Abstract
Despite intensive research into how amyloid structures can impair cellular viability, the molecular nature of these toxic species and the cellular mechanisms involved are not clearly defined and may differ from one disease to another. We systematically analyzed, in Saccharomyces cerevisiae, genes that increase the toxicity of an amyloid (M8), previously selected in yeast on the sole basis of its cellular toxicity (and consequently qualified as “artificial”). This genomic screening identified the Vps-C HOPS (homotypic vacuole fusion and protein sorting) complex as a key-player in amyloid toxicity. This finding led us to analyze further the phenotype induced by M8 expression. M8-expressing cells displayed an identical phenotype to vps mutants in terms of endocytosis, vacuolar morphology and salt sensitivity. The direct and specific interaction between M8 and lipids reinforces the role of membrane formation in toxicity due to M8. Together these findings suggest a model in which amyloid toxicity results from membrane fission.
Introduction
Amyloid proteins form a broad group of proteins that share the ability to display a particular quaternary structure: amyloid fibrils. Although unrelated in terms of their primary sequence and function, these proteins share common structural characteristics, such as fibrillar polypeptide aggregates with cross-beta protein conformations. Most studies have converged on amyloid proteins related to various diseases.Citation1 Indeed, the pathological processes associated with Parkinson, Alzheimer or prion diseases are due to the aggregation of α-synuclein (α-syn), Amyloid-β (Aβ) or Prp proteins, respectively. However, amyloids are also used for normal cellular functions.Citation2 This is the case in unicellular cells (in E. coli and S. cerevisiae) in which amyloid proteins allow the fixation of cells onto a glass surface and the formation of biofilms, but amyloid proteins may also form prions that can help the cells to survive in particular conditions.Citation3–Citation6 This is also the case in metazoans with Pmel17, which is involved in mammalian skin pigmentation.Citation7 Also, several endocrine hormones appear to be stored in secretory granules in an amyloid-like state.Citation8
Interestingly, both toxic and non-toxic amyloids share common or closely related oligomeric species. These oligomeric species are produced early during polymerization kinetics and are recognized by the same antibodies. These antibodies are directed against artificially made oligomers, synthesized by coupling an Aβ peptide to the surface of colloidal gold beads via its C-terminus. The assembly of toxic and immunoreactive species reaches a maximal level at the same time during polymerization, leading to the conclusion that these oligomeric species affect cellular viability.Citation9,Citation10 The link between the formation of toxic species and its structural requirements remains poorly understood. This is due in part to the experimental approaches used (typically based on adding large concentrations of proteins to cell cultures), as they do not represent the complexity of amyloid cellular toxicity accurately. We recently set-up a genetic screen based on generating toxic amyloids from an originally harmless amyloid.Citation11 Our model used the yeast S. cerevisiae to monitor the cellular toxicity of amyloids. Although the budding yeast does not allow modeling of complex neuronal diseases, such as Parkinson's or Alzheimer's, it does however pinpoint the role of factors involved in this process. For instance, eight of nine toxicity modifiers identified in yeast had similar effects on α-synuclein toxicity in yeast and in neuronal systems.Citation12 This model may therefore represent an attractive alternative model for studying amyloid toxicity.
The harmless amyloid peptide used in this study was the prion domain of the Het-s protein from P. anserina. The more toxic mutant (Mutant 8, M8) differs from the wild-type by ten mutations. In vivo, this mutant forms smaller dotted aggregates, which differ from the large annular wild-type (WT) aggregates. We then biochemically and biophysically characterized the structural characteristics and differences in this toxic M8 mutant.Citation13 In vitro, the fibers formed by WT and M8 amyloids differ significantly. WT amyloids exhibit typical µm-long fibers when observed through transmission electronic microscopy, whereas M8 amyloids assemble into 80 nm-long filaments. Wide Angle X-ray Scattering (WAXS) and ATR-FTIR spectroscopy confirmed that M8 is also an amyloid, but with a totally different secondary structure based on antiparallel β-sheets. However, one major question continues to persist: what makes an amyloid toxic?
Here, we have used a library of yeast gene deletion strains, known as the Euroscarf Library.Citation14 This yeast KO library has been successfully used to identify genes modulating cellular toxicity of Huntingtin with poly-glutamine (poly-Q) expansions and α-synuclein.Citation15 This collection contains 4,850 viable mutant haploid strains, each lacking a single gene. These strains were manually and individually transformed with constructs allowing conditional expression of the previously isolated toxic mutant M8. From these strains, we isolated 46 gene deletions that enhance M8 toxicity. Interestingly, the isolated genes were different from those previously identified as modulators of α-synuclein or poly-Q expressions. This set of isolated genes clearly highlights the role of traffic vesicles as a key factor in cellular toxicity. High M8 affinity for DMPC (1,2-dimyristoyl-sn-glycero-3-phosphocholine) and changes in cellular traffic due to its expression led us to suggest a model in which M8 toxicity occurs due to a greater propensity for it to be inserted into lipid layers, leading to vesicular trafficking “poisoning,” through a vesicular fission mechanism.
Results
Yeast knockout library screening reveals few toxicity enhancers.
Selecting deleted strains in which M8 toxicity was enhanced involved three steps. First, all strains from an ordered and frozen library were transformed using the microtiter plate transformation method.Citation16 This method was slightly tailored to the materials in our lab (see Materials and Methods).
