Abstract
Yeast prions are heritable protein-based genetic elements which rely on molecular chaperone proteins for stable transmission to cell progeny. Within the past few years, five new prions have been validated and 18 additional putative prions identified in Saccharomyces cerevisiae. The exploration of the physical and biological properties of these “nouveau prions” has begun to reveal the extent of prion diversity in yeast. We recently reported that one such prion, [SWI+], differs from the best studied, archetypal prion [PSI+] in several significant ways.1 Notably, [SWI+] is highly sensitive to alterations in Hsp70 system chaperone activity and is lost upon growth at elevated temperatures. In that report we briefly noted a correlation amongst prions regarding amino acid composition, seed number and sensitivity to the activity of the Hsp70 chaperone system. Here we extend that analysis and put forth the idea that [SWI+] may be representative of a class of asparagine-rich yeast prions which also includes [URE3], [MOT3+] and [ISP+], distinct from the glutamine-rich prions such as [PSI+] and [RNQ+]. While much work remains, it is apparent that our understanding of the extent of the diversity of prion characteristics is in its infancy.
Yeast prions are heritable elements, most of which are amyloid aggregates of single proteins. The three best studied yeast prions [PSI+], [RNQ+] (also called [PIN+]), and [URE3] are formed from amyloid aggregates of the cytosolic yeast proteins Sup35, Rnq1 and Ure2, respectively.Citation2 Yeast prions can spontaneously arise in an otherwise clonal cell population, a process referred to as prion formation or nucleation, but once formed their continued propagation is intimately related to molecular chaperone activity. Chaperone function is needed to fragment prion amyloids to create heritable seeds which can then be passed on to cell progeny, thus maintaining the prion in the cell line.Citation3 Yeast prions vary in the steady-state number of heritable seeds per cell; having more seeds increases the chances of passing the prion to progeny and hence prions with higher seed numbers are more mitotically stable.Citation4–Citation6
The currently accepted model of prion fragmentation posits that components of the Hsp70 chaperone system work in congress with the disaggregase Hsp104.Citation1,Citation7–Citation9 Hsp70-type chaperones function by repeatedly binding and releasing client polypeptides in an ATP-dependent manner, a cycle that is tightly regulated by co-chaperone proteins (). J-proteins (Hsp40s) stimulate Hsp70 ATP hydrolysis and peptide binding via a conserved J-domain whereas nucleotide exchange factors (NEFs) stimulate ADP/ATP exchange, restoring the ATP-bound (peptide unbound) state. In prion fragmentation, the J-protein Sis1, the Hsp70 Ssa, and nucleotide exchange factors (NEFs) of the Sse family are co-chaperones required as partners for the Hsp70 Ssa. While chaperone proteins may have additional functions in prion biology, e.g., prion formation, these additional functions are still poorly understood.Citation9
In the past few years, the number of known yeast prions has rapidly grown such that, to date, a total of eight yeast prions have been identified and an additional 18 proteins have been annotated as putative prions.Citation10 The biological and physical properties of these newly discovered prions are only beginning to be explored. We recently reported the results of an investigation into the biological properties of the prion [SWI+], which is formed from the chromatin-remodeling factor Swi1.Citation1 Swi1 is part of the SWI/SNF chromatin-remodeling complex that regulates the expression of approximately 6% of all yeast genes.Citation11 The presence of [SWI+] causes partial loss of SWI/SNF chromatin-remodeling function, resulting in the impaired ability to uptake certain sugars, among other phenotypes.Citation11 [SWI+] is a prion of particular interest because of its potential to alter global gene expression. Below we describe its intriguing interactions with molecular chaperone proteins and environmental stress, and the implications of these properties on yeast prion biology.
