Abstract
Cellular prion (PrPc) undergoes a regulated α-secretase-like cleavage by the disintegrin ADAM17 similar to the one taking place on β-Amyloid Precursor Protein (βAPP). Because these cleavages give rise to biologically active fragments, understanding their regulation could be of importance. We have established that the Extracellular Regulated Kinase-1 (ERK1) controls PrPc processing by modulating ADAM17 phosphorylation in a protein kinase C-dependent manner. Strikingly, we also demonstrated that ERK1 acts upstream to increase PrPc promoter transactivation in an AP-1 dependent manner. Therefore, ERK1 exerts a dual control of both PrPc metabolism and expression. Interestingly, α-secretase cleavage of βAPP appears to be independent of ERK1. I describe here similarities and differences in α-secretase-mediated PrPc and βAPP processing pathways and discuss putative physiopathological implications.
The cellular prion (PrPC) is a fascinating protein that has been at the center of a biological revolution.Citation1 Thus, this protein is naturally occurring in animals and human beings and converts into a pathogenic form, prion scrapie (PrPSc) to trigger fatal diseases.Citation2 The “revolution” stands in the concept that this conversion of PrPC into PrPSc occurs in a self-propagating manner without yet identified co-factors or nucleic acids.Citation3 This puzzling observation aroused numerous works aimed at better understanding how PrPC aggregates, converts, disseminates, how the diseases are propagated and transmitted, what are the organs and species barriers, etc.Citation4
What about the putative physiological role(s) of PrPC? Numerous functions have been proposed but not yet firmly identified. Among others, PrPC could participate in lymphocyte activation, cell-cell adhesion and cellular signaling (reviewed in ref. Citation5). More discussed were the observations concerning PrPC function in the control of cell death. Thus, PrPC could display both protective and trophic phenotypes6 while alternatively, PrPC could also potentiate cell death and caspase-3 activation in several cell systems challenged with apoptotic stimuli.Citation7–Citation9 Some of the discrepancies could stand in the fact that PrPC is physiologically processed by an α-secretase-like cleavage that yields both N- and C-terminal fragments that display distinct phenotypes. Thus, the α-secretase-derived N-terminal fragment, referred to as N1, exhibits a protective function related to its ability to control the proapoptotic tumor suppressor p53Citation10 while the C-terminal counterpart C1 is clearly toxic and triggers p53-dependent cell death.Citation11 Therefore, the survival/toxic functions ascribed to PrPC could be linked to the balance between the levels of the two fragments generated upon proteolytic maturation and therefore, could be directly dependent upon the enzymatic machinery associated to the biological system in which PrPC function is examined. The scheme is even complicated by the fact that the two fragments could functionally interact. Thus, N1 is able to protect against both PrPC-and C1-associated staurosporine-stimulated caspase-3 activation. It is therefore of importance to understand the PrPC physiopathological maturation process.
Our very first data on PrPC processing was the result of a bet. Previous literature indicated that PrPC underwent in vivo cleavage within its putative domain (sequence 106–126).Citation12 Working on Alzheimer disease and more particularly on the physiopathological maturation of the β-amyloid precursor protein (βAPP),Citation13–Citation15 it was striking that the membrane cleavage of PrPC was clearly reminiscent of the one taking place in the middle of the putative toxic domain of βAPP (i.e., the Aβ sequence). Therefore, in both cases, it appeared that this cleavage could be seen as an inactivating process. The bet was when we questioned whether PrPC and βAPP could undergo such a cleavage by similar processes and activities. Thus, βAPP was reported to be proteolyzed within the Aβ domain by a series of proteases belonging to the disintegrins family, namely ADAM10 and ADAM17.Citation16,Citation17 However, there was no example of proteins linked to the membranes by a glycosylphosphatidyl inositol group (as is PrPC) that could behave as a disintegrin substrate.Citation18 Indeed, the bet was successful since we established that both PrPC and βAPP undergo constitutive and regulated α-secretase cleavages by ADAM10 and ADAM17, respectively.Citation19,Citation20
We recently established that the Extracellular Regulated Kinase-1 (ERK1) controls the regulated ADAM17-mediated cleavage of PrPCCitation21 but also acts upstream and contributes to the regulation of the PrPC promoter transactivation and mRNA levels.Citation21 Thus, N1 production could be altered by both genetic or pharmacological manipulations of ERK1 or its upstream kinase MEK as well as by means of constitutively active or dominant negative ERK-/MEK-liked constructs.Citation21 We showed that this ERK-associated control of PrPC-mediated α-secretase cleavage was due to ERK1 ability to phosphorylate, and thereby to increase, ADAM17 enzymatic activity.Citation21 Both protein kinase C-stimulated and muscarinic receptors-dependent α-secretase processing of PrPC were fully dependent of ERK1 pathway.Citation21
Interestingly, we also observed an ERK1-dependent modulation of endogenous PrPC expression that we ascribed to ERK1-associated increase in PrPC promoter transactivation.Citation21 In agreement with previous works showing that ERK1 triggers the activation of the AP-1 transcription factor,Citation22 we delineated an AP-1 consensus site on the PrPC promoter region, the mutation of which fully abolished ERK1-mediated PrPC promoter transactivation.Citation21 The above set of data indicated that ERK1 could modulate PrPC physiology at two distinct steps.