Isolates of interest were those in which growth defects were specifically correlated with M8 expression. These clones were separated from false positive strains in which the growth defect may be due to the deletion itself or the transformation process. Thus, after the transformation step, we plated yeasts on dextrose medium for plasmid selection and non-selective galactose medium. The dextrose medium ensured the selection of transformed strains and prevented M8 expression. By contrast, the cell suspension grown on non-selective galactose medium was mostly populated with untransformed cells not expressing M8. The comparison between these two conditions allowed us to categorize the Euroscarf strains into three groups (Fig. S1).
Strains unable to grow on galactose medium but forming colonies on the dextrose plate. These strains were unable to grow on medium in which galactose was the sole carbon source. These strains were classified as false positives (as growth inhibition was not due to M8 but due to their incapacity to grow under the experimental conditions outlined). The 271 (Gal-) strains identified are listed in Supplementary Table 1.
Strains unable to grow on both dextrose or galactose media. These were either auxotrophic strains or strains for which the microtiter transformation protocol was ineffective. These strains were then individually re-transformed via a standard acetate lithium protocol.Citation17 Remaining non-transformable strains (auxotrophic or unable to grow on our minimal media) were not analyzed in this study. These remaining 113 strains are listed in Supplementary Table 2.
Strains able to grow on both dextrose or galactose media. These strains were analyzed and screened for M8 toxicity enhancers. At the end of this selection process, 72 strong toxicity enhancers were found for M8. One drawback to the yeast deletion library is that some strains potentially share an additional unexpected mutation.Citation18 As the same putative mutation cannot be present in a strain bearing the same KO but genetically independent from the previous library, we have checked for the toxicity phenotype in the opposite mating type (Mat a) strains obtained independently. We also tested for toxicity specificity using WT Het-s amyloid and finally isolated only 46 of the selected strains as strong M8 toxicity enhancers (Suppl. Table 3). Among these 46 strains, three also increase WT toxicity. Forty-four of these 46 mutants were completely different from those previously identified as a-synuclein and poly-glutamine toxicity enhancers,Citation15 suggesting alternative toxicity pathways for M8 toxicity. We further investigated the mechanism underlying M8 toxicity by classifying the identified genes into functional categories.
Gene ontology classification highlights the role of the vacuole.
Identified genes were classified using the FunSpec algorithm,Citation19 located at the Munich Information Center for Protein Sequences (MIPS) comprehensive yeast genome database and the Gene Ontology (GO) database. These classification methods helped us to identify possible clusters of genes involved in the same mechanism. In our case, it highlighted a group of genes involved in cellular traffic, which were strongly linked to vacuolar pathways (). Thirty percent of proteins encoded by the selected genes were localized in membranes, mitochondria and vacuoles, and were functionally involved in membrane organization and vesicle-mediated transport. Among these identified genes, three genes (PEP3, PEP5 and VPS16) belong to the class C VPS family. The Vps-C complexes contain the CORVET (class C core vacuole/endosome tethering) and HOPS (homotypic vacuole fusion and protein sorting) complexes. Both are found in endosomes and vacuoles, in which they promote membrane fusion. These complexes are built on a common Vps-C core complex formed by the products of four genes (PEP3 (VPS18), VPS16, PEP5 (VPS11 or END1) and VPS33 (SLP1)). The YPT7 gene is also involved in vacuolar function, but is not part of the Vps-C core complex. We thus characterized, in more detail, the reasons for which M8 expression is more deleterious in these mutants.
M8 selectively inhibits the growth of class C vps mutants.
Defective growth, scored by serial dilution, did not allow the identification of whether the changes occurred during the lag, exponential or stationary phases. We analyzed the toxic effects of M8 in class C vps-deleted strains more precisely; thus, we checked their growth against similar strains expressing WT amyloid or GFP alone (). BY4742 cells expressing WT or GFP reached the stationary phase 48 h after induction. Cells expressing M8 grew more slowly, but reached the same density after 96 h of induction (A). The stationary phase was reached later in pep3, pep5 and vps16 class C vps mutants (D–F) expressing M8, and the plateau was lower, indicating a higher mortality for the yeast cells.
Class C vps-deleted strains exhibited slower growth than wild type strains. Thus, their behavior in the presence of M8 may have been due to the combination of two other growth-slowing factors. We then investigated how other KO strains are affected by WT, M8 or GFP. These other KO strains (cpr7Δ and adk1Δ strainsCitation20,Citation21) were selected for their decreased rate of growth during the exponential phase. These strains have a slower growth, but reached the same plateau (stationary phase) whichever protein was expressed (WT, M8 or GFP) ( and C). Thus, toxicity enhancement observed in class C vps-deleted strains induced by M8 is specifically related to the production of M8, and does not result from other unconnected effects that slow growth. One trivial explanation for increasing M8 toxicity may be the capacity for vacuolar mutants to stabilize M8, thus leading to increasing its concentration up to a deleterious threshold level. However, western-blot analysis of M8 expressed in PEP3Δ, PEP5Δ or VPS16Δ does not sustain this hypothesis (Suppl. Fig 2). We then studied how M8 affects vacuolar protein sorting, as mutations in this cellular process increase its toxicity.
M8 interacts with lipids and affects cellular trafficking.
We investigated whether the presence of M8 results in a phenotype mimicking pep3Δ, pep5Δ and vps16Δ phenotypes. These mutants exhibit sensitivity to several metals or salts,Citation22 present some modifications of their vacuolar morphology, and display some defects in vesicular traffic via an alteration of endocytosis and cargo delivery to the vacuole.Citation23
Sensitivity to metals and salts.
