Abstract
The intricate complexity, at the molecular and cellular levels, of the processes leading to the development of amyloid proteinopathies is somehow counterbalanced by their common, universal structural basis. The later has fueled the quest for suitable model systems to study protein amyloidosis under quasi-physiological conditions in vitro and in simpler organisms in vivo. Yeast prions have provided several of such model systems, yielding invaluable insights on amyloid structure, dynamics and transmission. However, yeast prions, unlike mammalian PrP, do not elicit any proteinopathy. We have recently reported that engineering RepA-WH1, a bacterial DNA-toggled protein conformational switch (dWH1 → mWH1) sharing some analogies with nucleic acid-promoted PrPC → PrPSc replication, enables control on protein amyloidogenesis in vitro. Furthermore, RepA-WH1 gives way to a non-infectious, vertically-transmissible (from mother to daughter cells) amyloid proteinopathy in Escherichia coli. RepA-WH1 amyloid aggregates efficiently promote aging in bacteria, which exhibit a drastic lengthening in generation time, a limited number of division cycles and reduced fitness. The RepA-WH1 prionoid opens a direct means to untangle the general pathway(s) for protein amyloidosis in a host with reduced genome and proteome.
The development of suitable model systems for the study of the complex neurodegenerative and systemic human diseases caused by the aggregation of proteins into amyloid cross-β assemblies has been successfully attempted in different ways.Citation1 From the point of view of the macromolecules involved, besides those proteins directly involved in amyloid diseases (Alzheimer's β-amyloid and Tau, Creutzfeldt-Jakob's PrP, Parkinson's α-synuclein, Huntington's huntingtin or β2-microglobulin in dialysis-related amyloidosis), an ever increasing number of disease-unrelated proteins can be forced to unfold, and subsequently assemble, as amyloids under extreme, non-physiological physicochemical conditions. Both kinds of model proteins have been crucial to establish our current understanding of the common molecular basis for protein amyloidogenesis.Citation1 At the organisms side, although animal models, most notably mice, have returned invaluable information on protein amyloidosis, the complexity of the intricate regulatory and biochemical networks inherent to metazoans and their cultured cells, has hampered the outlining of a clear scenario on the mechanism(s) leading to cytotoxicity. Cytotoxicity can arise either from properties common to most amyloidogenic proteins, such as targeting of cell membranes by amyloid oligomers or co-aggregation of essential cell factors, or through pathways particular to each protein and its associated disease.Citation2 The key to such a riddle relies on comprehensive systems biology analyses, but also on resorting to experimental models with their number of potential variables (proteins and their interactions) drastically reduced, but yet showing the same (cytotoxic) response.
Since the discovery of the Ure2p/[URE3+] and Sup35p/[PSI+] prions in yeast, these (relatively) simple eukaryotic microorganisms have been instrumental in addressing the molecular basis for amyloid conformational templating, structural polymorphism and cell-to-cell transmissibility.Citation3–Citation5 However, two limitations to the applicability of yeast prions as universal models for amyloidosis are noteworthy: (1) the amyloidogenic sequence stretches in yeast prions are consistently Gln/Asn-rich, unlike most proteins involved in amyloid proteinopathies (which bear hydrophobic stretches) with the exception of the proteins involved in Huntington disease and in related ataxias; (2) even more importantly, while yeast prions are the epigenetic determinants of distinct, mildly advantageous phenotypes that improve adaptability to environmental challenges,Citation6,Citation7 they are not the causative agents of a proteinopathy in yeast albeit, when overexpressed, Sup35p/[PSI+] becomes detrimental for cell growth. Although this prion has recently been successfully propagated in Escherichia coli,Citation8 it still does not behave as a proper pathogenic agent in this microorganism. Natural amyloids have also been described and characterized in bacteria such as E. coli (curli/CsgA)Citation9 and Pseudomonas (FapC),Citation10 but, invariantly, they are extracellularly secreted and functional in scaffolding cellular consortia such as biofilms. A case apart is posed by inclusion bodies, intracellular protein aggregates accumulated in bacterial cytoplasm upon heterologous expression of recombinant proteins, which exhibit some amyloid featuresCitation11 but with a discrete detrimental effect on cell fitness.Citation12,Citation13
