Abstract
The crucial step for the fatal neurodegenerative prion diseases involves the conversion of a normal cellular protein, PrPC, into a fibrous pathogenic form, PrPSc, which has an unusual stability against heat and resistance against proteinase K digestion. A successful challenge to reverse the reaction from PrPSc into PrPC is considered valuable, as it would give a key to dissolving the complex molecular events into thermodynamic and kinetic analyses and may also provide a means to prevent the formation of PrPSc from PrPC eventually in vivo. Here we show that, by applying pressures at kbar range, the “proteinase K-resistant” fibrils (rHaPrPres) prepared from hamster prion protein (rHaPrP [23–231]) by seeding with brain homogenate of scrapie-infected hamster, becomes easily digestible. The result is consistent with the notion that rHaPrPres fibrils are dissociated into rHaPrP monomers under pressure and that the formation of PrPSc from PrPC is thermodynamically controlled. Moreover, the efficient degradation of prion fibrils under pressure provides a novel means of eliminating infectious PrPSc from various systems of pathogenic concern.
Abbreviations
PK | = | proteinase K |
PrPC | = | cellular form of prion protein |
PrPSc | = | scrapie form of prion protein |
rHaPrP | = | recombinant Hamster prion protein |
rHaPrPres | = | PK-resistant recombinant Hamster prion protein |
AFM | = | atomic force microscopy |
QUIC | = | quaking-induced conversion |
Introduction
The crucial step for the fatal neurodegenerative prion diseases involves the transformation of a normal cellular protein, PrPC, into a fibrous pathogenic form of the protein, PrPSc, either sporadically or by infection.Citation1 The transformation of PrPC into PrPSc is a slow process in vivo and, once formed, the fibril shows an exceptionally high stability against heat treatmentCitation2 and high resistivity against enzymatic proteolysis.Citation3 Thus the transformation is considered practically irreversible, prohibiting the study how PrPSc is thermodynamically and kinetically related to its normal precursor PrPC, one of the fundamental questions in pursuing the cause of prion disease, and use the relationship for efficient removal of PrPSc.
Pressure has proven to be a generally useful perturbation for investigating protein conformational equilibrium, particularly as it covers a wide dynamic range of conformation, namely from folded to unfolded of a globular proteinCitation4 as well as from monomers to oligomers in protein assembly.Citation5 In general, if a protein exists in solution in an equilibrium mixture of conformational states mutually differing in partial molar volume ΔV,Citation4 pressure shifts their equilibrium in favor of the lower volume states and increasing the relative population of the latter often dramatically by a factor ∼exp (–PΔV/RT).Citation5,6 Although no information is available on the volumetric property of Hamster rPrP in its monomeric and fibrous states, the study on Murine PrP showed that aggregated β-sheet-rich MrPrP [23–231] undergoes unfolding with ΔV = –43.6 ml mol−1.7 Furthermore, in view of the fact that rPrP oligomers of human prion protein is dissociable under pressure,Citation8 meaning ΔV = Vmonomer –Voligo < 0 and that hen lysozyme and its mutant are distinctly more voluminous in the amyloid protofibril state than in its naturally denatured monomeric state, ΔV = Vmonomer –Vfibril < 0,Citation9-13 we expect that under closely physiological conditions the volume is lower for the rPrP monomer, namely, ΔV = Vmonomer –Vfibril < 0. Furthermore, we found that the shrinking of the protofibril undergoes by the dissociation of a monomeric species at the growing end of the fibril,Citation12 i.e., by obeying the linear polymerization mechanism in which the fibril and the monomer are in dynamic equilibrium.Citation13 Thus we have ample reasons to expect that the application of pressure to a solution containing PrP fibrils would shift the equilibrium toward dissociation, increasing the equilibrium concentration of PrP monomers, PrPC, which, in the presence of proteinase K (PK), would be readily degraded into smaller fragments. The pressure-enhanced degradation of fibrils may be evaluated at 0.1 MPa after certain period of the reaction, by monitoring the diminishing number of fibrils with an atomic force microscopy (AFM) and their degradation into peptide fragments by western blotting.