We first explored the sensitivity of BY4742 cells expressing GFP, WT or M8 to LiCl, CaCl2, ZnCl2, MgCl2, MnCl2 and citric acid (). Growth was slower in all strains when plated on medium containing these molecules, but the presence of M8 did indeed increase the sensitivity of the yeast to these salts and metals. As control, we have verified that the three strains pep3Δ, pep5Δ and vps16Δ expressing GFP or WT are sensitive to these molecules (). It is difficult to measure the extent of salt sensitivity of M8 expression in BY4742 vs. deleted strains since without any chemical compounds these strains do not grew in the same way. However, the sensitivity of strains expressing M8 or bearing deletions appears to be roughly the same.
Vacuolar morphology.
We then analyzed vacuolar morphology by staining cells with lipophilic dye FM4–64. This vital fluorescent dye initially stains the plasma membrane and is then internalized and delivered to the vacuolar membrane.Citation24 Cells were incubated with FM4–64 for 10 min, washed, and subsequently incubated with FM4–64 for up to 45 min at room temperature. The presence of GFP or WT did not disturb vacuolar morphology ( and B). By contrast, several vesicles were placed side by side in M8 cells, suggesting that M8 probably triggers vacuole fragmentation ().
Lipid binding.
As amyloid toxicity is often related to the ability of these proteins to interact directly with membraneCitation25 we then analyzed the ability of M8 to directly bind to phosphatidylcholine (PC). The purified M8 peptide binds to DMPC (a representative component of PC in yeast membranes)Citation26 (). Under similar experimental conditions, WT was not found to be associated with this phospsholipid, as it was not detected on the filter with the fatty acid. This absence of detection was independent of the method used (direct observation with Ponceau red or immunodection). M8 binds to phospholipids, and thus we also investigated its ability to modify membrane traffic.
Vesicular trafficking.
We monitored endocytic traffic by measuring the transport of 5-fluoro-uracil (5-FU), a toxic analog of uracil that is imported by the permease Fur4p. In wild-type cells, Fur4p is continually removed from the plasma membrane by endocytosis. If endocytosis slows down or is blocked, Fur4p accumulates at the plasma membrane, and allows a significant amount of uracil or 5-FU into the cell, leading to its death.Citation27 We performed spotting assays by plating serial dilutions of BY4742 cells expressing GFP, WT and M8 onto two types of media: containing 5-FU or not (). After several days, whereas M8 expressing cells no longer display any toxicity phenotype as they reached the stationary plateau on a plate without 5-FU, cells producing M8 and grown on a 5-FU containing medium still display a toxicity phenotype due to their increased sensitivity to 5-FU, indicating a disturbance of endocytosis.
We then investigated the traffic between the Golgi and vacuole. Resident proteins of the vacuole are synthesized and translocated from the cytosol into the lumen of the ER or inserted into the ER membrane. They then move to the Golgi. Two pathways mediate transport between the late Golgi and the vacuole: vacuolar hydrolases, such as carboxypeptidase Y (CPY), are delivered to the vacuole via an endosome-like compartment, but alkaline phosphatases (ALP) bypass the endosome and are directly delivered to the vacuole.Citation28 The intracellular trafficking of proteins along these pathways is accompanied by post-translational modification, glycosylation and proteolytic processing.Citation29 CPY is synthesized as a precursor that is translocated into the lumen of the ER, where it undergoes signal peptide cleavage and N-linked core-glycosylation generating precursor 1CPY (p1:67 kDa). In the Golgi, CPY undergoes additional glycosylation, generating p2CPY (69 kDa). CPY is finally transported via late endosomes to the vacuole, where it is processed to give the 61 kDa mature species (mCPY).Citation29 As expected, vps mutants accumulate p2 CPY (), and this species is secreted into the medium where it is detected by colony immunoblotting (data not shown). By contrast, we detected no defect in CPY processing in M8 cells: p2CPY does not accumulate and is not secreted in the medium (). Similar results were obtained during studies of ALP processing (data not shown). Thus, M8 does not prevent the sorting of proteins and their transfer to the vacuole.