RepA-WH1: A DNA-Promoted Conformational Switch Enabling Amyloidosis
Along several years, in our laboratory we had worked out the mechanism responsible for the conformational activation of dimers of the Pseudomonas (plasmid pPS10) protein RepA, which act as transcriptional repressors of the repA gene, into DNA replication initiation-competent monomers.Citation14 RepA recognizes a conserved 8 bp DNA sequence in two alternative orientations: either an inverted repeat (operator, bound by RepA dimers) or direct repeats in tandem (iterons, bound by RepA monomers). RepA is made of two winged-helix (WH) domains, acting the N-terminal domain (RepA-WH1) as a dimerization module and the C-terminal domain (RepA-WH2) as the main DNA binding interface.Citation15 Allosteric binding of the origin of replication-specific dsDNA sequence to the RepA dimers exerts a conformational change that, besides dissociating the protein in its constituent monomers, refolds two α-helical stretches in RepA-WH1 as β-strands and loops, enabling both WH1 and WH2 to bind to the iteron sequence.Citation16–Citation18 By binding to the iteron repeats, RepA monomers build a nucleoprotein complex that initiates plasmid replication, de-stabilizing the DNA double helix and attracting to the origin the required host replication factors (helicase, primase, DNA polymerase). Once a full replication round is completed, the WH1 domain in RepA monomers is involved in negative regulation of DNA replication, to keep plasmid copy-number within control: interactions between WH1 domains in RepA monomers bound to two distinct, recently replicated plasmid molecules build the axial core of a nucleoprotein filament which curb new rounds of premature origin firing.Citation19
It did not escape our attention that quite a few analogies between RepA-WH1 and the mammalian prion protein (PrP) could be traced. Being structurally non-homologous, albeit topologically both classify as α + β protein folds, PrP C-terminal globular domain and RepA-WH1 undergo to a similar extent α-helix to β-strand/coil transitions upon binding to nucleic acids, RNA in the case of the mammalian proteinCitation20 and dsDNA in the bacterial one (for structural details on RepA-WH1, see ), which result in protein aggregation. Therefore we decided to test whether the RepA-WH1 aggregates would also be amyloid in nature. According to predictions made in silico, a peptide in WH1 (L26VLCAASLI34) would have a high potential to aggregate as β-strands.Citation21 However, it was located at the C-terminus of an α-helix (α2) in the crystal structure of dimeric RepA-WH1,Citation17 partially buried in the hydrophobic core of the domain but becoming exposed to the solvent in the monomeric species.Citation14,Citation16 The score for the aggregation prediction was highest in the case of a single point mutation (A31V) in that sequence, which was recurrently isolated in blind genetic searches for RepA variants exhibiting more efficient interactions with other proteins of the replication machinery of the cell.Citation14 A synthetic peptide spanning the sequence with the A31V mutation assembled into fibrils (visualized by TEM), increasing by 3-fold its β-sheet contents (according to CD spectroscopy). Fibrils were shown, by X-ray powder diffraction, to exhibit the characteristic amyloid pattern.Citation21 The whole WH1(A31V) domain formed amyloid (Congo red-reactive) fibers in vitro (), with a defined width (25 nm) and apparently composed of two twisted protofilaments, only if the dsDNA sequence (5′-CATTGACTTGT/5′-ACA AGTCAATG) spacing the inverted repeats at the repA operator was included in the assay.Citation21 The WH1-DNA complexes were transient, with the nucleic acid having a catalytic role in promoting amyloidogenesis. The wild-type WH1 domain was also able to assemble into amyloids (Congo red stainable, β-sheet enriched), but as nano-spheres, not as fibers.Citation21 Since the amyloidogenic stretch in WH1 is encompassed by the region mapped to be involved in building the nucleoprotein complex responsible for negative control of plasmid replication,Citation19 it is tempting to propose that an amyloid cross-β could constitute the axial core of such a complex, but this remains to be experimentally tested.
