Abstract
A typical feature of scrapie in sheep and goats is the accumulation of disease-associated prion protein. Scrapie consists of many strains with different biological properties. Nine natural sheep scrapie cases were transmitted to wild-type mice and mouse-passaged isolates were classified into 2 types based on incubation time: short and long. These 2 types displayed a distinct difference in their pathology. We attempted to transmit these mouse-passaged isolates to 2 murine cell lines (GT1–7 and L929) to compare their properties. All of the isolates were transmitted to L929 cells. However, only mouse-passaged field isolates with a long incubation time were transmitted to GT1–7 cells. This specific susceptibility of GT1–7 cells was also confirmed with a primary-passaged isolate that was not completely adapted to the new host species. Characterization of the mechanisms of the specific susceptibility of GT1–7 cells to isolates with a long incubation time may lead to a greater understanding of the differences among prion strains.
Introduction
Scrapie is a transmissible spongiform encephalopathy (TSE) in sheep and goats. The central event of TSE pathogenesis is the accumulation of the disease-associated isoform of the prion protein (PrPSc), which is thought to be the primary or sole component of the TSE agent (or prion).Citation1 PrPSc is generated by post-translational modification of a host-encoded cellular prion protein (PrPC), but they have different conformational and physicochemical properties.Citation2,3 The infectious agent itself remains enigmatic.Citation4
Scrapie prions are classified into many biologically different strains and more than 20 strains have been reported.Citation5 Prion strains are classified according to biological properties such as incubation time, histopathological lesion profile, and PrPSc deposition pattern in inbred mice.Citation6-8 No scrapie prions have been transmitted to humans. However, the detection of bovine spongiform encephalopathy (BSE) in goatsCitation9,10 has increased the need for surveillance and strain-typing of natural scrapie.
Some prion strains can be discriminated by the biochemical properties of PrPSc, such as molecular mass, glycoform ratio, proteinase K (PK) sensitivity,Citation11-13 and conformation stability.Citation14,15 However, some scrapie isolates have shown different biological properties irrespective of their similar PrPSc banding pattern.Citation16,17
We examined the characteristics of natural sheep scrapie cases by mouse transmission. Ten mouse-passaged scrapie isolates were obtained and classified into 2 types. One had a short incubation period and caused prominent vacuolation in affected mice (short-type). In contrast, the other had a long incubation period and caused relatively mild vacuolation in affected mice (long-type).
Several cell lines are known to show susceptibility to mouse-adapted prions.Citation18-21 By using these cell lines, a couple of methods have been developed for the discrimination of prion strains and the evaluation of prion infectivity.Citation22,23 GT1–7 cells have been used in prion research and exhibit susceptibility to a variety of mouse-adapted prion strains and stable PrPSc replication.Citation24-27 However, this cell line is insusceptible to the laboratory scrapie strain ME7.Citation28 In contrast, L929 cells exhibit susceptibility to several laboratory scrapie strains, including ME7.Citation28
In this study, we examined the susceptibility of GT1–7 cells and L929 cells to 2 types of mouse-passaged scrapie isolates. Both types of isolates were transmitted to L929 cells. In contrast, long-type isolates were transmitted to GT1–7 cells, but short-type isolates were not. This difference in transmissibility to GT1–7 cells was confirmed not only for prions from the subsequent passaged mice (2nd, 3rd or serial passage), but also in primary passaged mice.
Results
Classification of natural sheep scrapie isolates into 2 types
Nine Japanese natural scrapie cases were transmitted to wild-type (ICR) mice and 10 isolates were obtained. These isolates were categorized into 2 types based on their biological properties. One type displayed a short incubation time, marked vacuolation and widespread PrPSc distribution throughout the brain in mice (designated as short-type). Seven isolates (Obihiro,Citation29 Tsukuba-1,Citation16 Tok,Citation30 Tok-S24, Tok-S31, Ka/WCitation17 and Fukuoka) were classified as short-type isolates (). Based on their incubation times, the lesion profiles and the distribution of PrPSc, these isolates had characteristics reminiscent of ME7 (, K and ). The other type displayed polydipsia and polyuria with a long incubation time in diseased mice (designated as long-type). Three isolates, (Tsukuba-2,Citation16 Ka/OCitation17 and Oita) were classified as long-type isolates (). The lesion profiles of mice affected with long-type isolates were obviously different from those of mice affected with short-type isolates (). Mice affected with short-type isolates displayed fine and coarse granular PrPSc and prominent vacuolation throughout the brain (). In contrast, PrPSc of mice affected with long-type isolates were mainly observed in the thalamus, hypothalamus, and brainstem and some PrPSc plaques were seen in the corpus callosum (). In spite of different biological properties, there was no difference in PrPSc banding pattern between short-type and long-type isolates ().