Results and Discussion
illustrates the actual experimental procedure employed in the present work. Instead of directly working on PrPSc in vivo, we choose, at this stage, to work with proteinase K-resistant fibrils (rHaPrPres), which is prepared from recombinant hamster PrP (rHaPrP [23–231]) in vitro by seeding with brain homogenate of scrapie-infected hamster under quaking, or by QUIC (quaking-induced conversion),Citation14 the process mimicking the conversion of cellular PrPC into PrPSc. The automated shaking was performed on the solution containing rHaPrP for 24 h at 37 °C in 0.5 M Gdn-HCl, 150 mM NaCl, 150 mM HEPES buffer, pH7.0, in the presence of diluted brain homogenate of the hamster that had been infected with PrPSc from sheep. Because of the high effective molecular weight of the fibrils and the small quantity of the protein available for analysis (<1μg) at one time, we employed AFM and western blotting, both of which are capable of detecting a small quantity of proteins (<1μg) without using high pressure NMR spectroscopy.Citation8,15 The former gives a direct image, though qualitative, on what happens to rHaPrPres fibrils, while the latter should give the degradation process more quantitatively.
Figure 1. Illustration of the experimental procedure in the present work. Step1: rHamPrP was treated with brain homogenate of scrapie-infected hamster 263K brain homogenate to produce rHamPrP fibrils (the method of QUICCitation14). Step 2: rHamPrP fibrils were treated with proteinase K under different pressures at 25 °C. Step 3. The reaction products were subjected to analysis with western blotting and AFM. See Materials and Methods for more details.
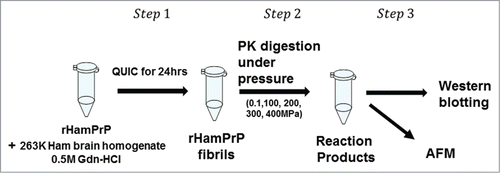
, far left, shows a representative AFM image of the rHaPrPres fibrils as prepared. The fibrils exhibit a heterogeneously aggregated morphological feature, similar to those reported for other prion fibrils.Citation16 The rest of the figures show representative AFM images of rHaPrPres fibrils treated with a high dose of PK for 1 h at pH 7.0 at 25 °C under different pressure conditions (0.1∼400 MPa). By treatment at 0.1 MPa for 1 h, fibrils remain, but effects were found on their shapes (, second from the left). By treatment with PK at increasing pressures, the fibrils diminish dramatically and disappear completely at 400 MPa (, far right).
Figure 2. Atomic force microscopy images of rHaPrPres fibrils PK-treated under different conditions. (A) A representative image of rHaPrPres fibrils as prepared by the method of QUICCitation14. (B) Representative images of rHaPrPres fibrils after PK-treatment for 1 h at respective pressures. See Materials and Methods for more details.
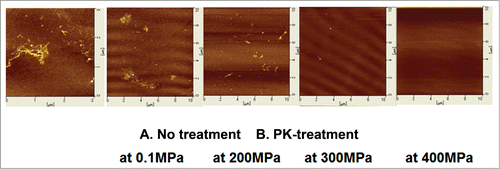
shows the western blotting patterns of the solution of monomeric rHaPrP (PrPC) (A) and those of the solution of rHaPrPres fibrils (B), treated similarly with a high dose of PK for 1 h at pH 7.0 at 25 °C under different pressure conditions (0.1∼400 MPa). For rHaPrP (PrPC) (), we note that the digestion by PK proceeds almost fully even at 0.1 MPa, but becomes complete above 100MPa. The accelerated digestion of monomeric proteins with a proteinase under pressure follows the general mechanism proposed for pressure-accelerated degradation of proteins.Citation17 For rHaPrPres fibrils (), we note that, at 0.1 MPa, the original 25 kDa band is replaced with the strong ∼12 kDa bands, indicating that residues 23 to 140 including the N-terminal flexible segment of rHaPrPres are selectively cleaved off, leaving fibrils consisting of “truncated” rHaPrPres monomers with only the C-terminal core part (residues ∼140–231).Citation3,14 This process of limited proteolysis of rHaPrPres is schematically illustrated in , in which the PK-resistant core parts of rHaPrPres are shown by gray column.