How could we rule out the possibility that ERK1-mediated control of N1 production could merely reflect the ability of the kinase to modulate PrPC transcription/expression and thus ADAM17 substrate? Three lines of data indicated that EK1-mediated control of PrPC expression and processing were independent events. First, ERK1 modulates ADAM17 activity as indicated by fluorimetric mesurements of the membrane embedded disintegrin activity on intact cells. Second, the expression of constitutively active MEK1 still triggers an increase of PrPC expression and promoter transactivation in fibroblasts devoid of ADAM17. Third, ERK-1 still modulates N1 in cells overexpressing a cmv-driven PrPC cDNA construct, i.e., in absence of endogenous promoter and therefore putative ERK-1/(AP-1)-linked responsive elements. Altogether, the above experiments undoubtedly support the conclusion that the ERK1 pathway independently controls both ADAM17-dependent N1 secretion and PrPC promoter transactivation and expression ().
It is interesting to note that although βAPP and PrPC share common proteolytic denominators,Citation23 there exist also a few differences that could be delineated. Thus, while the protein kinase C (PKC) isoforms α, δ and ε contributed to the regulated α-secretase cleavage of PrPC,Citation24 PKCδ did not participate to the PKC-stimulated βAPP processing.Citation25 Furthermore, unlike for PrPC, βAPP regulated α-secretase cleavage remained independent of ERK1 pathway.Citation25 The above data indicate that PrPC and βAPP processing could be modulated/activated by pharmacological approaches aimed at selectively potentiating the α-secretase cleavage of a given protein without directly interfering with the processing of the other.
Figures and Tables
Figure 1 Regulated α-secretase pathways of cellular prion and βAPP processing. M1 and M3 muscarinic receptors can be stimulated by acetylcholine and blocked by atropine. In the case of PrPC, subsequent to this agonist-receptor interaction, PKC stimulation triggers ERK1 phosphorylation and Thr735 phosphorylation of ADAM17, thereby increasing N1 production. Concomitantly, ERK1 increases PrPC promoter transactivation via AP-1 and thereby enhances PrPC expression. In the case of βAPP, PKC triggers ADAM17 phosphorylation by a yet unidentified intermediate effector that is distinct of ERK1. Both α-secretase cleavages yield fragments, N1 and sAPPα with similar protective properties.
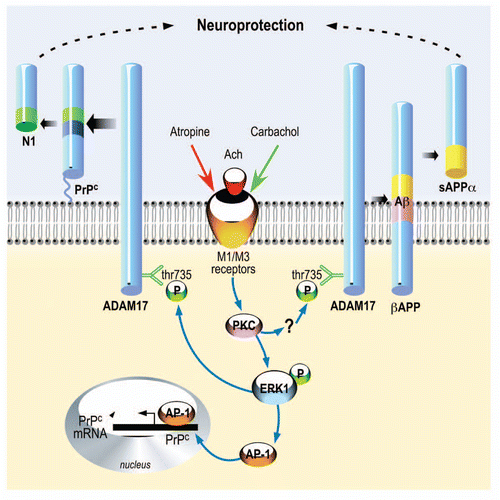
Acknowledgments
I wish to thank Franck Aguila for artwork. This work was supported by the Fondation pour la Recherche Médicale and the Conseil Général des Alpes Maritimes.