Table 1. Mouse-adapted field scrapie isolates used in this study
Figure 1. Characterization of 2 types of mouse-passaged scrapie isolates. Immunohistochemical detection of PrPSc was performed using the monoclonal antibody (mAb) SAF84. The coronal sections at the level of the hippocampus of brains affected with a short-type isolate (A) and a long-type isolate (F) are shown, respectively. Sections of the hippocampus of mice affected with Fukuoka (B and C), ME7 (D and E), Oita (G and H) and Tsukuba-2 (I and J) were subjected to H&E staining (B, D, G and I) and immunostaining of PrPSc (C, E, H and J). The bars represent 200 μm. Lesion profiles of mice affected with ME7, Obihiro, Tok, Fukuoka, Tsukuba-2 and Oita in 9 brain areas are shown (K). The brain vacuolation of mice affected with ME7 (closed circle, n = 6), Obihiro (gray opened circle, n = 6), Tok (blue opened circle, n = 3), Fukuoka (red opened circle, n = 6), Tsukuba-2 (closed diamond, n = 5), and Oita (opened diamond, n = 6) were scored on a scale of 0 to 5 (mean value + standard deviation). Serially passaged brains of ME7- and Obihiro-affected mice, second-passaged brains of Tok-, Fukuoka- and Oita-affected mice, and third-passaged brains of Tsukuba-2-affected mice were subjected to the scoring. The different brain areas are indicated as follows: 1 dorsal medulla, 2 cerebellar cortex, 3 superior cortex, 4 hypothalamus, 5 thalamus, 6 hippocampus, 7 septal nuclei of the paraterminal body, 8 cerebral cortex at the levels of the hypothalamus and the thalamus, 9 cerebral cortex at the level of the septal nuclei of the paraterminal body. Representative western blot of PrPSc in mice affected with a variety of mouse-passaged field scrapie isolates is shown (L). The name of the isolates and respective grouping into short- and long-type are indicated on the top of each lane. Total protein (10 μg) was loaded per lane and probed with mAb SAF84 to detect PrPSc. Molecular markers are indicated on the left side of the panel. Glycoform profiles of PrPSc in mouse brains affected with 7 mouse-passaged scrapie isolates (M). PrPSc was detected with mAb SAF84. Signal intensities of di-, mono-, and non-glycosylated PrPSc bands were analyzed. Black bar: ratio of diglycosylated PrPSc to total PrPSc. Gray bar: ratio of mono-glycosylated PrPSc to total PrPSc. White bar: ratio of non-glycosylated PrPSc to total PrPSc. Values are expressed as the mean ± standard deviation (%).
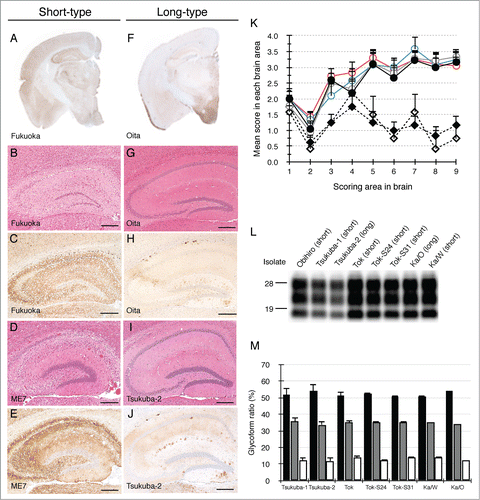
Transmission study of mouse-passaged field scrapie isolates to GT1–7 and L929 cell lines
In order to ascertain the transmissibility of the 2 types of isolates to murine cell lines, diseased mouse brain homogenates were exposed to GT1–7 cells and L929 cells, respectively. Interestingly, all the examined long-type isolates (Tsukuba-2,Citation16 Ka/OCitation17 and Oita) were successfully transmitted to GT1–7 cells and synthesized de novo PrPSc (), whereas all the examined short-type isolates were not transmitted, except for the laboratory strains Chandler and 22L ( and ). In contrast, L929 cells were susceptible to both short-type (Ka/W and Fukuoka) and long-type (Ka/O and Oita) isolates and synthesized de novo PrPSc ( and Table 1).
Figure 2. Transmission of short-type and long-type isolates to murine cell lines. Representative protein gel blots of GT1–7 cells exposed to short-type and long-type isolates are shown (A and B). The name of the isolate used for the challenge and respective grouping into short- and long-type are indicated on the top of each lane. Nl indicates the use of an uninfected mouse brain homogenate as the inoculum. Chandler strain was used for the positive control of the GT1–7 infection. Representative western blot of L929 cells exposed to short-type and long-type isolates is shown (C). The name of the isolate used for the challenge and respective grouping into short- and long-type are indicated on the top of each lane. Total protein (500 μg) was loaded into each lane except for the lane of Chandler (80 μg). The presence of PrPSc was analyzed after 7 passages and more to eliminate the influence of PrPSc derived from the brain homogenates. PrPSc was detected with mAb T2. Molecular markers are indicated on the left side of each panel.
