Abstract
Exocytosis molecular mechanisms in plant cells are not fully understood. The full characterisation of molecular determinants, such as SNAREs, for the specificity in vesicles delivery to the plasma membrane should shed some light on these mechanisms. Nicotiana tabacum Syntaxin 1 (NtSyr1 or SYP121) is a SNARE protein required for ABA control of ion channels and appears involved in the exocytosis of exogenous markers. NtSyr1 is mainly localised on the plasma membrane, but when over expressed the protein also appears on endomembranes. Since NtSyr1 is a tail-anchored protein inserted into the target membrane post-translationally, it is not clear whether its initial anchoring site is the ER or the plasma membrane. In this study, we investigated the sorting events of NtSyr1 in vivo using its full-length cDNA or its C-terminal domain, fused to a GFP tag and transiently expressed in protoplasts or in the leaves of Nicotiana tabacum cv. SR1. Five chimeras were produced of which two were useful to investigate the protein sorting within the endomembrane system. One (GFP-H3M) had a dominant negative effect on exocytosis; the other one (SP1-GFP) resulted in a slow targeting to the same localisation of the full-length chimera (GFP-SP1). The insertion of signal peptides on SP1-GFP further characterised the insertion site for this protein. Our data indicates that NtSyr1 is firstly anchored on ER membrane and then sorted to plasma membrane.
Introduction
The Soluble N-ethyl-maleimide sensitive factor Attachment protein Receptors (SNARE proteins) are involved in the membrane fusion during vesicle trafficking. Nicotiana tabacum Syntaxin 1 (NtSyr1 or SYP121) is a SNARE protein required for ABA control of ion channelsCitation1–Citation3 and appears to be involved in exocytosis. In fact, the expression of a dominant negative mutant of NtSyr1 shows that this syntaxin mediates the traffic between the Golgi complex and the plasma membrane.Citation4
To date, little is known about the molecular events and post-transcriptional control of exocytosisCitation4,Citation5 in plant cells. The full characterization of molecular determinants, such as syntaxins, which are involved in vesicle delivery to the plasma membrane, should shed light on these mechanisms. Syntaxins are cytoplasmically oriented tail-anchored (TA) proteins inserted through the membrane with a C-terminal hydrophobic domain.Citation6 As a consequence, TA proteins must be targeted to the appropriate membrane, post-translationally. This targeting process distinguishes them from classical type II membrane proteins, which are delivered to the ER by the SRP-dependent cotranslational pathway. Usually very few polar residues are present in TA proteins downstream the C-terminal hydrophobic domain and it is difficult to verify whether they span the bilayer. However their exact topology remains controversial even if it has been demonstrated that some TA proteins can translocate their COOH terminus across the bilayer.Citation7,Citation8
NtSyr1, like all syntaxins, has 3 domains: (Ha/b/c) with high probability of forming coiled-coil structures in protein-protein interactions, an adjacent domain (H3) of 84% identity (92% homology) with the epimorphin consensus sequence and the C-terminal trans-membrane domain (TMD) that works as tail-anchor (TA).Citation1
NtSyr1 has been mainly localized on the plasma membrane (PM) but it has also shown to be spread through the endomembrane system when overexpressed.Citation2 However, it is not clear yet whether the PM is the direct insertion site or the final destination of sorting events.
NtSyr1 expressed from a cDNA was named SP1. SP1 was fused to a GFP tag and expressed in Nicotiana tabacum cv. SR1 protoplasts and leaf epidermal cells to examine the localization and intracellular trafficking of this protein directly by confocal microscopy. The distribution and the fluorescent pattern of five different chimeras were analysed and allowed us to confirm the localization of NtSyr1 on the PM and to define some aspects of its sorting.
Materials and Methods
Gene constructs and vector mobilization.
Chimeras were obtained by fusing a GFP sequence containing the S65T mutation to NtSyr1 domains through the insertion, in this last sequence, of cohesive specific restriction sites. GFP fragments with appropriate cohesive restriction ends were already in preexisting constructsCitation9 in a pUC19-derived vector for protoplast transformation.Citation10 A BamHI/NheI fragment was used for N-terminal fusions to GFP (SP1-GFP, SP2-GFP) and a SalI/PstI fragment (GFP-SP1, GFP-SP2) or BglII/PstI fragment (GFP-H3M) for C-terminal fusions. Point mutations to create restriction sites in NtSyr1 were obtained by PCR using the following primers: STX3 (gcggatccatgaatgatctatttt-for) and 2NtSP (agacaaagctagcttttttccatggc-rev) for SP1-GFP; STX3 and 1NtSP (gaagaatgctagcaaaacaagtcc-rev) for SP2-GFP; STXFSal (gtcgaccat-gaatgatctattttcaggatc-for) and STX1 (gcctgcagtcattttttccatggc-rev) for GFP-SP1; STXFSal and STX2 (gcctgcagttaacaagtccatttt-rev) for GFP-SP2; 2CtSP (ttataccgtcacaggagatcttcc-for) and STX1 for GFP-H3M. Plasmid DNA was used for transient transformation of protoplasts after column purification following the Qiagen plasmid purification method. These modified genes were then inserted into the binary vector pBinCitation11–Citation13 and Agrobacterium tumefaciens (GV3101) was transformed by triparental mating using the E. coli helper strain HB101 pRK2013. The construct SP1-GFP was further modified by the insertion of targeting signals. SP1-GFPKDEL and SP1-GFPChi were obtained by the substitution of the C-terminus of SP1-GFP, between the restriction sites ClaI/PstI, with the C-terminus of sGFPKDEL and GFP-ChiCitation9 respectively. The ssVSD, used to produce the construct SP1-AleuGFP, was obtained inserting, by PCR, a new NheI restriction site in the sequence of AleuGFP6Citation9 by using the primers 005 (gctagcgccgtcgcctcctcctcctcctt-for) and 004 (taatgatcagcgagttgcacgccgccgtcttcg-rev). The amplification product produced an NheI/NheI fragment then inserted in SP1-GFP. The correct ligation of the fragment was verified by PCR with the primers 2CtSP/004.