Alterations in Hsp70 System Activity and Exposure to Environmental Stress can Cure [SWI+]
[PSI+], [RNQ+] and [URE3],Citation7,Citation12 as well as [SWI+],Citation1 require the activity of the J-protein Sis1 for continued propagation. However, [SWI+], unlike the other three prions, is also reliant upon the activity of another J-protein Ydj1.Citation1 In addition to being sensitive to J-protein depletion, some prions are also sensitive to J-protein overexpression. For example, [URE3] is highly sensitive to the overexpression of several J-proteins, whereas other prions, such as [PSI+] and [RNQ+], are relatively insensitive to such alterations. [SWI+] was more similar to [URE3] in this regard, being destabilized by overexpression of either Ydj1 or Sis1, as well as a third related J-protein Apj1. Given the variety of J-proteins capable of destabilizing [SWI+], we reasoned that [SWI+] may be sensitive to J-domain function generically (i.e., Ssa ATPase stimulation). Indeed, overexpression of only a J-domain linked to a hemagglutinin tag was sufficient to cure [SWI+].
Interestingly, [SWI+] was not only sensitive to alterations in J-protein expression, but also NEF expression, being destabilized by either overexpression or depletion of the NEF Sse1.Citation1 These results are revealing in that [SWI+] was profoundly destabilized by alterations that change the Hsp70 cycle in any of four possible ways: (1) reduction of ATPase stimulation (J-protein depletion), (2) enhancement of ATPase stimulation (J-protein/domain overexpression), (3) reduction of ATP/ADP exchange (NEF depletion), (4) enhancement of ATP/ADP exchange (NEF overexpression). Further, J-protein (or J-domain) overexpression or Sse deletion should favor the accumulation of the Ssa•ADP state, which is likely to be associated with prion proteins, whereas J-protein depletion or Sse overexpression should favor the Ssa•ATP state, which only transiently binds client proteins ( and ). [SWI+], then, appears to be finely tuned, either to the relative steady state levels of these two forms, or to the overall rate of Hsp70 cycling, since any alteration can potentially alter the rate of cycling. We should note, in addition, that Ssa itself is difficult to overexpress significantly in yeast due to the presence of multiple compensatory isoforms (Ssa1-4) and tight regulatory mechanisms. Despite this impediment, we found that dominant expression of an Ssa variant known to weakly destabilize [PSI+],Citation1,Citation13 caused complete curing of [SWI+] as well, underscoring the acute sensitivity of the prion towards alterations in Hsp70 activity.
The high sensitivity of [SWI+] to changes in chaperone activity raised the question of whether [SWI+] might be more sensitive than other prions to certain stress conditions known to alter chaperone levels. While it is well known that some of the well-studied prions can be mildly destabilized by certain natural stress conditions,Citation14–Citation16 prion loss is typically incomplete, indicating that these prions are generally stable under most stress conditions. We found no evidence for [SWI+] loss in response to acute stresses like heat-, ethanol- or oxidative-shock.Citation1 However, because prion curing typically requires cell growth, we wondered whether these conditions simply failed to alter chaperone levels for a long enough period of time to allow for prion curing. Indeed, shifting cells to elevated temperatures (37°C) for several days resulted in massive [SWI+] loss, indicating that [SWI+] is sensitive to sustained stress conditions. None of the other well-studied prions discussed here are destabilized by growth at 37°C. To the best of our knowledge, this experiment is the most striking example of prion curing by an environmentally relevant growth condition. Recently Halfmann et al. described yeast prion epigenetics as a “life cycle” between prion formation and curing, both potentially stress induced.Citation10 Our finding of temperature-induced [SWI+] curing currently stands as the most distinct example of the latter process. Further exploration of the stability of [SWI+] under other environmental conditions will delineate the variety of stress conditions to which this prion is susceptible.
In sum, since [SWI+] was previously shown to rely upon the activity of the disaggregating ATPase Hsp104 for propagation,Citation11 it was not surprising to find that the other core components of the prion-chaperone machinery (Sis1, Ssa, Sse) were also required for [SWI+] propagation, and thus strengthening the argument that this machinery may be universally required among amyloid-forming yeast prions.Citation1 However the degree to which [SWI+] appears to rely upon moderate Hsp70 cycling is striking and currently unprecedented among the prions studied thus far. These results imply that [SWI+] serves as an example of a meta-stable element, sitting precariously on a razor's edge of precisely balanced chaperone activities.