Discussion
We have previously carried out a structure-toxicity analysis of an amyloid protein that is well characterized at the biochemical level.Citation30 We aimed to isolate mutants on the basis of their toxicity and to compare their properties with those of the WT amyloid. This unbiased screen isolated a mutant containing 10 single mutations. Indeed, this mutant M8 is quite different from the WT, both in vivo and in vitro. M8 forms smaller aggregates and displays rapid polymerization, involving highly structured intermediates.Citation31 Biochemical characterization is only part of the answer, as information on toxicity results from both biochemical and cellular data. Here, we used a whole-genome approach to determine the mutations that modulate in trans the toxicity due to M8 expression. The yeast deletion library screening procedure isolated 46 KO mutants, of which two were previously shown to be involved in amyloid toxicity. One of these mutants (yol049WΔ) displays a partial loss of viability on the overproduction of exon 1 of human HD protein.Citation15 The corresponding gene encodes for a glutathione synthetase carrying out glutathione synthesis from γ-glutamylcysteine and glycine.Citation32 The corresponding GSH-deficient mutant dies rapidly if the cells are directly exposed to lethal temperatures.Citation33 Thus, this gene may have a general role in protection and may not be specific to amyloid toxicity. The yol108CΔ strain has already being identified as a modulator of α-synuclein toxicity.Citation15 This gene encodes a transcriptional activator of the ENO1 gene,Citation34 an enolase required for vacuole fusion and protein transport to the vacuole.Citation35 Interestingly, oxidative inactivation of human ENO1 (the corresponding ortholog) may lead to the development of Alzheimer disease.Citation36 The link with the vacuole was reinforced by the presence of 3 of the 4 members of the Vps-C core complex. As previously described, knocking out these genes leads to the complete absence of the vacuole.Citation23 This absence may be in part responsible for an M8 toxicity increase, through the relocation of the toxic amyloid to another compartment in which it could have a deleterious effect on the cell. This suggestion is, however, unlikely, as M8 does not appear to be specifically localized to the vacuole.Citation11 Moreover, the identification of YPT7 (a Rab-GTPase involved in binding the Vps-C HOPS complex to the vacuole membraneCitation37) as a modulator of M8 toxicity argues for a role of the Vps-C complex that is independent to the presence of the vacuole. The vacuole is highly fragmented in ypt7Δ cells, and we found a similar phenotype if M8 was expressed in yeast cells. This phenotype indicates that M8 has the capacity to interfere in the equilibrium between fusion and fission of vacuolar vesicles, but does not elicit its toxic effect through vacuolar function alone.
M8 expression failed to block the traffic between the Golgi apparatus and the vacuole, including cargo delivery to the vacuole, as carboxypeptidase Y and alkaline phosphatase are efficiently processed in the presence of M8. Interestingly, M8 impaired but did not suppress endocytosis, as FM4–64 was still internalized in yeast cells. Thus, the presence of M8 in the cell triggers some of the phenotypes observed in class C vps-deleted strains (increased sensitivity to metals or salts and impairment of endocytic traffic), but this effect was significantly attenuated. The synergetic effects between M8 and class-C vps mutants raise the possibility, in addition to their involvement in endosome-to-vacuole transport, that M8 and the class-C VPS complex play additional roles at other transport steps. This suggestion is consistent with the role of the class-C VPS complex in the processes of membrane docking and fusion at both the Golgi-to-endosome and endosome-to-vacuole stages of transport.Citation38 M8 affinity for lipids may lead directly to vesicle fission. In our model (), M8 antagonizes the fusion function of the Vps-C HOPS complex. In the absence of this complex, small vesicle formation is favored, leading to significantly greater growth impairment. The target vesicles may be vacuolar vesicles or other endocytosis vesicles. Mitochondria were excluded, as M8 did not affect mitochondrial networks and its toxicity remained the same in rho° cells (Suppl. Fig. 1). This mechanism echoes the recent finding on α-synuclein toxicity in which a genome-wide analysis has linked its toxicity in yeast to endocytosis of the protein and vacuolar fusion defects.Citation39 It may also be correlated to the disruption of endocytosis which was earlier identified as one of the causes for cellular toxicity induced by aggregated poly-glutamines.Citation40 Our model is also strengthened by a recent study based on in vitro experiments linking disruption of liposome membranes and loss of cell viability to amyloid fibril length.Citation41 Unusual M8 amyloid characteristics (short fibrils of 80 nm), together with the presence of oligomeric intermediates, are consistent with a model in which toxicity results from membrane disruption. Our findings, based on an unbiased screen to isolate a new and artificial toxic amyloid and to search for genes modulating its toxicity through trans interactions, showed that membrane fusion/fission is an essential phenomenon in amyloid toxicity and offers a powerful model for studying this phenomenon at the molecular level.
Materials and Methods
Yeast strains, media and plasmids.
Yeast strains used are isogenic to BY4742 strain (MATα, his3D1, leu2D0, ura3D0). All deletion strains were from the Euroscarf yeast deletion library [14]:
adk1Δ (MATα, his3Δ1, leu2Δ0,ura3Δ0, YDR226W::kanMX4)
cpr7Δ (MATα, his3Δ1, leu2Δ0,ura3Δ0, YJR032W::kanMX4)
pep3Δ (MATα, his3Δ1, leu2Δ0,ura3Δ0, YLR148W::kanMX4),
pep5Δ (MATα, his3Δ1,leu2Δ0, ura3Δ0, YMR231W::kanMX4)
vps16Δ (MATα,his3Δ1, leu2Δ0, ura3Δ0, YPL045W::kanMX4).
As specified, yeasts were grown in SD medium (0.67% yeast nitrogen base, 2% dextrose) or SG medium (0.67% yeast nitrogen base, 2% galactose) supplemented with 20 mg/L histidine (H), 20 mg/L lysine (K) and 60 mg/L leucine (L) or 0.67% casaminoacids. We used multicopy (2 µ) yeast expression plasmids with the URA3 selectable marker in this study: they include pYeYGFP2U (GFP), pYecHetsYGFP2U (WT), pYecHetsm8YGFP2U (M8).Citation11 The fusion of the HET-s(PrD) and yeast optimized GFPCitation42 is expressed under control of a GAL10 promoter in a pYeHFN2U.Citation43 pYEF2mtRFP allows expression of mitochondrial RFP. It is derived from pYEF1mtRFP,Citation44 which was constructed using PYX-mtGFP.Citation45 The selectable marker was changed from URA3 (pYEF1mtRFP) to LEU2 (pYEF2mtRFP).