Figure 3. Western blotting of rHaPrP and rHaPrPres fibrils PK-treated under different pressures. (A) rHaPrP treated with proteinase K at different pressures at 25 °C for 1 h. (B) rHaPrPres fibrils treated with proteinase K at different pressures at 25 °C for 1 h See Materials and Methods for more details.
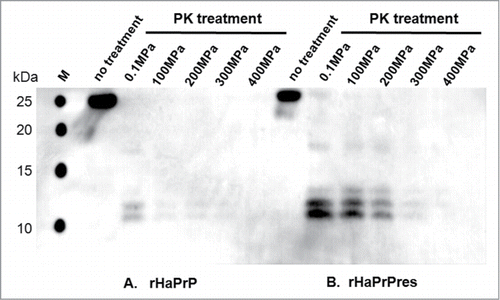
Figure 4. Illustration of the molecular events in the PK-treatment of rHaPrPres fibrils. (A) At 0.1 MPa, where no dissociation of fibrous rHaPrPres takes place, only the flexible N-terminal segments (residues 23 to 140) are cleaved off by proteinase K, leaving the fibril consisting only of the core parts (residues ∼141 to 231). (B) At high pressures (100–400 MPa), fibrous rHaPrPres dissociates into monomeric rHaPrP one by one, which is then readily degraded by proteinase K.
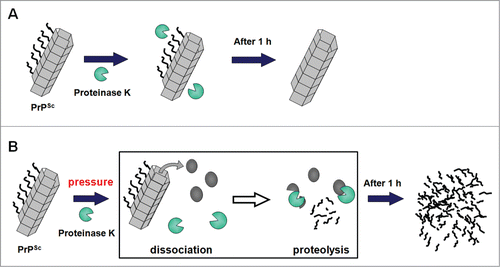
What is dramatic is the degradation of the ∼12 kDa bands, corresponding to the core part of rHaPrP, which start diminishing by treatment with PK at elevated pressures at 300–400 MPa (, right). The result clearly indicates that rHaPrPres fibrils are fully degraded into smaller peptide fragments by PK at elevated pressures. This result is consistent with the notion that rHaPrPres is dissociated into monomeric species under the elevated pressures, while PK remains active. The activity of PK at pressures (100–400 MPa) is verified by , in which an efficient degradation of rHaPrP (PrPC) with PK at 100–400 MPa is shown. The dramatic process of PK-degradation of rHaPrPres fibrils (PrPSc) is schematically illustrated in , in which the fibrillar PrPSc is dissociated into monomeric PrPC under elevated pressures, followed immediately by the efficient degradation of PrPC by proteinase K.
The entire process described above may be more adequately described thermodynamically in the following manner: The PrP fibrils (PrPSc) and PrP monomers, namely PrPC, are basically in dynamic equilibrium (PrPSc ⇌ PrPC). At 0.1 MPa, the equilibrium is strongly shifted toward the former (PrPSc). Under applied pressure, the equilibrium will be shifted more in favor of the latter (PrPC) by a factor anticipated by ∼exp (–PΔV/RT) with a negative value of ΔV = Vmonomer – Vfibril < 0.Citation5,6 The lower volume of the latter state (PrPC) would further be substantiated under pressure by pressure unfolding of PrPC (PrPC[folded] ⇌ PrPC[unfolded]).Citation17 The increased equilibrium population of PrP monomers, PrPC, under pressure would render the PrP fibrils degraded continually until they disappear totally, thanks to the dynamic equilibrium PrPSc ⇌ PrPC(folded) ⇌ PrPC(unfolded).
Implication of the Present Finding
The present experiment has shown that the normal cellular PrPC and the infectious form, PrPSc are interconvertible by pressure. Namely, an equilibrium holds basically between PrPSc and PrPC, namely PrPSc ⇌ PrPC. Accordingly, we may conclude that the crucial molecular event of the prion disease is also likely to be a thermodynamically controlled process.
