, Leshchyns’ka I, Schachner M. Prion protein recruits its neuronal receptor NCAM to lipid rafts to activate p59fyn and to enhance neurite outgrowth. J Cell Biol 2005; 169:341-54; PMID:15851519; http://dx.doi.org/10.1083/jcb.200409127
- Schmitt-Ulms G, Legname G, Baldwin MA, Ball HL, Bradon N, Bosque PJ, Crossin KL, Edelman GM, DeArmond SJ, Cohen FE, et al. Binding of neural cell adhesion molecules (N-CAMs) to the cellular prion protein. J Mol Biol 2001; 314:1209-25; PMID:11743735; http://dx.doi.org/10.1006/jmbi.2000.5183
- Viegas P, Chaverot N, Enslen H, Perrière N, Couraud PO, Cazaubon S. Junctional expression of the prion protein PrPC by brain endothelial cells: a role in trans-endothelial migration of human monocytes. J Cell Sci 2006; 119:4634-43; PMID:17062642; http://dx.doi.org/10.1242/jcs.03222
- Steele AD, Emsley JG, Ozdinler PH, Lindquist S, Macklis JD. Prion protein (PrPc) positively regulates neural precursor proliferation during developmental and adult mammalian neurogenesis. Proc Natl Acad Sci U S A 2006; 103:3416-21; PMID:16492732; http://dx.doi.org/10.1073/pnas.0511290103
- Zhang CC, Steele AD, Lindquist S, Lodish HF. Prion protein is expressed on long-term repopulating hematopoietic stem cells and is important for their self-renewal. Proc Natl Acad Sci U S A 2006; 103:2184-9; PMID:16467153; http://dx.doi.org/10.1073/pnas.0510577103
- Lee YJ, Baskakov IV. Treatment with normal prion protein delays differentiation and helps to maintain high proliferation activity in human embryonic stem cells. J Neurochem 2010; 114:362-73; PMID:20089130; http://dx.doi.org/10.1111/j.1471-4159.2010.06601.x
- Panigaj M, Glier H, Wildova M, Holada K. Expression of prion protein in mouse erythroid progenitors and differentiating murine erythroleukemia cells. PLoS One 2011; 6:e24599; PMID:21912705; http://dx.doi.org/10.1371/journal.pone.0024599
- Santos TG, Silva IR, Costa-Silva B, Lepique AP, Martins VR, Lopes MH. Enhanced neural progenitor/stem cells self-renewal via the interaction of stress-inducible protein 1 with the prion protein. Stem Cells 2011; 29:1126-36; PMID:21608082; http://dx.doi.org/10.1002/stem.664
- Mouillet-Richard S, Laurendeau I, Vidaud M, Kellermann O, Laplanche JL. Prion protein and neuronal differentiation: quantitative analysis of prnp gene expression in a murine inducible neuroectodermal progenitor. Microbes Infect 1999; 1:969-76; PMID:10617928; http://dx.doi.org/10.1016/S1286-4579(99)80514-0
- Monnet C, Marthiens V, Enslen H, Frobert Y, Sobel A, Mège RM. Heterogeneity and regulation of cellular prion protein glycoforms in neuronal cell lines. Eur J Neurosci 2003; 18:542-8; PMID:12911750; http://dx.doi.org/10.1046/j.1460-9568.2003.02777.x
- Novitskaya V, Makarava N, Sylvester I, Bronstein IB, Baskakov IV. Amyloid fibrils of mammalian prion protein induce axonal degeneration in NTERA2-derived terminally differentiated neurons. J Neurochem 2007; 102:398-407; PMID:17472702; http://dx.doi.org/10.1111/j.1471-4159.2007.04537.x
- Prodromidou K, Papastefanaki F, Sklaviadis T, Matsas R. Functional cross-talk between the cellular prion protein and the neural cell adhesion molecule is critical for neuronal differentiation of neural stem/precursor cells. [Epub ahead of print]. Stem Cells 2014; 32:1674-87; PMID:24497115; http://dx.doi.org/10.1002/stem.1663
- Salès N, Hässig R, Rodolfo K, Di Giamberardino L, Traiffort E, Ruat M, Frétier P, Moya KL. Developmental expression of the cellular prion protein in elongating axons. Eur J Neurosci 2002; 15:1163-77; PMID:11982627; http://dx.doi.org/10.1046/j.1460-9568.2002.01953.x
- Kanaani J, Prusiner SB, Diacovo J, Baekkeskov S, Legname G. Recombinant prion protein induces rapid polarization and development of synapses in embryonic rat hippocampal neurons in vitro. J Neurochem 2005; 95:1373-86; PMID:16313516; http://dx.doi.org/10.1111/j.1471-4159.2005.03469.x