In a subsequent study,Citation22 we found that a symmetrically substituted tetra-sulphonated derivative of the indigo stain was able to inhibit dsDNA-promoted amyloidogenesis of RepA-WH1, by binding with reasonable affinity (ITC assays) at the DNA recognition interface in the protein dimers (involving electrostatic interactions with the Arg residues 78, 81, 91 and 93; ). This was, to our knowledge, one of the earliest examples of inhibition of protein amyloidosis by interfering with the binding of an allosteric effector (DNA), rather than through directly targeting the assembly of an amyloidogenic peptide stretch in a cross-β sheet. We proposed a kinetically-driven scenario for dsDNA-promoted RepA-WH1 amyloidogenesis, in which the extent of the conformational change exerted by any given dsDNA sequence would be inversely correlated with the order achieved upon assembly: a quick, efficient α-to-β transformation (iteron as effector) would result in amorphous aggregation, whereas slower accretion (operator spacer as effector) would lead to a more ordered deposition, thus building-up well-defined fibers.
Cytotoxic RepA-WH1 Amyloids Assembled and Propagated in E. coli
Initially, the interest of the aforementioned parallelism between RepA-WH1 and PrP amyloids was merely formal, because, besides them, none of the other proteins for which amyloidogenesis had been achieved did require nucleic acids as co-factors in templated replication in vitro,Citation23 a feature also fulfilled later by α-synuclein.Citation24 We decided to go a step further by performing in vivo studies, surveying the effects of the expression in E. coli of diverse RepA-WH1 constructs fused to a monomeric red (mCherry) variant of GFP. Our findings, as reported in a recent publication,Citation25 can be summarized as follows ():
WH1(WT) expression leads to a pattern of diffused fluorescence across the cytoplasm, whereas WH1(A31V) results in fluorescent foci (aggregated protein particles) with various numbers (1–6, with mode in 2) and spatial distributions (predominantly at 1/4–3/4 along the main cell axis, although single subpolar or central, or even side-by-side paired foci, are also found) ().
An amyloid-specific fluorescent label (BTA-1) stains the aggregated inclusions, thus confirming their amyloid nature.
The presence of multiple, plasmid-borne extra copies (besides those intrinsically found in the bacterial chromosome) of an amyloidogenic DNA effector sequence increases significantly the size of the inclusions and the frequency of cells carrying them. This points to a role of DNA as promoter of RepA-WH1 amyloidogenesis in vivo, as previously found in vitro.Citation21,Citation22
The locations of the bacterial nucleoid and the foci are mutually exclusive, pointing to restrictions to free diffusion, which arise from the large size of the protein aggregates, as the entropic factor driving their spatial organization in the bacterial cytoplasm.Citation26 Time-lapse microscopy shows that, before septation in cytokinesis, small aggregated particles become evident and are easily transmitted to the progeny. Notwithstanding, the partition of such inclusions between daughter cells is often asymmetric and seems governed by stochasticity.
Compared with cells not expressing the hyper-amyloidogenic WH1(A31V) protein, or expressing the WH1(WT) species, amyloid-carrying cells expand their generation times by five-fold and, after a few division cycles, stop growing. Failure in transmitting a seed to a daughter cell results in amyloidosis cured from its progeny. The population of such aggregate-free cells, due to their superior fitness, quickly overtakes the cultures.
The aforementioned heterogeneities in the intracellular distribution and number of the RepA-WH1 amyloid aggregates, their seed-mediated propagation and acute cytotoxicity clearly put them aside from conventional bacterial inclusion bodies.
Amyloid inclusions purified from bacteria (ex vivo) are able to seed the growth of amyloid fibers in vitro from soluble WH1(A31V) in the absence of any supply of dsDNA (). This finding shows that once DNA has forged the amyloidogenic conformational change in WH1 inside the cells, this is preserved to template amyloidosis on further molecules of the protein. Such molecular transmissibility is a key feature in any amyloid proteinopathy.Citation27
Based on the evidences discussed above, RepA-WH1 protein aggregates constitute the first intracellular, vertically transmissible, amyloid proteinopathy ever described in prokaryotes, thus allowing the study of amyloidosis in an organism with barely 3,000 genes/proteins and thus with a reduced interactome.