Correlations Among Yeast Prions
In order to understand the significance of the high sensitivity of [SWI+] to chaperones, we compared our results with those previously obtained for other prions (). In addition to [PSI+], [RNQ+], [URE3] and [SWI+], we included in our analysis the hybrid prion, [PSI+PS], which is formed from a chimera of Sup35 of S. cerevisiae and Pichia methanolytica.Citation17–Citation19 One possible confounding factor in making such comparisons is the influence of yeast strain background. For example, [SWI+] has only been analyzed in the 74D-694 background,Citation1 while [URE3] has been studied in W303.Citation7 However, we recently compared [PSI+] and [RNQ+] in these two backgrounds with similar results.Citation1,Citation7 Thus, we can vicariously, though not directly, compare [SWI+] and [URE3]. When compared to other prions, it is evident that, apart from Sis1 repression, both [RNQ+] and [PSI+] are inherently stable when other facets of the Hsp70 system chaperone are altered. In sharp contrast, [SWI+], [URE3] and the hybrid prion [PSI+PS] are far more sensitive to such alterations.Citation1,Citation19
What structural features might underlie the observed differences in chaperone sensitivity? One plausible answer is amino acid composition. The amino acid composition of the putative Prion-forming Domains (PrDs) of Ure2 and Swi1 are dissimilar from those of Sup35 and Rnq1, as recently pointed out by Crow and Li and reaffirmed in our recent study.Citation1,Citation20 The PrDs of Sup35 and Rnq1 are enriched in glutamine (Q) compared to asparagine (N), rich in glycine, and sparse in certain large hydrophobic residues (F, W, L, I, V, & M), compared to other prions (). In contrast, the putative PrDs of Swi1, Ure2, Mot3 and Sfp1 (which form the prions [SWI+], [URE3], [MOT3+] and [ISP+], respectively) are all N-rich, poor in glycine and more plentiful in large hydrophobic residues, all characteristics which have been found to favor amyloid formation.Citation21–Citation24 If indeed these N-rich prion proteins are more highly amyloidogenic, this property could affect sensitivity to chaperones in several ways. Hyper-amyloidogenic prions might have higher nucleation or fiber extension rates, altered ability to laterally associate, or could simply have a more resilient amyloid core making them less frangible. This last hypothesis is the simplest, and would explain the reduced seed numbers associated with [SWI+] and [URE3] relative to [RNQ+] and [PSI+] ().Citation1,Citation7 Heightened chaperone sensitivity may simply be a consequence of having a lower number of heritable seeds per cell with which to buffer against temporary changes in prion fragmentation rates brought on by altered chaperone expression.
Might prions with more amyloidogenic PrDs be expected to appear more frequently in a cell population? The uninduced rate of [PSI+] formation, that is, the rate uninfluenced by protein overexpression or environmental stresses, was recently reported to be 5.7 × 10−7/cell/generation.Citation25 In contrast, some, if not all, of the N-rich prions discussed above form at much higher rates (). For example, using the same assay that was used to calculate [PSI+] formation, Volkov et al. determined the formation rate of [ISP+] to be 1 × 10−4/cell/generation.Citation26,Citation27 Likewise, the formation rate for [MOT3+] has been reported to be 1 × 10−4.Citation24 The rates of [URE3] and [SWI+] formation are less clear. Uninduced [URE3] formation rates as high as 1 × 10−4 have been reported, but generally vary between 1 × 10−6 and 1 × 10−5, with specific rates depending upon the assay and the composition of the growth media used.Citation28–Citation30 Similarly, Du et al. examined spontaneous [SWI+] formation and detected two [SWI+] isolates out of 48 candidates in one particular attempt.Citation11 While they note that they tried the experiment several times before succeeding, the ability to detect spontaneous [SWI+] formation from a relatively small yeast cell population strongly suggests that [SWI+] appears significantly more often than [PSI+], which would only be expected to appear at a rate of 5 out of 10,000,000 cells/generation. Taken together, the above estimates indicate that higher formation rates may be another characteristic of the N-rich prions discussed herein. When considered as a group, prions may form far more frequently than previously thought when [PSI+] was used as the primary example. In addition to having higher formation rates, we speculate that, based on their similar amino-acid compositions, the prions [MOT3+] and [ISP+] are likely to be less mitotically stable than [PSI+] and more sensitive to chaperone function. Further investigation is needed to test this hypothesis.