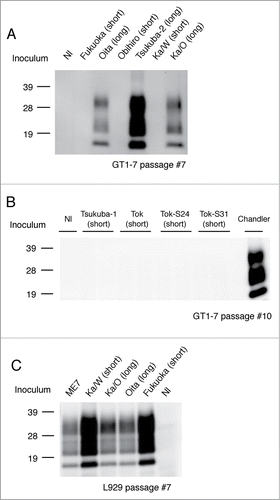
Specific detection of long-type isolates in primary-passaged mice by GT1–7 cells
The specific response of GT1–7 cells against long-type isolates was examined using primary-passaged mouse brains affected with Fukuoka or Oita scrapie. Both scrapie isolates were transmissible to ICR mice with a 100% attack rate and their incubation times were 442 ± 62.4 d (Fukuoka) and 447 ± 33.7 d (Oita), respectively, at primary passage (). As shown in , the distribution patterns of PrPSc in the brains of the mice affected with these 2 isolates were alike and PrPSc was mainly detected in the hypothalamus of both isolates of scrapie-affected mice. Neuropathological analysis also demonstrated similar lesion profiles in primary-passaged mice (). In addition, both isolates displayed indistinguishable biochemical properties of PrPSc, including PK-sensitivity, glycoform profile, and conformational stability ( and ). No clear difference was observed in the primary-passaged mice of Fukuoka and Oita scrapie. However, subsequent passages revealed differences in their biological properties: Fukuoka isolate was classified as a short-type and Oita isolate was classified as a long-type isolate ( and ).
Table 2. Conformation stability of PrPSc of mice affected with Fukuoka and Oita at primary passage
Figure 3. Biochemical and biological properties of short- and long-type prions in primary-passaged mouse brains. PrPSc distributions of primary-passaged Fukuoka and Oita affected mice are shown, respectively (A and B). Immunostaining was performed using the mAb SAF84. The coronal sections at the level of the hippocampus are shown. Lesion profiles of Fukuoka- and Oita-affected mice in 9 brain areas at first passage (C). The brain vacuolation of Fukuoka- (closed circle) and Oita-affected mice (opened circle) was scored on a scale of 0 to 5 (mean value ± standard deviation). The brain areas are as indicated in . Representative protein gel blot of PrPSc in Fukuoka- and Oita-affected mice (D). The name of the isolate and passage number in mice are indicated on the top of panel. For each sample, 10 μg of total protein was loaded per lane and probed with mAb SAF84 to detect PrPSc. Molecular markers are indicated on the left side. PK sensitivity of PrPSc between Fukuoka- and Oita-affected mice at first passage is compared (E). Closed circles and opened circles represent the results of Fukuoka- and Oita-affected mice, respectively. PrPSc was detected with mAb 6H4. Data represent the mean ± standard deviation from 4 to 5 independent experiments. Glycoform profiles of PrPSc in Fukuoka- and Oita-affected mice at first passage (F). PrPSc was detected with mAb 6H4. Signal intensities of di-, mono-, and non-glycosylated PrPSc bands were analyzed. Black bar: ratio of diglycosylated PrPSc to total PrPSc. Gray bar: ratio of mono-glycosylated PrPSc to total PrPSc. White bar: ratio of non-glycosylated PrPSc to total PrPSc. Values are expressed as the mean ± standard deviation (%). Representative western blot of GT1–7 cells exposed to Fukuoka (short-type isolate) and Oita (long-type isolate) is shown (G). Inocula used in this study are indicated on the top of each lane. Nl indicates the use of an uninfected mouse brain homogenate as the inoculum. PrPSc was detected by mAb T2. Molecular markers are indicated on the left side of panel. Total protein (500 μg) was loaded into each lane except for the lane of Chandler (80 μg).
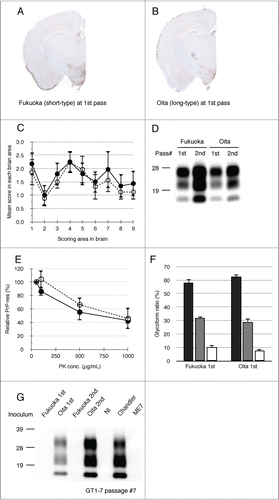
As shown in , PrPSc was detected from Oita-challenged GT1–7 cells at primary and secondary passage (lane 2 and 4). In contrast, no PrPSc was detected from Fukuoka-challenged cells (lane 1 and 3). GT1–7 cells were preferably permissive only to the Oita isolate and produced de novo PrPSc, irrespective of their passage numbers. These results indicate that the transmissibility of mouse-passaged isolates to cell lines is determined prior to their biological adaptation to the new host.