Microtiter plate transformations.
We used the microtiter plate transformation methodCitation16 with a few modifications. We used 6 µg of carrier DNA (Ozyme) per well. To lower the cross-contamination risks during supernatant elimination, we also covered the sink used to recover the supernatant with paper. We also used a 48-well replicator to plate solutions onto standard Petri dishes. Moreover, we used pipette tips specific for viscous solutions, i.e., with a larger opening (Dutcher), particularly for solutions with PEG.
Spotting assay.
All spotting assays were performed under identical conditions. Tenfold serial dilutions starting with an equal number of cells (1OD; l = 600 nm) were prepared in sterile water. Three independent fresh transformant samples were pooled for the spotting assays. Five-microliter drops were then plated onto SD or SG medium.
Selecting clones of interest.
After a one-night recovery culture on rich dextrose solid medium (YPD), frozen yeasts from the Euroscarf library were screened using the Gietz microtiter plate transformation protocol. A two-step selection was then used to separate clones of interest from false positive candidates. The transformation mixture was plated onto both selective minimal dextrose media and non-selective minimal galactose media. The comparison between these two types of media allowed us to eliminate three false positive families: isolates unable to grow on minimal medium, isolates that did not undergo transformation and isolates that were unable to grow on minimal galactose medium. Selected transformants were then replica plated onto a selective galactose medium to induce M8 expression. Deletion mutants presenting a growth defect were then selected and processed via a serial dilution assay, to analyze their sensitivity to M8 expression more precisely. Thus, all strains remained in their exact original position during the microtiter plate transformation protocol, such that each strain could be identified by its position on the plate. Moreover, selected deletion mutants presenting a growth defect could be easily identified.
Gene ontology classification.
Genes identified during the library screen and confirmed via fresh transformation of Mat yeast strains were processed using FunSpeCitation19 for MIPS and GO classification. An additional GO classification for the function and the localization was also performed (http://db.yeastgenome.org/cgi-bin/GO/goSlimMapper.pl).
FM4–64 labeling.
1 ml of exponentially growing cells was concentrated into 100 µl, placed on ice and incubated in the presence of 20 µM FM 4–64 (Invitrogen) for 15 min. Cells were then washed twice with 1 ml of cold medium, resuspended in 1 ml of medium (t0) and incubated for 45 min at room temperature.
Microscopy.
Cells were washed in water and resuspended in medium. DNA was stained by adding 2 ng/mL Hoechst 33342 to the mounting solution. Cells were observed using a DMLB (Leica) fluorescence microscope coupled with a ColorView II (Olympus) color camera.
Protein extraction and western blotting.
Alkaline lysis extraction was used for protein extraction. Briefly, 5 OD (l = 600 nm) units of yeast cells in exponential growth were permeabilized with 500 µL of 0.185 M NaOH, 0.2% β-mercaptoethanol. After a 10-min incubation on ice, Trichloroacetic acid (TCA) was added to a final concentration of 5%, and the samples were incubated for an additional 10 min on ice. Precipitates were then collected by centrifugation at 14,000 g for 5 min. Pellets were dissolved in 30 µL of dissociation buffer (4% sodium dodecyl sulfate, 0.1 M Tris-HCl pH 6.8, 4 mM EDTA, 20% glycerol, 2% 2-mercaptoethanol and 0.02% bromophenol blue) and 15 µl of 1 M Tris-base. Yeast proteins were incubated for 5 min at 100°C and separated on a 12% SDS-PAGE. Proteins were electrically transferred onto nitrocellulose membranes (Optitran BA-S83, Schleicher & Schuell) in the presence of transfer buffer (39 mM Glycine, 48 mM Tris-base, 2% EtOH and 0.037% SDS) and were probed with monoclonal 0.5 µg/ml anti-CPY antibodies (Molecular Probes). Peroxidase-conjugated anti-mouse antibodies (Sigma) were used as secondary antibodies. Binding was detected with the SuperSignal reagent (Pierce) and the VersaDoc Imaging system (BioRad).
Protein/lipid interactions in vitro.
WT and M8 histidine-tagged proteins were produced as described previously.Citation13 Proteins in urea were desalted in 10 mM HCl pH 2.0. To start polymerisation, native proteins were prepared at concentrations of 20 µM in sterile water and pH was risen to 7.4 by adding PBS buffer (1X final). For dot blots, 15 µl of DMPC (1 mg/ml 1,2-dimyristoyl-sn-glycero-3-phosphocholine; Aventi Polar Lipids, Inc., Alabaster, AL USA) was allowed to dry on a PVDF transfer membrane (HybondTM-P, GE Healthcare Europe GmbH, Orsay, France) for 15 min. The DMPC membranes were then incubated at 37°C for 24 h with 2 ml of 20 µM WT or M8 proteins. Blots were washed twice with PBS and blocked with PBS 5% dried milk (Régilait, Saint Martin Belle Roche France). To detect the proteins bound to blotted DMPC, Ponceau red (Sigma, St. Louis, MO USA), mouse anti-His-tagged antibodies (Amersham, Piscataway, NJ, USA) and SuperSignal® West Dura Pierce chemoluminescence kits (Thermo Scientific, Perbio Science France SAS, Brebières, France) were used.