Yeast Prions as Quasi-Existent Elements: Is Strong [PSI+] a Representative Prion Specimen?
In describing the implications of our recent investigations, we, like others analyzing newly discovered prions, have relied heavily on comparisons with [PSI+]. Indeed, many of the paradigms regarding yeast prions have come from studies of [PSI+]. Because of the technical advantages of high mitotic stability and robust colony color phenotype, as well as its early discovery, strong [PSI+] has become the model prion against which others are compared. However, the comparisons described above suggest that strong [PSI+] represents an extreme example of indelibility among prions. [SWI+] stands as its antithesis, disappearing from the cell population at the first sign of trouble. The fact that multiple newly discovered prions have characteristics that are more similar to [SWI+] than to [PSI+], indicates that [SWI+] may actually be closer to the “norm” than [PSI+]. Thus, the characteristics of [SWI+] support the view that prions are dynamic elements that may be only transiently present in cell populations. The apparent absence of the prions [PSI+] and [URE3] from wild yeast strains has been suggested to be evidence that prions are diseases of yeast.Citation31 However, considering that many prion strains are inherently weak, having relatively high rates of loss and formation, it seems reasonable to expect that prions may frequently appear and disappear from a cell population. Thus, while prions are aptly described as meta-stable, we might also consider them to be quasi-existent.
Implications of Prion Instability
What is the physiological advantage to yeast of an instable prion? Indeed, whether prions in general are either beneficial to yeast or mal-adaptive is point of controversy.Citation10,Citation31 The N-rich prions [SWI+], [URE3], [MOT3+] and [ISP+] are all formed from proteins which regulate gene transcription. Indeed prion-forming proteins in general are enriched for transcriptional regulators.Citation24,Citation27 Transcriptional regulators may make especially potent prions because their effect on cell physiology is leveraged by pleotropic effects. For example, Swi1 sequestration due to the presence of [SWI+] causes a partial loss of SWI/SNF function, potentially altering the expression of hundreds of genes. It is unsurprising then that [SWI+] can be either advantageous or detrimental to yeast depending on the growth conditions.Citation11,Citation24 If [SWI+] is more commonly detrimental than beneficial, its instability would be desirable. Hence, as we speculated in our original investigation, the potency of the [SWI+] phenotype to affect cell physiology, perhaps often in a mal-adaptive fashion, may create a selective pressure to maintain [SWI+] as an easily lost prion. Thus chaperone sensitivity may be the result of an evolutionary trade-off, resulting in a powerful (but flaky) prion.
Financial Support
This work was supported by the National Institute of Health Grant GM31107 (E.A.C.) and National Institute of Health NRSA postdoctoral fellowship GM846542 (J.K.H.).
Note added in proof
A recent publication by the Lindquist laboratory (Halfmann, et al. Opposing effects of glutamine and asparagine govern prion formation by intrinsically disordered proteins. Mol Cell 2011; 43:72–84) has provided additional support for the idea presented herein that amino acid composition of a PrD, particularly the ratio of N to Q residues, affects both mitotic stability and the propensity for de novo prion formation.