Discussion
Prion strain typing is based on the incubation time and lesion profile of mouse-adapted isolates.Citation5,7 In the interspecies transmission of prions, a couple of passage histories are required to stabilize properties such as incubation time, brain pathology, and topology of accumulated PrPSc.Citation5,12 In this study, we transmitted 9 natural sheep scrapie cases to ICR mice to explore the extent of phenotypic variation and obtained 10 mouse-passaged isolates. No distinct difference was observed in PrPSc banding pattern and glycoprofile among all isolates. Thus, to distinguish these isolates, biological analysis using mouse bioassays were necessary. The isolates were categorized into 2 types. The short-type isolates that were examined were all reminiscent of ME7 by their biological characterization (). The incubation times of the laboratory scrapie strains 22L and Chandler in ICR mice were approximately 140 d () and were similar to those of short-type isolates in this study. GT1–7 cells are known to be insusceptible to ME7, but susceptible to 22L and Chandler.Citation28 Therefore, the insusceptibility of GT1–7 cells to the examined short-type isolates also suggests similarity of these Japanese isolates with ME7.
Although present and previous studies used different mouse lines with the same PrP allele (ICR and C57BL), the pathology of mice affected with long-type isolates was similar with the reported pathology of mouse-passaged scrapie strain 87A.Citation31,32 There is no report regarding the susceptibility of GT1–7cells to mouse-passaged scrapie isolates with a long incubation time. Our results indicate the possibility that GT1–7 cells may be susceptible to 87A. Indeed, it has been reported that GT1–7 cells are susceptible to a mouse-passaged sporadic Creutzfeldt-Jakob isolate that shows a long incubation time in ICR mice.Citation33 This cell line will be a useful tool for the analysis of slowly propagated prions in the in vivo environment.
GT1–7 cells could amplify PrPSc of a long-type isolate from the primary-passaged mice, even though the isolate did not yet adapt to the new host. Furthermore, mice affected with long-type isolates showed pathology similar to mice affected with short-type isolates at this stage (). A PrPSc tracing experiment using a conformation-specific monoclonal antibody revealed that the PrPSc structure was altered during prion adaptation to the new host animals.Citation34 Our study demonstrated that the susceptibility of prions to GT1–7 cells was independent from this conformational transition of PrPSc in interspecies transmission. This isolate-specific response of GT1–7 cells will be useful as a time- and cost-saving alternative to the mouse bioassay.
Since ICR mice, GT1–7 cells and L929 cells express the same PrP alleles, the difference in susceptibility to long-type isolates between GT1–7 cells and L929 cells is not associated with the amino acid sequence of PrP. Though the precise mechanisms of the altered susceptibility of GT1–7 cells to some mouse-passaged scrapie isolates are unknown, conformational differences of PrPSc among strains and/or isolates are considerable. The different susceptibility of GT1–7 cells to RML and ME7 could be accounted for by the different conformation of PrPSc.Citation14 Another technique will be necessary to detect the structural differences of PrPSc between long-type and short-type isolates. Another possibility is structural differences of PrPC between GT1–7 cells and L929 cells. It also may link to the PrPSc tropisms in mouse prion strains. The different origin of 2 cell lines, hypothalamic neurons (GT1–7 cells)Citation35 and fibroblasts (L929 cells)Citation20 may contribute to this difference.
In vitro classification of prion strains by the use of several cell lines has been reported.Citation36,37 Our results also indicate that the isolate-specific response of GT1–7 cells could work from the primary-passaged mice. The specific amplification of prion in the cell culture may be useful for the cloning and/or isolation of a strain. Clarification of the molecular mechanisms involved in this phenomenon may lead to a better understanding of prion pathogenesis.
Materials & Methods
Scrapie sources
The mouse-passaged field scrapie isolates used in this study are listed in . The details of some of those isolates were described in previous reports.Citation16,17,29,30 Rodent-passaged laboratory scrapie strains (Chandler, 22L and ME7) were maintained by serial-passage into wild-type mice (ICR; Japan SLC, Hamamatsu, Japan).Citation38 All experiments involving animals were approved by the Animal Ethical Committee and the Animal Care and Use Committee of the National Institute of Animal Health (authorization 11–008 and 11–012).
Scrapie transmission to wild-type mice
Twenty microliter of 10% (w/v) brain homogenate from the diseased sheep was intracerebrally inoculated into 3-week-old female ICR mice. Mouse brains were collected at the terminal stage of the disease or after death and subjected to biological and biochemical analyses and subsequent transmission to wild-type mice. Incubation was measured as the time between inoculation and the clinical endpoint or death. All brains of euthanized and dead mice were examined for the presence of PrPSc by Western blot.