Figures and Tables
Figure 1 Viability assays for WT and KO strains. Growth of BY4742 or mutant cells expressing GFP (▴), WT (◆) or M8 (▪). Cells were grown overnight on dextrose medium supplemented with casaminoacids (0.67%). When cells reached the exponential phase, they were transferred into galactose medium supplemented with casaminoacids (0.05 OD600 nm/ml). Cell concentrations were evaluated by OD600 nm at various times after the induction over a period of 120 h.
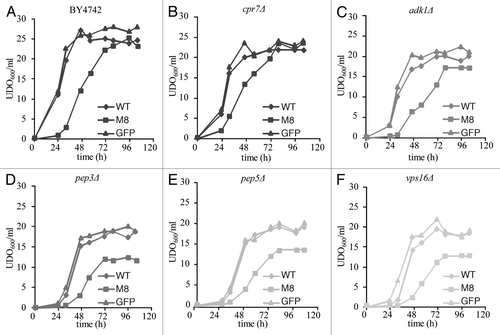
Figure 2 Metal and salt sensitivity spotting assay. Ten-fold dilutions of exponentially growing cultures of BY4742 cells expressing GFP, WT or M8 were spotted onto SD agar supplemented with casaminoacids (0.67%) and containing 8 mM MnCl2, 100 mM MgCl2, 25 mM LiCl, 100 mM CaCl2 or 10 mM citric acid (A). pep3Δ, pep5Δ and vps16Δ expressing GFP or WT were tested in the same conditions (B). The cells were incubated at 30°C for 4 days.
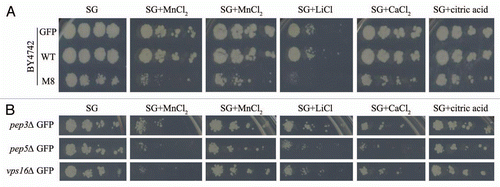
Figure 3 Vacuolar morphology observation by microscopy. Exponentially growing cultures of BY4742 cells expressing GFP, WT or M8 were incubated for 15 min on ice with the fluorescent marker FM 4–64 (20 µM). Then, the cells were washed twice and were incubated for 45 min at 30°C. Cells were mounted in medium and observed with a DMRB microscope with a 100X HCX PL fluotar objective.
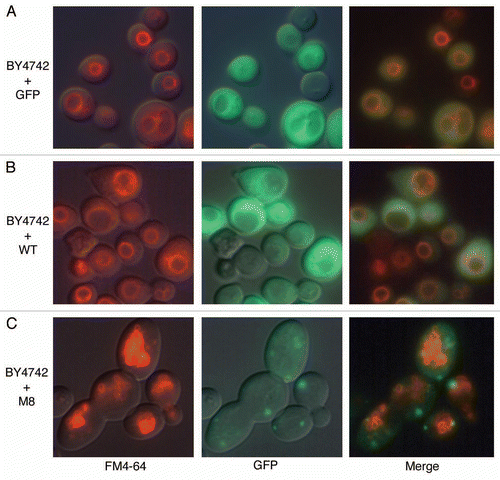
Figure 4 M8 interacts specifically with lipids in vitro. M8 interacts with DMPC blotted onto a PVDF membrane. M8 is detected directly by Ponceau red and indirectly by an anti-histidine antibody.
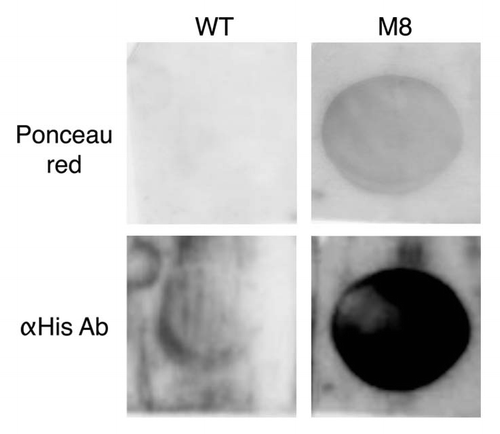
Figure 5 Traffic monitoring. (A) Ten-fold dilutions of exponentially growing cultures of BY4742 cells expressing GFP, WT or M8 were spotted onto SG agar with or without 5 µM 5-FU. Petri dishes were observed after 10 days at 30°C to allow the observation of M8 growth. (B) Exponentially growing cultures of BY4742 or mutant cells expressing GFP or M8 were used to prepare total cell extracts. The samples were run on a 12% SDS-PAGE gel, transferred onto a nitrocellulose membrane and exposed to anti-CPY antibodies. m, mature protein; p, precursor protein.
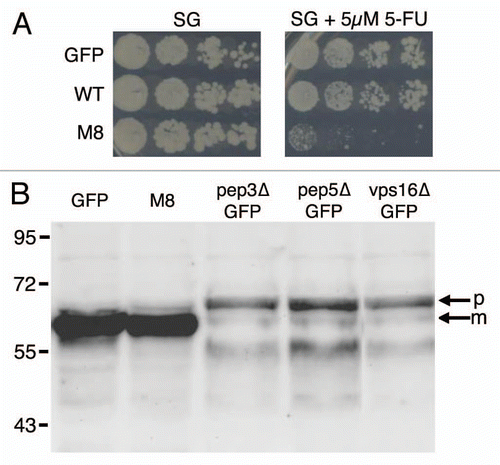
Figure 6 M8 affects vesicle trafficking. The class C HOPS complex promotes small vesicles, like early endosomes, fusion to the vacuole. M8 may prevent this fusion by coating the vacuole with an hydrophobic layer of proteins. Both M8 expression and the absence of the class C HOPS complex will affects vesicle formation leading to an accumulation of small vesicles and to a significantly greater growth impairment. Target vesicles may be vacuolar vesicles or may be other endocytosis vesicles.