Conclusions and Further Perspectives
RepA-WH1 therefore fulfills the recent definition of a prionoid, applied to any molecularly (in vitro) and vertically (coupled to cell division in vivo) transmissible amyloid protein particle failing to show microbiological infectivity (horizontal transmission).Citation27 In these terms, RepA-WH1 is thus clearly distinct to the PrP prion, albeit both share nucleic acid-promoted amyloidogenesis, but closer to β-amyloid, α-synuclein, huntingtin and Tau. However, because horizontal (cell-to-cell) transmission has been recently reported for some prionoids,Citation28 testing whether this could also be the case for RepA-WH1, even under contrived experimental conditions, is a matter of utmost interest. In order to become broadly regarded as a simple, yet valuable model system in the assault on these human proteinopathies, the bacterial RepA-WH1 prionoid still needs further characterization to fully address, among other issues, the structural dynamics, thermodynamics and kinetics of dsDNA-promoted RepA-WH1 amyloidogenesis, the role of molecular chaperones, macromolecular crowding and stochasticity in the spread of amyloidosis, and the molecular pathways leading to cytotoxicity and aging in bacteria. Amyloid aggregation of the RNA binding protein CPEB in Aplysia neurons has been recently proposed as a molecular mechanism likely involved in long-term potentiation of synapses (memory).Citation29 If nucleic acids were involved in CPEB amyloidogenesis, any knowledge derived from studies on their efficient role as effectors in RepA-WH1 amyloidosis would be of great additional value. Besides these biomedical goals, the DNA-modulated RepA-WH1 conformational switch opens new ways to a reliable and controlled use of amyloids in nanotechnology and synthetic biology.Citation30
Figures and Tables
Figure 1 The bacterial RepA-WH1 prionoid highlighted at the molecular and cellular levels. The stable, soluble dimers of the isolated RepA-WH1 domain (dWH1, 1HKQ.pdb) undergo a conformational transformation upon transient, low affinity (albeit sequence-specific) binding to dsDNA ligand, thus resulting in metastable, aggregation-prone monomers (mWH1, modeled on 1REP.pdb). The roughly invariant (rms deviation for 76 Cα atoms: 1.34 Å) WH fold is depicted in cyan, whereas segments showing a significant structural shift are shown in blue. The amyloidogenic peptide L26VLCAVSLI34 (C-terminal 2/3 of α2),Citation21 is colored in red, with the side-chain of the hyper-amyloidogenic mutant residue V31 (bold) rendered as CPK spheres. A possible pathway for the exposure of the amyloidogenic stretch is sketched (dashed grey arrows 1–3): (1) DNA binding to dWH1 results in a bend in the β-hairpin (β2–β3),Citation17 which carries the sensor Arg residues (R78, R81, R91 and R93; yellow),Citation22 thus disrupting the dimerization interface (second dWH1 subunit in grey). (2) Simultaneously, β2–β3 pushes the N-terminal α1 that swingsCitation16 and partially unfolds. (3) The opening of such α1 latch releases C-terminal α5 which, in the whole mRepA, will then pack with the N-terminus of the WH2 domain to build an antiparallel β-sheet,Citation17 but in the isolated mWH1 is unfolded (dashed coil) to leave the amyloidogenic stretch largely exposed to the solvent and ready to refold and assemble into an amyloid cross-β sheet.Citation21 Models rendered with PyMOL (www.pymol.org).