Transmission of mouse-passaged field scrapie isolates to GT1–7 and L929 cell lines
GT1–7 cells were maintained and exposed to mouse- passaged scrapie prions as described previously.Citation39 Briefly, cells were grown on 6-well plates at 10Citation5 cells/well. After 2 d of incubation, cells were exposed to 0.1% (w/v) brain homogenate in medium (1 mL) for 1 day. Additionally, 1 mL of plain medium was added to each well. Cells were incubated for 1 day and seeded into new 6-well plates for the first passage (P1). L929 cells were maintained in Dulbecco's modified eagle medium adding 10% fetal bovine serum and exposed to mouse- passaged scrapie prions as described above. For subsequent passages, GT1–7 and L929 cells were seeded at a 1:4 and 1:10 ratio every 4 days, respectively. In order to eliminate the influence of PrPSc in the exposed brain homogenate and stabilize PrPSc accumulation in cells, the presence of PrPSc in cells was analyzed after 7 passages and more.
Histopathology and immunohistochemistry
The right lobes of brains were fixed in 10% neutral buffered formalin (pH 7.4) containing 10% methanol overnight at 37°C. Coronal slices of the brains were immersed in 98% formic acid for 60 min at room temperature (RT) to reduce infectivity and then embedded in paraffin wax. Serial sections were stained with hematoxylin and eosin (H&E) for neuropathological changes including the lesion profileCitation6 or for immunohistochemistry. After epitope retrieval, PrPSc immunohistochemistry was performed using the mouse monoclonal antibody (mAb) SAF84 (Bertin Pharma, Montigny le Bretonneux, France).Citation40 Immunoreactions were developed using the anti-mouse, universal immunoperoxidase polymer (Nichirei Histofine Simple Stain MAX-PO (M); Nichirei, Tokyo, Japan) as the secondary antibody and were visualized with 3,3′-diaminobenzedine tetrachloride as the chromogen.
PrPSc detection from brains and cells
Brains were homogenized in 4 volumes of buffer containing 150 mM NaCl and 50 mM Tris-HCl (pH 7.6) to obtain 20% w/v, and were stored at −80°C until use. For the detection of PrPSc, 250 μL of 5% (w/v) brain homogenate was mixed with an equal volume of detergent buffer containing 4% (w/v) Zwittergent 3–14, 1% (w/v) Sarkosyl, 100 mM NaCl, and 50 mM Tris-HCl (pH 7.6), and then treated with 1.6 μL of 80 mg/mL collagenase and 1 μL of 10 mg/mL DNase I for 30 min at 37°C. To digest PrPC, the sample was treated with 1 μL of 20 mg/mL PK (40 μg/mL final concentration) (Roche Diagnostics, Basel, Switzerland) for 30 min at 37°C then the digestion was terminated by adding 2.5 μL of 0.4 M 4-(2-aminoethyl)-benzenesulfonyl fluoride hydrochloride (Pefablock; Roche Diagnostics). The sample was then mixed with a 2-butanol: methanol mixture (5:1) and centrifuged at 20,000 × g for 10 min at RT. The pellet was resuspended in SDS-sample buffer and boiled for 5 min. Then, the samples were then subjected to western blot analysis.
In order to detect PrPSc in cells, confluent cells were washed with PBS and then lysed with lysis buffer containing 50 mM Tris-HCl [pH 7.6], 150 mM NaCl, 0.5% (w/v) Triton X-100, 0.5% (w/v) sodium deoxycholate, and 5 mM EDTA. After 2 min of centrifugation at 6,500 × g at 4°C, the supernatant was collected and the total protein concentration was measured using the Bio-Rad protein assay (Bio-Rad Laboratories, Hercules, CA). The protein concentration of each cell lysate was adjusted to 1 mg/mL and the samples were treated with 2 μL of 20 mg/mL PK (40 μg/mL final concentration) at 37°C for 30 min. Following PK digestion, the samples were mixed with a 2-butanol: methanol mixture (5:1) and centrifuged at 20,000 × g for 10 min at RT. The pellet was solubilized in SDS-sample buffer and boiled for 5 min. The samples were then subjected to Western Blot analysis.
Brain and cell samples were loaded onto a 12% polyacrylamide gel. The proteins were transferred onto an Immobilon-P membrane (Millipore, Billerica, MA). The blotted membrane was incubated with anti-PrP mAbs T2,Citation41 6H4 (Prionics, Zurich, Switzerland) and SAF84 at 4°C overnight. Following twice washing with PBST, the membrane was incubated with horseradish peroxidase (HRP)-conjugated anti-mouse IgG (Jackson ImmunoResearch, West Grove, PA) for 1 h at RT. Signals were developed with a chemiluminescent substrate (SuperSignal; Thermo Fisher Scientific, Rockland, IL). Blots were imaged with Fluochem (Alpha Innotech, San Leandro, CA) and analyzed with ImageReader software (AlphaEaseFC; Alpha Innotech) according to the manufacturer's instructions.