References
- Prusiner SB. Molecular biology and pathogenesis of prion diseases. Trends Biochem Sci 1996; 21:482 - 487; PMID: 9009832; http://dx.doi.org/10.1016/S0968-0004(96)10063-3
- Prusiner SB. Prions. Proc Natl Acad Sci USA 1998; 95:13363 - 13383; PMID: 9811807; http://dx.doi.org/10.1073/pnas.95.23.13363
- Tuite MF, Serio TR. The prion hypothesis: from biological anomaly to basic regulatory mechanism. Nat Rev Mol Cell Biol 2010; 11:823 - 833; PMID: 21081963; http://dx.doi.org/10.1038/nrm3007
- Aguzzi A, O'Connor T. Protein aggregation diseases: pathogenicity and therapeutic perspectives. Nat Rev Drug Discov 2010; 9:237 - 248; PMID: 20190788; http://dx.doi.org/10.1038/nrd3050
- Linden R, Martins VR, Prado MA, Cammarota M, Izquierdo I, Brentani RR. Physiology of the prion protein. Physiol Rev 2008; 88:673 - 728; PMID: 18391177; http://dx.doi.org/10.1152/physrev.00007.2007
- Martins VR, Beraldo FH, Hajj GN, Lopes MH, Lee KS, Prado MM, et al. Prion Protein: Orchestrating Neurotrophic Activities. Curr Issues Mol Biol 2010; 12:63 - 86; PMID: 19767651
- Paitel E, Fahraeus R, Checler F. Cellular prion protein sensitizes neurons to apoptotic stimuli through Mdm2-regulated and p53-dependent caspase 3-like activation. J Biol Chem 2003; 278:10061 - 10066; PMID: 12529324; http://dx.doi.org/10.1074/jbc.M211580200
- Paitel E, Sunyach C, Alves da Costa C, Bourdon JC, Vincent B, Checler F. Primary cultured neurons devoid of cellular prion display lower responsiveness to staurosporine through the control of p53 at both transcriptional and post-transcriptional levels. J Biol Chem 2004; 279:612 - 618; PMID: 14570892; http://dx.doi.org/10.1074/jbc.M310453200
- Sunyach C, Checler F. Combined pharmacological, mutational and cell biology approaches indicate that p53-dependent caspase 3 activation triggered by cellular prion is dependent on its endocytosis. J Neurochem 2005; 92:1399 - 1407; PMID: 15748158; http://dx.doi.org/10.1111/j.1471-4159.2004.02989.x
- Guillot-Sestier MV, Sunyach C, Druon C, Scarzello S, Checler F. The alpha-secretase-derived N-terminal product of cellular prion, N1, displays neuroprotective function in vitro and in vivo. J Biol Chem 2009; 284:35973 - 35986; PMID: 19850936; http://dx.doi.org/10.1074/jbc.M109.051086
- Sunyach C, Cisse MA, da Costa CA, Vincent B, Checler F. The C-terminal products of cellular prion protein processing, C1 and C2, exert distinct influence on p53-dependent staurosporine-induced caspase-3 activation. J Biol Chem 2007; 282:1956 - 1963; PMID: 17121821; http://dx.doi.org/10.1074/jbc.M609663200
- Chen SG, Teplow DB, Parchi P, Teller JK, Gambetti P, Autilio-Gambetti L. Truncated forms of the human prion protein in normal brain and in prion disease. J Biol Chem 1995; 270:19173 - 19180; PMID: 7642585; http://dx.doi.org/10.1074/jbc.270.32.19173
- Ancolio K, Marambaud P, Dauch P, Checler F. α-secretase-derived product of β-amyloid precursor protein is decreased by presenilin 1 mutations linked to familial Alzheimer's disease. J Neurochem 1997; 69:2494 - 2499; PMID: 9375682; http://dx.doi.org/10.1046/j.1471-4159.1997.69062494.x