This conclusion does not depend, in essence, on whether some complex reactions and/or intermediates are involved or not in this overall equilibrium above. The conclusion is particularly important, as it means that the reversible nature of the fibril-forming reaction, found in much simpler amyloidogenic protein systems so far studied,Citation9-13 is now extended to the highly heterogeneous assembly of fibrils of rHaPrPres such as those seen in . Furthermore, the present result, showing the increase in the monomer fraction with increasing pressure, implies that, under closely physiological conditions, the partial molar volume of the prion protein is smaller in the monomeric state, PrPC, than in the fibrous state, PrPSc, namely ΔV = Vmonomer – Vfibril < 0. This places pressure as a useful general tool for controlling the PrPC-PrPSc conversion, prompting us to carry on further detailed analysis of the conversion reaction between PrPC and PrPSc based on the thermodynamic and/or kinetic approach.
Technically, the method of pressure-accelerated proteolysis combined with western blotting introduced here is a novel approach generally applicable to the thermodynamic and kinetic analyses of various bio-macromolecular systems of high degree of association, for many of which a normal spectroscopic approach would fail because of the low sensitivity of detection and because of the slowness of the reaction at ambient conditions. In practice, the dramatic pressure effect on increasing the PK-digestibility of otherwise “PK-resistant” prion fibrils within a moderate range of pressure (< a few hundred MPa) suggests a simple and efficient means of eliminating infectious PrPSc from various systems in vitro.
Materials and Methods
Preparation of rHaPrPres fibrils
Fibrils of rHaPrPres were prepared from recombinant hamster prion protein (rHaPrP [23–231]) by shaking with brain homogenate of scrapie-infected hamster at 25 °C in 0.5 M Gdn-HCl, 150 mM NaCl, 150 mM HEPES buffer, pH 7.0 (QUIC).Citation14
Proteolysis of rHaPrPres
For the proteolysis reaction, the above solution containing rHamPrPres was diluted with pure water by 2.5-fold, making the final solution containing 40μg/ml of rHamPrPC, to which proteinase K was added to the concentration of 10μg /ml in 0.2 M Gdn-HCl, 60 mM NaCl, 60 mM HEPES, pH 7.0. Each 30μl of the above mixture, held in a tiny Teflon tube placed within a home-made pressure vessel, was subjected to a pressure ranging from 0.1 MPa to 400 MPa at 25 °C for 1 h. After the reaction was completed, 22μl of each solution was used for western blotting and the rest for atomic force microscopy (AFM) measurement.
Western blotting
The resultant solutions were subjected to western blotting with an anti PrP-goat polyclonal IgG, specific to residues 121–231 as primary antibody and alkaline phosphatase (AP) -anti-Goat IgG as secondary antibody. M refers to the lane for the molecular weight marker.
Atomic force microscopy
For each PK-treated samples, 2 μl of the solution was applied on a new mica surface, rinsed with pure water, and dried. AFM figures of fibrils were recorded with a cyclic contact mode at a frequency of 128 kHz on SPI-3800 scanning probe microscopy (Seiko Instruments Inc.).
Disclosure of Potential Conflicts of Interest
No potential conflicts of interest were disclosed.
Acknowledgments
This work was performed under the auspicies of the Academic Frontier Program 07F010 (Kinki University) and the Global COE Program (Nagasaki University) from the Ministry of Education, Culture, Sports, Science and Technology of Japan (MEXT) and supported in part by a Grant-in-Aid for Scientific Research (C) No.24570180. We thank I. Ichimiya of Science Technology Interact Co., LTD for her help in organizing the collaboration in this work.