PK-sensitivity assay
Brain samples were incubated with various PK concentrations (50, 100, 500, and 1000 μg/mL) at 37°C for 30 min. Samples were subjected to Western Blot as described above. PrPSc signals were detected with mAb 6H4 and normalized by the signal intensity of a 50 μg/mL PK-treated sample.
Conformational Stability Assay
The conformational stability assay was performed as previously described.Citation42 Briefly, 25 μL of 10% (w/v) brain homogenate was added to an equal volume of guanidine hydrochloride (GdnHCl) in the concentration range of 1–8 M. Samples were mixed well and incubated at 37°C for 1 h. Then, the sample was diluted by the addition of 425 μL of a buffer containing 10 mM Tris-HCl (pH 8.0), 150 mM NaCl, 0.5% (w/v) Triton-X 100, and 0.5% (w/v) deoxycholate. Then, 25 μL of GdnHCl was added to each sample to a final concentration of 0.4 M. Following GdnHCl treatment, samples were digested with 20 μg/mL PK at 37°C for 1 h. PrPSc was precipitated and subjected to Western Blot analysis. PrPSc signals were detected using mAb SAF84 and normalized by the signal intensity of the 0.5 M GdnHCl-treated sample. The data were fitted into a sigmoidal dose-response curve using the KaleidaGraph software (Synergy Software, Reading, PA). Then, the half maximal effective concentrations of GdnHCl ([GdnHCl]1/2, M) were calculated.
Acknowledgments
We are grateful to Naoko Tabeta, Ritsuko Miwa, Naomi Furuya, Junko Yamada, and the animal laboratory staff of NIAH for their technical and animal care assistance.
Funding
This work was supported by a Grant-in-Aid from the BSE and other Prion Disease Control Project of the Ministry of Agriculture, Forestry, and Fisheries, and in part by Grants-in-Aid from the Ministry of Health, Labor, and Welfare of Japan and in part by a Grant-in-Aid for Young Scientists (Category B) from the Ministry of Education, Culture, Sports, Science, and Technology of Japan.
References
- Prusiner SB. Novel proteinaceous infectious particles cause scrapie. Science 1982; 216:136-44; PMID: 6801762; http://dx.doi.org/10.1126/science.6801762
- Bueler H, Aguzzi A, Sailer A, Greiner RA, Autenried P, Aguet M, Weissmann C. Mice devoid of PrP are resistant to scrapie. Cell 1993; 73:1339-47; PMID:8100741; http://dx.doi.org/10.1016/0092-8674(93)90360-3
- Prusiner SB. Molecular biology of prion diseases. Science 1991; 252:1515-22; PMID:1675487; http://dx.doi.org/10.1126/science.1675487
- Manuelidis L. Infectious particles, stress, and induced prion amyloids: a unifying perspective. Virulence 2013; 4:373-83; PMID:23633671; http://dx.doi.org/10.4161/viru.24838
- Bruce ME. TSE strain variation. Br Med Bull 2003; 66:99-108; PMID:14522852; http://dx.doi.org/10.1093/bmb/66.1.99
- Fraser H, Dickinson AG. The sequential development of the brain lesion of scrapie in three strains of mice. J Comp Pathol 1968; 78:301-11; PMID:4970192; http://dx.doi.org/10.1016/0021-9975(68)90006-6
- Bruce ME. Scrapie strain variation and mutation. Br Med Bull 1993; 49:822-38; PMID:8137131
- Bruce ME, McBride PA, Farquhar CF. Precise targeting of the pathology of the sialoglycoprotein, PrP, and vacuolar degeneration in mouse scrapie. Neurosci Lett 1989; 102:1-6; PMID:2550852; http://dx.doi.org/10.1016/0304-3940(89)90298-X
- Eloit M, Adjou K, Coulpier M, Fontaine JJ, Hamel R, Lilin T, Messiaen S, Andreoletti O, Baron T, Bencsik A, et al. BSE agent signatures in a goat. Vet Rec 2005; 156:523-4; PMID:15833975
- Spiropoulos J, Lockey R, Sallis RE, Terry LA, Thorne L, Holder TM, Beck KE, Simmons MM. Isolation of prion with BSE properties from farmed goat. Emerg Infect Dis 2011; 17:2253-61; PMID:22172149; http://dx.doi.org/10.3201/eid1712.110333
- Bessen RA, Marsh RF. Biochemical and physical properties of the prion protein from two strains of the transmissible mink encephalopathy agent. J Virol 1992; 66:2096-101; PMID:1347795