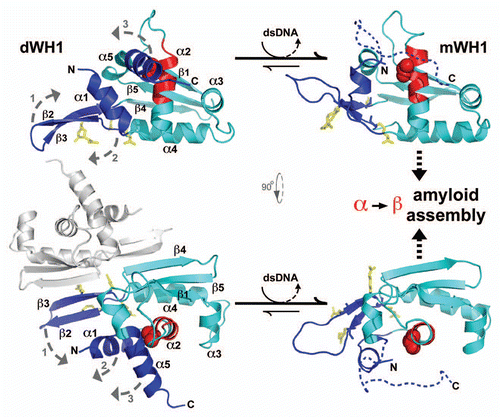
Figure 2 Linking propagation of the bacterial RepA-WH1 prionoid in vitro and in vivo. (A) Electron micrograph of amyloid fibers assembled in vitro by RepA-WH1(A31V) in the presence of effector dsDNA molecules.Citation21,Citation22 Magnification bar: 0.1 µm. (B) E. coli K-12 expressing mCherry-tagged RepA-WH1(A31V) accumulate multiple red fluorescent, aggregated amyloid foci which severely hamper cell proliferation.Citation25 Magnification bar: 1 µm. (C) Molecular transmissibility, the ability of purified bacterial amyloid inclusions/seeds (arrows) to template the transformation and assembly into fibers of soluble RepA-WH1(A31V) molecules,Citation25 links together amyloidosis in vitro and in vivo. Magnification bar: 0.1 µm.
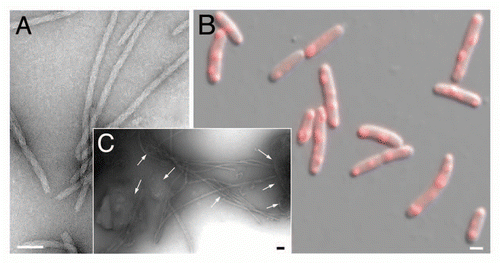
Acknowledgments
We apologize to the colleagues whose relevant work we have not quoted due to strict space limitations. Research in our laboratory on RepA-WH1 amyloids is currently financed by Spanish MICINN grants BIO2009-06952 and CSD2009-00088.
References
- Chiti F, Dobson CM. Protein misfolding, functional amyloid and human disease. Annu Rev Biochem 2006; 75:333 - 366
- Olzscha H, Schermann SM, Woerner AC, Pinkert S, Hecht MH, Tartaglia GG, et al. Amyloid-like aggregates sequester numerous metastable proteins with essential cellular functions. Cell 2011; 144:67 - 78
- Wickner RB, Edskes HK, Shewmaker F, Nakayashiki T. Prions of fungi: Inherited structures and biological roles. Nat Rev Microbiol 2007; 5:611 - 618
- Tessier PM, Lindquist S. Unraveling infectious structures, strain variants and species barriers for the yeast prion [PSI+]. Nat Struct Mol Biol 2009; 16:598 - 605
- Greenwald J, Riek R. Biology of amyloid: Structure, function and regulation. Structure 2010; 18:1244 - 1260
- Tuite MF, Serio TR. The prion hypothesis: From biological anomaly to basic regulatory mechanism. Nat Rev Mol Cell Biol 2010; 11:823 - 833
- Halfmann R, Lindquist S. Epigenetics in the extreme: Prions and the inheritance of environmentally acquired traits. Science 2010; 330:629 - 632
- Garrity SJ, Sivanathan V, Dong J, Lindquist S, Hochschild A. Conversion of a yeast prion to an infectious form in bacteria. Proc Natl Acad Sci USA 2010; 107:10596 - 10601
- Wang X, Zhou Y, Ren JJ, Hammer ND, Chapman MR. Gatekeeper residues in the major curlin subunit modulate bacterial amyloid fiber biogenesis. Proc Natl Acad Sci USA 2010; 107:163 - 168
- Dueholm MS, Petersen SV, Sønderkaer M, Larsen P, Christiansen G, Hein KL, et al. Functional amyloid in Pseudomonas. Mol Microbiol 2010; 77:1009 - 1020
- DeGroot NS, Sabaté R, Ventura S. Amyloids in bacterial inclusion bodies. Trends Biochem Sci 2009; 34:408 - 416
- Lindner AB, Madden R, Demarez A, Stewart EJ, Taddei F. Asymmetric segregation of protein aggregates is associated with cellular aging and rejuvenation. Proc Natl Acad Sci USA 2008; 105:3076 - 3081