References
- Prusiner SB. Prions. Proc Natl Acad Sci U S A 1998; 95:13363-83; PMID:9811807; http://dx.doi.org/10.1073/pnas.95.23.13363
- Bocharova OV, Makarava N, Breydo L, Anderson M, Salnikov VV, Baskakov IV. Annealing PrP fibrils at high temperature results in extension of a proteinase K resistant core. J Biol Chem 2006; 281:2373-9; PMID:16314415; http://dx.doi.org/10.1074/jbc.M510840200
- Race R, Jenny A, Sutton D. Scrapie infectivity and proteinase K-resistant prion protein in sheep placenta, brain, spleen, and lymph node: implications for transmission and antemortem diagnosis. J Infect Dis 1998; 178:949-53; PMID:9806020; http://dx.doi.org/10.1086/515669
- Akasaka K. Probing conformational fluctuation of proteins by pressure perturbation. Chem Rev 2006; 106:1814-35; PMID:16683756; http://dx.doi.org/10.1021/cr040440z
- Silva JL, Weber G. Pressure stability of proteins. Annu Rev Phys Chem 1993; 44:89-113; PMID:8257561; http://dx.doi.org/10.1146/annurev.pc.44.100193.000513
- Royer CA. Revisiting volume changes in pressure-induced protein unfolding. Biochim Biophys Acta 2002; 1595:201-9; PMID:11983396; http://dx.doi.org/10.1016/S0167-4838(01)00344-2
- Cordeiro Y, Kraineva J, Winter R, Silva JL. Volume and energy folding landscape of prion protein revealed by pressure. Braz J Med Biol Res 2005; 38:1195-201; PMID:16082459; http://dx.doi.org/10.1590/S0100-879X2005000800006
- Sasaki K, Gaikwad J, Hashiguchi S, Kubota T, Sugimura K, Kremer W, Kalbitzer HR, Akasaka K. Reversible monomer-oligomer transition in human prion protein. Prion 2008; 2:118-22; PMID:19158507; http://dx.doi.org/10.4161/pri.2.3.7148
- Akasaka K, Latif AR, Nakamura A, Matsuo K, Tachibana H, Gekko K. Amyloid protofibril is highly voluminous and compressible. Biochemistry 2007; 46:10444-50; PMID:17715944; http://dx.doi.org/10.1021/bi700648b
- Niraula TN, Konno T, Li H, Yamada H, Akasaka K, Tachibana H. Pressure-dissociable reversible assembly of intrinsically denatured lysozyme is a precursor for amyloid fibrils. Proc Natl Acad Sci U S A 2004; 101:4089-93; PMID:15016916; http://dx.doi.org/10.1073/pnas.0305798101
- Kamatari YO, Yokoyama S, Tachibana H, Akasaka K. Pressure-jump NMR study of dissociation and association of amyloid protofibrils. J Mol Biol 2005; 349:916-21; PMID:15907935; http://dx.doi.org/10.1016/j.jmb.2005.04.010
- Abdul Latif AR, Kono R, Tachibana H, Akasaka K. Kinetic analysis of amyloid protofibril dissociation and volumetric properties of the transition state. Biophys J 2007; 92:323-9; PMID:16997869; http://dx.doi.org/10.1529/biophysj.106.088120
- Shah BR, Maeno A, Matsuo H, Tachibana H, Akasaka K. Pressure-accelerated dissociation of amyloid fibrils in wild-type hen lysozyme. Biophys J 2012; 102:121-6; PMID:22225805; http://dx.doi.org/10.1016/j.bpj.2011.10.041
- Atarashi R, Wilham JM, Christensen L, Hughson AG, Moore RA, Johnson LM, Onwubiko HA, Priola SA, Caughey B. Simplified ultrasensitive prion detection by recombinant PrP conversion with shaking. Nat Methods 2008; 5:211-2; PMID:18309304; http://dx.doi.org/10.1038/nmeth0308-211
- Akasaka K, Yamada H. (2001) On-line cell high pressure nuclear magnetic resonance technique: Application to protein studies. In: James TL, Ed.(2001) Methods in Enzymology 338, Academic Press, New York, pp 134-158.
- Torrent J, Alvarez-Martinez MT, Harricane MC, Heitz F, Liautard JP, Balny C, Lange R. High pressure induces scrapie-like prion protein misfolding and amyloid fibril formation. Biochemistry 2004; 43:7162-70; PMID:15170353; http://dx.doi.org/10.1021/bi049939d
- Akasaka K, Nagahata H, Maeno A, Sasaki K. Pressure acceleration of proteolysis. A general mechanism. Biophysics (Oxf) 2008; 4:29-32; http://dx.doi.org/10.2142/biophysics.4.29