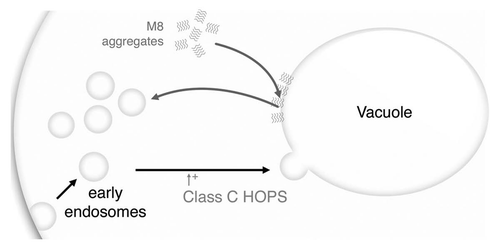
Table 1 MIPS and GO classification of verified M8 toxicity enhancers
Additional material
Download Zip (3.1 MB)Acknowledgements
We gratefully acknowledge Johanna Coindreau for her technical help and enthusiasm in searching for KO strains showing changes in M8 and WT sensitivity. This work was supported by grants from the CNRS (Programme Interdisciplinaire—Interface Physique Chimie Biologie—Soutien à la prise de Risque) and from the French Research National Agency ANR grant, project ANR-06-MRAR-011-01 «AMYLOI». K.B. received fellowships from the ANR and the Conseil Régional d'Aquitaine.
References
- Hammer ND, Wang X, McGuffie BA, Chapman MR. Amyloids: friend or foe?. J Alzheimers Dis 2008; 13:3 - 6
- Fowler DM, Koulov AV, Balch WE, Kelly JW. Functional amyloid—from bacteria to humans. Trends Biochem Sci 2007; 32:217 - 224
- Barnhart MM, Chapman MR. Curli biogenesis and function. Annu Rev Microbiol 2006; 60:131 - 147
- Cegelski L, Pinkner JS, Hammer ND, Cusumano CK, Hung CS, Chorell E, et al. Small-molecule inhibitors target Escherichia coli amyloid biogenesis and biofilm formation. Nat Chem Biol 2009; 5:913 - 919
- Sondheimer N, Lindquist S. Rnq1: an epigenetic modifier of protein function in yeast. Mol Cell 2000; 5:163 - 172
- True HL, Lindquist SL. A yeast prion provides a mechanism for genetic variation and phenotypic diversity. Nature 2000; 407:477 - 483
- Fowler DM, Koulov AV, Alory-Jost C, Marks MS, Balch WE, Kelly JW. Functional amyloid formation within mammalian tissue. PLoS Biol 2006; 4:6
- Maji SK, Perrin MH, Sawaya MR, Jessberger S, Vadodaria K, Rissman RA, et al. Functional amyloids as natural storage of peptide hormones in pituitary secretory granules. Science 2009; 325:328 - 332
- Baglioni S, Casamenti F, Bucciantini M, Luheshi LM, Taddei N, Chiti F, et al. Prefibrillar amyloid aggregates could be generic toxins in higher organisms. J Neurosci 2006; 26:8160 - 8167
- Kayed R, Head E, Thompson JL, McIntire TM, Milton SC, Cotman CW, et al. Common structure of soluble amyloid oligomers implies common mechanism of pathogenesis. Science 2003; 300:486 - 489
- Couthouis J, Rébora K, Immel F, Berthelot K, Castroviejo M, Cullin C. Screening for toxic amyloid in yeast exemplifies the role of alternative pathway responsible for cytotoxicity. PLoS ONE 2009; 4:4539
- Gitler AD, Chesi A, Geddie ML, Strathearn KE, Hamamichi S, Hill KJ, et al. Alpha-synuclein is part of a diverse and highly conserved interaction network that includes PARK9 and manganese toxicity. Nat Genet 2009; 41:308 - 315
- Berthelot K, Immel F, Géan J, Lecomte S, Oda R, Kauffmann B, et al. Driving amyloid toxicity in a yeast model by structural changes: a molecular approach. FASEB J 2009; 23:2254 - 2263
- Winzeler EA, Shoemaker DD, Astromoff A, Liang H, Anderson K, Andre B, et al. Functional characterization of the S. cerevisiae genome by gene deletion and parallel analysis. Science 1999; 285:901 - 906
- Willingham S, Outeiro TF, DeVit MJ, Lindquist SL, Muchowski PJ. Yeast genes that enhance the toxicity of a mutant huntingtin fragment or alpha-synuclein. Science 2003; 302:1769 - 1772
- Gietz RD, Schiestl RH. Microtiter plate transformation using the LiAc/SS carrier DNA/PEG method. Nat Protoc 2007; 2:5 - 8
- Gietz RD, Schiestl RH, Willems AR, Woods RA. Studies on the transformation of intact yeast cells by the LiAc/SS-DNA/PEG procedure. Yeast 1995; 11:355 - 360
- Nickerson DP, Brett CL, Merz AJ. Vps-C complexes: gatekeepers of endolysosomal traffic. Curr Opin Cell Biol 2009; 21:543 - 551
- Robinson MD, Grigull J, Mohammad N, Hughes TR. FunSpec: a web-based cluster interpreter for yeast. BMC Bioinformatics 2002; 3:35
- Duina AA, Marsh JA, Gaber RF. Identification of two CyP-40-like cyclophilins in Saccharomyces cerevisiae, one of which is required for normal growth. Yeast 1996; 12:943 - 952
- Gauthier S, Coulpier F, Jourdren L, Merle M, Beck S, Konrad M, et al. Co-regulation of yeast purine and phosphate pathways in response to adenylic nucleotide variations. Mol Microbiol 2008; 68:1583 - 1594
- Paidhungat M, Garrett S. Cdc1 and the vacuole coordinately regulate Mn2+ homeostasis in the yeast Saccharomyces cerevisiae. Genetics 1998; 148:1787 - 1798