Abbreviations
NEF | = | nucleotide exchange factor |
PrD | = | prion-forming domain |
ATP | = | adenosine triphosphate |
ADP | = | adenosine diphosphate |
Figures and Tables
Figure 1 The Cyclic Hsp70 Chaperone System. Ssa (purple), the yeast cytosolic Hsp70, binds and releases client polypeptides (blue) in a regulated and ATP-dependent manner. J-proteins (aquamarine) including Sis1, Ydj1 and others, stimulate Ssa ATP hydrolysis by virtue of a conserved J-domain and thereby catalyze the “forward” direction of the cycle as indicated above. ADP•Ssa more stably associates with client polypeptides than the ATP-bound form and hence J-proteins favor the ADP•Ssa•Peptide complex. In some cases, J-proteins can also bind and deliver client polypeptides to Hsp70s via C-terminal domains (also shown above). Nucleotide exchange factors (NEFs), including the Sse proteins (dark blue) which share some structural homology with Ssa, catalyze the “reverse” direction of the cycle by facilitating ADP release and subsequent ATP binding, and thus favor an ATP•Ssa state with a dissociated peptide.
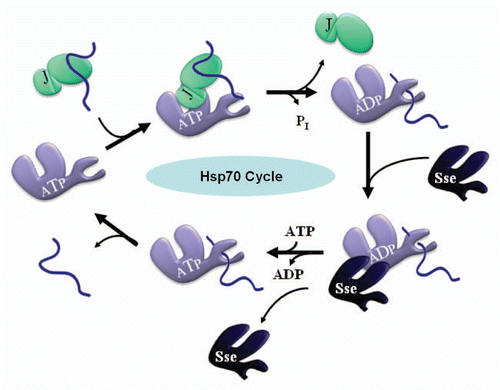
Table 1 Sensitivity of prions to ectopic chaperone expression
Table 2 Correlations amongst yeast prions
References
- Hines JK, Li X, Du Z, Higurashi T, Li L, Craig EA. [SWI], the prion formed by the chromatin remodeling factor Swi1, is highly sensitive to alterations in Hsp70 chaperone system activity. PLoS Genet 2011; 7:1001309
- Wickner RB, Edskes HK, Shewmaker F, Nakayashiki T. Prions of fungi: inherited structures and biological roles. Nat Rev Microbiol 2007; 5:611 - 618
- Jones GW, Tuite MF. Chaperoning prions: the cellular machinery for propagating an infectious protein?. Bioessays 2005; 27:823 - 832
- Satpute-Krishnan P, Langseth SX, Serio TR. Hsp104-dependent remodeling of prion complexes mediates protein-only inheritance. PLoS Biol 2007; 5:24
- Byrne LJ, Cole DJ, Cox BS, Ridout MS, Morgan BJ, Tuite MF. The number and transmission of [PSI] prion seeds (Propagons) in the yeast Saccharomyces cerevisiae. PLoS One 2009; 4:4670
- Tanaka M, Collins SR, Toyama BH, Weissman JS. The physical basis of how prion conformations determine strain phenotypes. Nature 2006; 442:585 - 589
- Higurashi T, Hines JK, Sahi C, Aron R, Craig EA. Specificity of the J-protein Sis1 in the propagation of 3 yeast prions. Proc Natl Acad Sci USA 2008; 105:16596 - 16601
- Tipton KA, Verges KJ, Weissman JS. In vivo monitoring of the prion replication cycle reveals a critical role for Sis1 in delivering substrates to Hsp104. Mol Cell 2008; 32:584 - 591
- Masison DC, Kirkland PA, Sharma D. Influence of Hsp70s and their regulators on yeast prion propagation. Prion 2009; 3:65 - 73
- Halfmann R, Lindquist S. Epigenetics in the extreme: prions and the inheritance of environmentally acquired traits. Science 2010; 330:629 - 632
- Du Z, Park KW, Yu H, Fan Q, Li L. Newly identified prion linked to the chromatin-remodeling factor Swi1 in Saccharomyces cerevisiae. Nat Genet 2008; 40:460 - 465
- Sondheimer N, Lopez N, Craig EA, Lindquist S. The role of Sis1 in the maintenance of the [RNQ+] prion. EMBO J 2001; 20:2435 - 2442