- Lopez-Perez E, Zhang Y, Frank SJ, Creemers J, Seidah N, Checler F. Constitutive alpha-secretase cleavage of the beta-amyloid precursor protein in the furin-deficient LoVo cell line: involvement of the pro-hormone convertase 7 and the disintegrin metalloprotease ADAM10. J Neurochem 2001; 76:1532 - 1539; PMID: 11238737; http://dx.doi.org/10.1046/j.1471-4159.2001.00180.x
- Marambaud P, Lopez-Perez E, Wilk S, Checler F. Constitutive and protein kinase C-regulated secretory cleavage of Alzheimer's β amyloid precursor protein: different control of early and late events by the proteasome. J Neurochem 1997; 69:2500 - 2505; PMID: 9375683; http://dx.doi.org/10.1046/j.1471-4159.1997.69062500.x
- Lammich S, Kojro E, Postina R, Gilbert S, Pfeiffer R, Jasionowski M, et al. Constitutive and regulated α-secretase cleavage of Alzheimer's amyloid precursor protein by a disintegrin metalloprotease. Proc Natl Acad Sci USA 1999; 96:3922 - 3927; PMID: 10097139; http://dx.doi.org/10.1073/pnas.96.7.3922
- Buxbaum JD, Liu KN, Luo Y, Slack JL, Stocking KL, Peschon JJ, et al. Evidence that tumor necrosis factor α converting enzyme is involved in regulated α-secretase cleavage of the Alzheimer amyloid protein precursor. J Biol Chem 1998; 273:27765 - 27767; PMID: 9774383; http://dx.doi.org/10.1074/jbc.273.43.27765
- Vincent B, Checler F. α-secretase in Alzheimer's disease and beyond: mechanistic, regulation and function. Curr Alzheimer Res 2011; In press PMID: 21605031
- Vincent B, Paitel E, Frobert Y, Lehmann S, Grassi J, Checler F. Phorbol ester-regulated cleavage of normal prion protein in HEK293 human cells and murine neurons. J Biol Chem 2000; 275:35612 - 35616; PMID: 10952979; http://dx.doi.org/10.1074/jbc.M004628200
- Vincent B, Paitel E, Saftig P, Frobert Y, Hartmann D, De Strooper B, et al. The disintegrins ADAM10 and TACE contribute to the constitutive and phorbol ester-regulated normal cleavage of the cellular prion protein. J Biol Chem 2001; 276:37743 - 37746; PMID: 11477090
- Cissé M, Duplan E, Guillot-Sestier MV, Rumigny J, Bauer C, Pages G, et al. The extracellular regulated kinase-1 (ERK1) controls regulated {alpha}-secretase-mediated processing, promoter transactivation and mRNA levels of the cellular prion protein. J Biol Chem 2011; 286:29192 - 29206; PMID: 21586567; http://dx.doi.org/10.1074/jbc.M110.208249
- Boutros T, Chevet E, Metrakos P. Mitogen-activated protein (MAP) kinase/MAP kinase phosphatase regulation: roles in cell growth, death and cancer. Pharmacol Rev 2008; 60:261 - 310; PMID: 18922965; http://dx.doi.org/10.1124/pr.107.00106
- Checler F, Vincent B. Alzheimer's and prion diseases: distinct pathologies, common proteolytic denominators. Trends Neurosci 2002; 25:616 - 620; PMID: 12446128; http://dx.doi.org/10.1016/S0166-2236(02)02263-4
- Alfa Cissé M, Louis K, Braun U, Mari B, Leitges M, Slack BE, et al. Isoform-specific contribution of protein kinase C to prion processing. Mol Cell Neurosci 2008; 39:400 - 410; PMID: 18722532; http://dx.doi.org/10.1016/j.mcn.2008.07.013
- Cisse M, Braun U, Leitges M, Fisher A, Pages G, Checler F, et al. ERK1-independent alpha-secretase cut of beta-amyloid precursor protein via M1 muscarinic receptors and PKCalpha/epsilon. Mol Cell Neurosci 2011; 47:223 - 232; PMID: 21570469; http://dx.doi.org/10.1016/j.mcn.2011.04.008