- Somerville RA, Chong A, Mulqueen OU, Birkett CR, Wood SC, Hope J. Biochemical typing of scrapie strains. Nature 1997; 386:564; PMID:9121579; http://dx.doi.org/10.1038/386564a0
- Collinge J, Sidle KC, Meads J, Ironside J, Hill AF. Molecular analysis of prion strain variation and the aetiology of ‘new variant’ CJD. Nature 1996; 383:685-90; PMID:8878476; http://dx.doi.org/10.1038/383685a0
- Thackray AM, Hopkins L, Klein MA, Bujdoso R. Mouse-adapted ovine scrapie prion strains are characterized by different conformers of PrPSc. J Virol 2007; 81:12119-27; PMID:17728226; http://dx.doi.org/10.1128/JVI.01434-07
- Safar J, Wille H, Itri V, Groth D, Serban H, Torchia M, Cohen FE. Eight prion strains have PrP(Sc) molecules with different conformations. Nat Med 1998; 4:1157-65; PMID:9771749; http://dx.doi.org/10.1038/2654
- Hirogari Y, Kubo M, Kimura KM, Haritani M, Yokoyama T. Two different scrapie prions isolated in Japanese sheep flocks. Microbiol Immunol 2003; 47:871-6; PMID:14638998; http://dx.doi.org/10.1111/j.1348-0421.2003.tb03453.x
- Masujin K, Shu Y, Okada H, Matsuura Y, Iwamaru Y, Imamura M, Mohri S, Yokoyama T. Isolation of two distinct prion strains from a scrapie-affected sheep. Arch Virol 2009; 154:1929-32; PMID:19876594; http://dx.doi.org/10.1007/s00705-009-0534-2
- Rubenstein R, Carp RI, Callahan SM. In vitro replication of scrapie agent in a neuronal model: infection of PC12 cells. J Gen Virol 1984; 65 (Pt 12):2191-8; PMID:6439820; http://dx.doi.org/10.1099/0022-1317-65-12-2191
- Butler DA, Scott MR, Bockman JM, Borchelt DR, Taraboulos A, Hsiao KK, Kingsbury DT, Prusiner SB. Scrapie-infected murine neuroblastoma cells produce protease-resistant prion proteins. J Virol 1988; 62:1558-64; PMID:3282080
- Vorberg I, Raines A, Story B, Priola SA. Susceptibility of common fibroblast cell lines to transmissible spongiform encephalopathy agents. J Infect Dis 2004; 189:431-9; PMID:14745700; http://dx.doi.org/10.1086/381166
- Iwamaru Y, Takenouchi T, Ogihara K, Hoshino M, Takata M, Imamura M, Tagawa Y, Hayashi-Kato H, Ushiki-Kaku Y, Shimizu Y, et al. Microglial cell line established from prion protein-overexpressing mice is susceptible to various murine prion strains. J Virol 2007; 81:1524-7; PMID:17121794; http://dx.doi.org/10.1128/JVI.01379-06
- Klohn PC, Stoltze L, Flechsig E, Enari M, Weissmann C. A quantitative, highly sensitive cell-based infectivity assay for mouse scrapie prions. Proc Natl Acad Sci U S A 2003; 100:11666-71; PMID:14504404; http://dx.doi.org/10.1073/pnas.1834432100
- Neale MH, Mountjoy SJ, Edwards JC, Vilette D, Laude H, Windl O, Saunders GC. Infection of cell lines with experimental and natural ovine scrapie agents. J Virol 2010; 84:2444-52; PMID:20032176; http://dx.doi.org/10.1128/JVI.01855-09
- Liu Y, Sun R, Chakrabarty T, Manuelidis L. A rapid accurate culture assay for infectivity in Transmissible Encephalopathies. J Neurovirol 2008; 14:352-61; PMID:18989813; http://dx.doi.org/10.1080/13550280802105283
- Manuelidis L, Liu Y, Mullins B. Strain-specific viral properties of variant Creutzfeldt-Jakob disease (vCJD) are encoded by the agent and not by host prion protein. J Cell Biochem 2009; 106:220-31; PMID:19097123; http://dx.doi.org/10.1002/jcb.21988
- Manuelidis L, Chakrabarty T, Miyazawa K, Nduom NA, Emmerling K. The kuru infectious agent is a unique geographic isolate distinct from Creutzfeldt-Jakob disease and scrapie agents. Proc Natl Acad Sci U S A 2009; 106:13529-34; PMID:19633190; http://dx.doi.org/10.1073/pnas.0905825106
- Miyazawa K, Emmerling K, Manuelidis L. Replication and spread of CJD, kuru and scrapie agents in vivo and in cell culture. Virulence 2011; 2:188-99; PMID:21527829; http://dx.doi.org/10.4161/viru.2.3.15880
- Grassmann A, Wolf H, Hofmann J, Graham J, Vorberg I. Cellular aspects of prion replication in vitro. Viruses 2013; 5:374-405; PMID:23340381; http://dx.doi.org/10.3390/v5010374