- Bremer J, Baumann F, Tiberi C, Wessig C, Fischer H, Schwarz P, Steele AD, Toyka KV, Nave KA, Weis J, et al. Axonal prion protein is required for peripheral myelin maintenance. Nat Neurosci 2010; 13:310-8; PMID:20098419; http://dx.doi.org/10.1038/nn.2483
- Stella R, Massimino ML, Sandri M, Sorgato MC, Bertoli A. Cellular prion protein promotes regeneration of adult muscle tissue. Mol Cell Biol 2010; 30:4864-76; PMID:20679477; http://dx.doi.org/10.1128/MCB.01040-09
- Thomson JA, Itskovitz-Eldor J, Shapiro SS, Waknitz MA, Swiergiel JJ, Marshall VS, Jones JM. Embryonic stem cell lines derived from human blastocysts. Science 1998; 282:1145-7; PMID:9804556; http://dx.doi.org/10.1126/science.282.5391.1145
- Nishikawa S, Jakt LM, Era T. Embryonic stem-cell culture as a tool for developmental cell biology. Nat Rev Mol Cell Biol 2007; 8:502-7; PMID:17522593; http://dx.doi.org/10.1038/nrm2189
- Emdad L, D’Souza SL, Kothari HP, Qadeer ZA, Germano IM. Efficient differentiation of human embryonic and induced pluripotent stem cells into functional astrocytes. Stem Cells Dev 2012; 21:404-10; PMID:21631388; http://dx.doi.org/10.1089/scd.2010.0560
- Sharp J, Hatch M, Nistor G, Keirstead H. Derivation of oligodendrocyte progenitor cells from human embryonic stem cells. Methods Mol Biol 2011; 767:399-409; PMID:21822891; http://dx.doi.org/10.1007/978-1-61779-201-4_29
- Nat R, Nilbratt M, Narkilahti S, Winblad B, Hovatta O, Nordberg A. Neurogenic neuroepithelial and radial glial cells generated from six human embryonic stem cell lines in serum-free suspension and adherent cultures. Glia 2007; 55:385-99; PMID:17152062; http://dx.doi.org/10.1002/glia.20463
- Ford MJ, Burton LJ, Li H, Graham CH, Frobert Y, Grassi J, Hall SM, Morris RJ. A marked disparity between the expression of prion protein and its message by neurones of the CNS. Neuroscience 2002; 111:533-51; PMID:12031342; http://dx.doi.org/10.1016/S0306-4522(01)00603-0
- Moser M, Colello RJ, Pott U, Oesch B. Developmental expression of the prion protein gene in glial cells. Neuron 1995; 14:509-17; PMID:7695897; http://dx.doi.org/10.1016/0896-6273(95)90307-0
- Kercher L, Favara C, Striebel JF, LaCasse R, Chesebro B. Prion protein expression differences in microglia and astroglia influence scrapie-induced neurodegeneration in the retina and brain of transgenic mice. J Virol 2007; 81:10340-51; PMID:17652390; http://dx.doi.org/10.1128/JVI.00865-07
- Jackson WS, Krost C, Borkowski AW, Kaczmarczyk L. Translation of the prion protein mRNA is robust in astrocytes but does not amplify during reactive astrocytosis in the mouse brain. PLoS One 2014; 9:e95958; PMID:24752288; http://dx.doi.org/10.1371/journal.pone.0095958
- Lainé J, Marc ME, Sy MS, Axelrad H. Cellular and subcellular morphological localization of normal prion protein in rodent cerebellum. Eur J Neurosci 2001; 14:47-56; PMID:11488948; http://dx.doi.org/10.1046/j.0953-816x.2001.01621.x
- Criado JR, Sánchez-Alavez M, Conti B, Giacchino JL, Wills DN, Henriksen SJ, Race R, Manson JC, Chesebro B, Oldstone MB. Mice devoid of prion protein have cognitive deficits that are rescued by reconstitution of PrP in neurons. Neurobiol Dis 2005; 19:255-65; PMID:15837581; http://dx.doi.org/10.1016/j.nbd.2005.01.001
- Nazor KE, Seward T, Telling GC. Motor behavioral and neuropathological deficits in mice deficient for normal prion protein expression. Biochim Biophys Acta 2007; 1772:645-53; PMID:17531449; http://dx.doi.org/10.1016/j.bbadis.2007.04.004
- Tobler I, Gaus SE, Deboer T, Achermann P, Fischer M, Rülicke T, Moser M, Oesch B, McBride PA, Manson JC. Altered circadian activity rhythms and sleep in mice devoid of prion protein. Nature 1996; 380:639-42; PMID:8602267; http://dx.doi.org/10.1038/380639a0
- Varki A. Sialic Acids. In: Varki A, Cummings R, Esko J, Freeze H, Hart G, Marth J, eds. Essentials of Glycobiology. Cold Spring Harbor, NY: Cold Spring Harbor Laboratory Press, 1999:195-210.