- Jung G, Jones G, Wegrzyn RD, Masison DC. A role for cytosolic hsp70 in yeast [PSI(+)] prion propagation and [PSI(+)] as a cellular stress. Genetics 2000; 156:559 - 570
- Halfmann R, Alberti S, Lindquist S. Prions, protein homeostasis and phenotypic diversity. Trends Cell Biol 2010; 20:125 - 133
- Newnam GP, Birchmore JL, Chernoff YO. Destabilization and recovery of a yeast prion after mild heat shock. J Mol Biol 2011; 408:432 - 448
- Tuite MF, Mundy CR, Cox BS. Agents that cause a high frequency of genetic change from [psi+] to [psi−] in Saccharomyces cerevisiae. Genetics 1981; 98:691 - 711
- Kushnirov VV, Kryndushkin DS, Boguta M, Smirnov VN, Ter-Avanesyan MD. Chaperones that cure yeast artificial [PSI+] and their prion-specific effects. Curr Biol 2000; 10:1443 - 1446
- Kushnirov VV, Kochneva-Pervukhova NV, Chechenova MB, Frolova NS, Ter-Avanesyan MD. Prion properties of the Sup35 protein of yeast Pichia methanolica. EMBO J 2000; 19:324 - 331
- Kryndushkin D, Wickner RB. Nucleotide exchange factors for Hsp70s are required for [URE3] prion propagation in Saccharomyces cerevisiae. Mol Biol Cell 2007; 18:2149 - 2154
- Crow E, Du Z, Li L. New insights into prion biology from the novel [SWI+] system. Prion 2008; 2:141 - 144
- Toombs JA, McCarty BR, Ross ED. Compositional determinants of prion formation in yeast. Mol Cell Biol 2010; 30:319 - 332
- Ross ED, Toombs JA. The effects of amino acid composition on yeast prion formation and prion domain interactions. Prion 2010; 4
- Petkova AT, Ishii Y, Balbach JJ, Antzutkin ON, Leapman RD, Delaglio F, et al. A structural model for Alzheimer's beta-amyloid fibrils based on experimental constraints from solid state NMR. Proc Natl Acad Sci USA 2002; 99:16742 - 16747
- Alberti S, Halfmann R, King O, Kapila A, Lindquist S. A systematic survey identifies prions and illuminates sequence features of prionogenic proteins. Cell 2009; 137:146 - 158
- Lancaster AK, Bardill JP, True HL, Masel J. The spontaneous appearance rate of the yeast prion [PSI+] and its implications for the evolution of the evolvability properties of the [PSI+] system. Genetics 2010; 184:393 - 400
- Volkov KV, Aksenova AY, Soom MJ, Osipov KV, Svitin AV, Kurischko C, et al. Novel non-Mendelian determinant involved in the control of translation accuracy in Saccharomyces cerevisiae. Genetics 2002; 160:25 - 36
- Rogoza T, Goginashvili A, Rodionova S, Ivanov M, Viktorovskaya O, Rubel A, et al. Non-Mendelian determinant [ISP+] in yeast is a nuclear-residing prion form of the global transcriptional regulator Sfp1. Proc Natl Acad Sci USA 2010; 107:10573 - 10577
- Wickner RB. [URE3] as an altered URE2 protein: evidence for a prion analog in Saccharomyces cerevisiae. Science 1994; 264:566 - 569
- Brachmann A, Toombs JA, Ross ED. Reporter assay systems for [URE3] detection and analysis. Methods 2006; 39:35 - 42
- Aigle M, Lacroute F. Genetical aspects of [URE3], a non-mitochondrial, cytoplasmically inherited mutation in yeast. Mol Gen Genet 1975; 136:327 - 335
- Wickner RB, Edskes HK, Kryndushkin D, McGlinchey R, Bateman D, Kelly A. Prion diseases of yeast: Amyloid structure and biology. Semin Cell Dev Biol 2011;