- Winkler J, Seybert A, König L, Pruggnaller S, Haselmann U, Sourjik V, et al. Quantitative and spatio-temporal features of protein aggregation in Escherichia coli and consequences on protein quality control and cellular ageing. EMBO J 2010; 29:910 - 923
- Giraldo R, Fernández-Tresguerres ME. Twenty years of the pPS10 replicon: Insights on the molecular mechanism for the activation of DNA replication in iteron-containing bacterial plasmids. Plasmid 2004; 52:69 - 83
- Giraldo R, Andreu JM, Díaz-Orejas R. Protein domains and conformational changes in the activation of RepA, a DNA replication initiator. EMBO J 1998; 17:4511 - 4526
- Díaz-López T, Lages-Gonzalo M, Serrano-López A, Alfonso C, Rivas G, Díaz-Orejas R, et al. Structural changes in RepA, a plasmid replication initiator, upon binding to origin DNA. J Biol Chem 2003; 278:18606 - 18616
- Giraldo R, Fernández-Tornero C, Evans PR, Díaz-Orejas R, Romero A. A conformational switch between transcriptional repression and replication initiation in the RepA dimerization domain. Nat Struct Biol 2003; 10:565 - 571
- Díaz-López T, Dávila-Fajardo C, Blaesing F, Lillo MP, Giraldo R. Early events in the binding of the pPS10 replication protein RepA to single iteron and operator DNA sequences. J Mol Biol 2006; 364:909 - 920
- Gasset-Rosa F, Díaz-López T, Lurz R, Prieto A, Fernández-Tresguerres ME, Giraldo R. Negative regulation of pPS10 plasmid replication: Origin pairing by zipping-up DNA-bound RepA monomers. Mol Microbiol 2008; 68:560 - 572
- Silva JL, Lima LM, Foguel D, Cordeiro Y. Intriguing nucleic-acid-binding features of mammalian prion protein. Trends Biochem Sci 2008; 33:132 - 140
- Giraldo R. Defined DNA sequences promote the assembly of a bacterial protein into distinct amyloid nanostructures. Proc Natl Acad Sci USA 2007; 104:17388 - 17393
- Gasset-Rosa F, Maté MJ, Dávila-Fajardo C, Bravo J, Giraldo R. Binding of sulphonated indigo derivatives to RepA-WH1 inhibits DNA-induced protein amyloidogenesis. Nucl Acids Res 2008; 36:2249 - 2256
- Deleault NR, Harris BT, Rees JR, Supattapone S. Formation of native prions from minimal components in vitro. Proc Natl Acad Sci USA 2007; 104:9741 - 9746
- Hegde ML, Vasudevaraju P, Rao KJ. DNA induced folding/fibrillation of alpha-synuclein: New insights in Parkinson's disease. Front Biosci 2010; 15:418 - 436
- Fernández-Tresguerres ME, Moreno-Díaz de la Espina S, Gasset-Rosa F, Giraldo R. A DNA-promoted amyloid proteinopathy in Escherichia coli. Mol Microbiol 2010; 77:1456 - 1469
- Jun S, Wright A. Entropy as the driver of chromosome segregation. Nat Rev Microbiol 2010; 8:600 - 607
- Aguzzi A. Beyond the prion principle. Nature 2009; 459:924 - 925
- Brundin P, Melki R, Kopito R. Prion-like transmission of protein aggregates in neurodegenerative diseases. Nat Rev Mol Cell Biol 2010; 11:301 - 307
- Si K, Choi YB, White-Grindley E, Majumdar A, Kandel ER. Aplysia CPEB can form prion-like multimers in sensory neurons that contribute to long-term facilitation. Cell 2010; 140:421 - 435
- Giraldo R. Amyloid assemblies: Protein legos at a crossroads in bottom-up synthetic biology. Chem Bio Chem 2010; 11:2247 - 2257