- Shinagawa M, Matsuda A, Sato G, Takeuchi M, Ichijo S, Ono T. Occurrence of ovine scrapie in Japan: clinical and histological findings in mice inoculated with brain homogenates of an affected sheep. Nihon Juigaku Zasshi 1984; 46:913-6; PMID:6441054; http://dx.doi.org/10.1292/jvms1939.46.913
- Shimada K, Hayashi HK, Ookubo Y, Iwamaru Y, Imamura M, Takata M, Schmerr MJ, Shinagawa M, Yokoyama T. Rapid PrP(Sc) detection in lymphoid tissue and application to scrapie surveillance of fallen stock in Japan: variable PrP(Sc) accumulation in palatal tonsil in natural scrapie. Microbiol Immunol 2005; 49:801-4; PMID:16113510; http://dx.doi.org/10.1111/j.1348-0421.2005.tb03660.x
- Bruce ME, Dickinson AG. Biological evidence that scrapie agent has an independent genome. J Gen Virol 1987; 68:79-89; PMID:3100717; http://dx.doi.org/10.1099/0022-1317-68-1-79
- Thackray AM, Hopkins L, Lockey R, Spiropoulos J, Bujdoso R. Emergence of multiple prion strains from single isolates of ovine scrapie. J Gen Virol 2011; 92:1482-91; PMID:21270287; http://dx.doi.org/10.1099/vir.0.028886-0
- Nishida N, Katamine S, Manuelidis L. Reciprocal interference between specific CJD and scrapie agents in neural cell cultures. Science 2005; 310:493-6; PMID:16239476; http://dx.doi.org/10.1126/science.1118155
- Ushiki-Kaku Y, Endo R, Iwamaru Y, Shimizu Y, Imamura M, Masujin K, Yamamoto T, Hattori S, Itohara S, Irie S, et al. Tracing conformational transition of abnormal prion proteins during interspecies transmission by using novel antibodies. J Biol Chem 2010; 285:11931-6; PMID:20177064; http://dx.doi.org/10.1074/jbc.M109.058859
- Mellon PL, Windle JJ, Goldsmith PC, Padula CA, Roberts JL, Weiner RI. Immortalization of hypothalamic GnRH neurons by genetically targeted tumorigenesis. Neuron 1990; 5:1-10; PMID:2196069; http://dx.doi.org/10.1016/0896-6273(90)90028-E
- Mahal SP, Baker CA, Demczyk CA, Smith EW, Julius C, Weissmann C. Prion strain discrimination in cell culture: the cell panel assay. Proc Natl Acad Sci U S A 2007; 104:20908-13; PMID:18077360; http://dx.doi.org/10.1073/pnas.0710054104
- Karapetyan YE, Saa P, Mahal SP, Sferrazza GF, Sherman A, Sales N, Weissmann C, Lasmézas CI. Prion strain discrimination based on rapid in vivo amplification and analysis by the cell panel assay. PloS One 2009; 4:e5730; PMID:19478942; http://dx.doi.org/10.1371/journal.pone.0005730
- Iwamaru Y, Takenouchi T, Imamura M, Shimizu Y, Miyazawa K, Mohri S, Yokoyama T, Kitani H. Prion replication elicits cytopathic changes in differentiated neurosphere cultures. J Virol 2013; 87:8745-55; PMID:23740992; http://dx.doi.org/10.1128/JVI.00572-13
- Nishida N, Harris DA, Vilette D, Laude H, Frobert Y, Grassi J, Casanova D, Milhavet O, Lehmann S. Successful transmission of three mouse-adapted scrapie strains to murine neuroblastoma cell lines overexpressing wild-type mouse prion protein. J Virol 2000; 74:320-5; PMID:10590120; http://dx.doi.org/10.1128/JVI.74.1.320-325.2000
- Okada H, Sato Y, Sata T, Sakurai M, Endo J, Yokoyama T, Mohri S. Antigen retrieval using sodium hydroxide for prion immunohistochemistry in bovine spongiform encephalopathy and scrapie. J Comp Pathol 2011; 144:251-6; PMID:21112058; http://dx.doi.org/10.1016/j.jcpa.2010.10.001
- Shimizu Y, Kaku-Ushiki Y, Iwamaru Y, Muramoto T, Kitamoto T, Yokoyama T, Mohri S, Tagawa Y. A novel anti-prion protein monoclonal antibody and its single-chain fragment variable derivative with ability to inhibit abnormal prion protein accumulation in cultured cells. Microbiol Immunol 2010; 54:112-21; PMID:20377745; http://dx.doi.org/10.1111/j.1348-0421.2009.00190.x
- Masujin K, Shu Y, Yamakawa Y, Hagiwara K, Sata T, Matsuura Y, Imamura M, Okada H, Mohri S, Yokoyama T. Biological and biochemical characterization of L-type-like bovine spongiform encephalopathy (BSE) detected in Japanese black beef cattle. Prion 2008; 2:123-8; PMID:19158500; http://dx.doi.org/10.4161/pri.2.3.7437