- Rieder SE, Emr SD. A novel RING finger protein complex essential for a late step in protein transport to the yeast vacuole. Mol Biol Cell 1997; 8:2307 - 2327
- Vida TA, Emr SD. A new vital stain for visualizing vacuolar membrane dynamics and endocytosis in yeast. J Cell Biol 1995; 128:779 - 792
- Arispe N, Rojas E, Pollard HB. Alzheimer disease amyloid beta protein forms calcium channels in bilayer membranes: blockade by tromethamine and aluminum. Proc Natl Acad Sci USA 1993; 90:567 - 571
- Gaspar ML, Aregullin MA, Jesch SA, Henry SA. Inositol induces a profound alteration in the pattern and rate of synthesis and turnover of membrane lipids in Saccharomyces cerevisiae. J Biol Chem 2006; 281:22773 - 22785
- Longley DB, Harkin DP, Johnston PG. 5-fluorouracil: mechanisms of action and clinical strategies. Nat Rev Cancer 2003; 3:330 - 338
- Burd CG, Babst M, Emr SD. Novel pathways, membrane coats and PI kinase regulation in yeast lysosomal trafficking. Semin Cell Dev Biol 1998; 9:527 - 533
- Avaro S, Belgareh-Touzé N, Sibella-Argüelles C, Volland C, Haguenauer-Tsapis R. Mutants defective in secretory/vacuolar pathways in the EUROFAN collection of yeast disruptants. Yeast 2002; 19:351 - 371
- Wasmer C, Lange A, Van Melckebeke H, Siemer AB, Riek R, Meier BH. Amyloid fibrils of the HET-s (218–289) prion form a beta solenoid with a triangular hydrophobic core. Science 2008; 319:1523 - 1526
- Berthelot K, Lecomte S, Géan J, Immel F, Cullin C. A yeast toxic mutant of Het-s (218–289) prion displays alternative intermediates of amyloidogenesis. Biophys J 2010; 99:1239 - 1246
- Inoue Y, Kimura A. Identification of the structural gene for glyoxalase I from Saccharomyces cerevisiae. J Biol Chem 1996; 271:25958 - 25965
- Sugiyama K, Kawamura A, Izawa S, Inoue Y. Role of glutathione in heat-shock-induced cell death of Saccharomyces cerevisiae. Biochem J 2000; 352:71 - 78
- Chen M, Lopes JM. Multiple basic helix-loop-helix proteins regulate expression of the ENO1 gene of Saccharomyces cerevisiae. Eukaryot Cell 2007; 6:786 - 796
- Decker BL, Wickner WT. Enolase activates homotypic vacuole fusion and protein transport to the vacuole in yeast. J Biol Chem 2006; 281:14523 - 14528
- Butterfield DA, Poon HF, St Clair D, Keller JN, Pierce WM, Klein JB, et al. Redox proteomics identification of oxidatively modified hippocampal proteins in mild cognitive impairment: insights into the development of Alzheimer's disease. Neurobiol Dis 2006; 22:223 - 232
- Hickey CM, Stroupe C, Wickner W. The major role of the Rab Ypt7p in vacuole fusion is supporting HOPS membrane association. J Biol Chem 2009; 284:16118 - 16125
- Peterson MR, Emr SD. The class C Vps complex functions at multiple stages of the vacuolar transport pathway. Traffic 2001; 2:476 - 486
- Zabrocki P, Bastiaens I, Delay C, Bammens T, Ghillebert R, Pellens K, et al. Phosphorylation, lipid raft interaction and traffic of alpha-synuclein in a yeast model for Parkinson. Biochim Biophys Acta 2008; 1783:1767 - 1780
- Meriin AB, Zhang X, Miliaras NB, Kazantsev A, Chernoff YO, McCaffery JM, et al. Aggregation of expanded polyglutamine domain in yeast leads to defects in endocytosis. Mol Cell Biol 2003; 23:7554
- Xue WF, Hellewell AL, Gosal WS, Homans SW, Hewitt EW, Radford SE. Fibril fragmentation enhances amyloid cytotoxicity. J Biol Chem 2009; 284:34272 - 34282
- Taneja V, Maddelein ML, Talarek N, Saupe SJ, Liebman SW. A non-Q/N-rich prion domain of a foreign prion, [Het-s], can propagate as a prion in yeast. Mol Cell 2007; 27:67 - 77
- Cullin C, Minvielle-Sebastia L. Multipurpose vectors designed for the fast generation of N- or C-terminal epitope-tagged proteins. Yeast 1994; 10:105 - 112
- Rinaldi T, Hofmann L, Gambadoro A, Cossard R, Livnat-Levanon N, Glickman MH, et al. Dissection of the carboxyl-terminal domain of the proteasomal subunit Rpn11 in maintenance of mitochondrial structure and function. Mol Biol Cell 2008; 19:1022 - 1031
- Westermann B, Neupert W. Mitochondria-targeted green fluorescent proteins: convenient tools for the study of organelle biogenesis in Saccharomyces cerevisiae. Yeast 2000; 16:1421 - 1427