- Miranda A, Ramos-Ibeas P, Pericuesta E, Ramirez MA, Gutierrez-Adan A. The role of prion protein in stem cell regulation. Reproduction 2013; 146:R91-9; PMID:23740082; http://dx.doi.org/10.1530/REP-13-0100
- Tremblay P, Bouzamondo-Bernstein E, Heinrich C, Prusiner SB, DeArmond SJ. Developmental expression of PrP in the post-implantation embryo. Brain Res 2007; 1139:60-7; PMID:17292334; http://dx.doi.org/10.1016/j.brainres.2006.12.055
- Peralta OA, Huckle WR, Eyestone WH. Developmental expression of the cellular prion protein (PrP(C) ) in bovine embryos. Mol Reprod Dev 2012; 79:488-98; PMID:22674901; http://dx.doi.org/10.1002/mrd.22057
- Novitskaya V, Bocharova OV, Bronstein I, Baskakov IV. Amyloid fibrils of mammalian prion protein are highly toxic to cultured cells and primary neurons. J Biol Chem 2006; 281:13828-36; PMID:16554307; http://dx.doi.org/10.1074/jbc.M511174200
- Young R, Passet B, Vilotte M, Cribiu EP, Béringue V, Le Provost F, Laude H, Vilotte JL. The prion or the related Shadoo protein is required for early mouse embryogenesis. FEBS Lett 2009; 583:3296-300; PMID:19766638; http://dx.doi.org/10.1016/j.febslet.2009.09.027
- Bribián A, Fontana X, Llorens F, Gavín R, Reina M, García-Verdugo JM, Torres JM, de Castro F, del Río JA. Role of the cellular prion protein in oligodendrocyte precursor cell proliferation and differentiation in the developing and adult mouse CNS. PLoS One 2012; 7:e33872; PMID:22529900; http://dx.doi.org/10.1371/journal.pone.0033872
- Hartmann CA, Martins VR, Lima FR. High levels of cellular prion protein improve astrocyte development. FEBS Lett 2013; 587:238-44; PMID:23238082; http://dx.doi.org/10.1016/j.febslet.2012.11.032
- Arantes C, Nomizo R, Lopes MH, Hajj GN, Lima FR, Martins VR. Prion protein and its ligand stress inducible protein 1 regulate astrocyte development. Glia 2009; 57:1439-49; PMID:19243016; http://dx.doi.org/10.1002/glia.20861
- Miranda A, Pericuesta E, Ramírez MA, Gutierrez-Adan A. Prion protein expression regulates embryonic stem cell pluripotency and differentiation. PLoS One 2011; 6:e18422; PMID:21483752; http://dx.doi.org/10.1371/journal.pone.0018422
- Peralta OA, Huckle WR, Eyestone WH. Expression and knockdown of cellular prion protein (PrPC) in differentiating mouse embryonic stem cells. Differentiation 2011; 81:68-77; PMID:20926176; http://dx.doi.org/10.1016/j.diff.2010.09.181
- Santos TG, Beraldo FH, Hajj GN, Lopes MH, Roffe M, Lupinacci FC, Ostapchenko VG, Prado VF, Prado MA, Martins VR. Laminin-γ1 chain and stress inducible protein 1 synergistically mediate PrPC-dependent axonal growth via Ca2+ mobilization in dorsal root ganglia neurons. J Neurochem 2013; 124:210-23; PMID:23145988; http://dx.doi.org/10.1111/jnc.12091
- Belachew S, Aguirre AA, Wang H, Vautier F, Yuan X, Anderson S, Kirby M, Gallo V. Cyclin-dependent kinase-2 controls oligodendrocyte progenitor cell cycle progression and is downregulated in adult oligodendrocyte progenitors. J Neurosci 2002; 22:8553-62; PMID:12351729
- Relaño-Ginès A, Gabelle A, Hamela C, Belondrade M, Casanova D, Mourton-Gilles C, Lehmann S, Crozet C. Prion replication occurs in endogenous adult neural stem cells and alters their neuronal fate: involvement of endogenous neural stem cells in prion diseases. PLoS Pathog 2013; 9:e1003485; PMID:23935493; http://dx.doi.org/10.1371/journal.ppat.1003485