Protoplast transient expression.
Nicotiana tabacum cv. SR1 protoplasts were isolated following the protocol of Maliga and coworkers,Citation14 cultured and rinsed using the indicated media and transformed by PEG-mediated direct gene transfer essentially as described.Citation15,Citation16 Ten micrograms plasmid were used for the transformation of about 600000 protoplasts. After two hours protoplasts were rinsed to remove the PEG, resuspended in 2 ml culture medium and incubated at 26°C in the dark.
FM4-64 dye staining.
For staining protoplasts, the dye FM4-64 (Molecular Probes, Leiden, The Netherlands) was used in a concentration of 100 µM, from a stock (1 mM) in 0.4 M mannitol. Within the first 10 minutes the dye stains only PM of protoplasts, then is rapidly internalized. Images were produced from 30 to 60 minutes after staining.
Confocal microscopy.
Protoplasts transiently expressing sec-GFP were observed by fluorescence microscopy in their culture medium at different times after transformation; leaf epidermis was mounted in water. They were examined by using a confocal laser-microscope LSM Pascal Zeiss. GFP was detected with the filter set for FITC (505–530 nm) while chlorophyll epifluorescence was detected with the filter set for TRITC (>650 nm) with 488-nm excitation. To detect FM4-64 fluorescence, the He-Ne laser was used to produce a 543-nm excitation and the emission was recorded with the 560–615 nm filter set.
Transient expression of GFPs in leaf.
Agrobacterium tumefaciens GV3101 bacterial cultures were incubated at 28°C with agitation until stationary growth phase and used for direct infiltration of leaf tissue as previously described.Citation17 GFP-dependent fluorescence of lower epidermis was analyzed within 24 hours post-infiltration.
Protein extraction from protoplasts and enzymatic tests.
Protoplasts were harvested by 5 min centrifugation at 65 g, resuspended in 0.1 M Na-acetate pH 5 and lysed by three cycles of freezing (in liquid nitrogen) and thawing. The soluble proteins were separated from insoluble residues by centrifugation, 5 minutes at 10000 g. The extract was directly used to measure enzymatic activity of secreted rat β-glucuronidase (secRGUS) and α-mannosidase (the constitutive enzyme used as internal control). Measurements were made in a RF-5301 Shimadzu PC Spectrofluorophotometer. The used reaction substrate was 4-Methyl-Umbelliferyl-β-D-Glucuronide (BIOSYNTH, Staad, Switzerland) and 4-Methyl-Umbelliferyl-a-D-Mannoside (SIGMA, Steinheim, Germany) to test secRGUS and mannosidase activity respectively. Tests under normal conditions were normalized by comparing secRGUS activity to the internal control (α-mannosidase); both samples were excited at 370 nm and fluorescence measured at 480 nm.
Protein extraction for blotting.
Protoplasts were harvested by 5 min centrifugation at 65 g and resuspended in the extraction buffer (TBS 1% supplemented with Proteinase inhibitors cocktail “Complete” by Roche). Protoplasts were lysed by three consecutive freezing-thawing cycles. Lysed cells were centrifuged for 30 min at 14000 g. Supernatant was considered to contain the soluble protein fraction, the pellet was resuspended in the extraction buffer supplemented with 2% SDS and left at room temperature for 10 minutes to solubilize membrane proteins. Insoluble aggregates in the membrane fraction were removed with a short centrifugation at 10000 g. “Soluble protein” and “membrane bound proteins” fractions were precipitated by 10% TCA and washed with acetone. Pellets were resuspended in volumes proportionate to the original sample for gel analysis.
Western blotting and immunolabeling.
The proteins were separated in polyacrylamide gels with SDS (4% stacking gel, 15% separation gel).Citation18 The proteins were separated in a minigel system “Mini-Protean II Dual Slab Gel System” from Biorad. Then they were electrophoretically transferred on a nitro-cellulose membrane (Hybond-C Extra) and incubated in 100 ml milk-TBS 5% (20 mM Tris-Cl pH7.5; 500 mM NaCl; 5% w/v milk powder) to saturate the nitro-cellulose membrane with proteins over night. GFP detection was obtained by using anti GFP (Molecular Probes A6455) and anti-NtSyr1Citation2 primary antibodies; anti-rabbit secondary antibodies coupled to peroxidase were used for both detections.
Results
GFP fusions and association to membranes.
The sorting and the final localization of NtSyr1, were examined by fusing NtSyr1, or some of its domains, to a plant adapted GFP coding sequence.Citation9 Five chimeras were prepared; the constructs and the amino acidic sequences used for each fusion are shown in .
GFP-SP1 construct was made by fusing GFP to the N-terminus of SP1. GFP-H3M construct was made by fusing GFP to the N-terminus of a large C-terminal portion of SP1, which included H3 and TMD but not the regulatory domain Ha/b/c. In these two constructs, the TMD remains exposed at the C-terminus and its insertion into the membrane should not be obstacled.
In the chimera SP1-GFP, GFP was fused to the C-terminus of SP1, following the TMD in the coding sequence. This orientation may represent a possible obstacle to TMD function. In the last two chimeras, GFP was fused to SP1 deprived of the TMD; the soluble variant of SP1 is named SP2.Citation4 GFP was fused to N-terminus (GFP-SP2) or C-terminus (SP2-GFP) of SP2.
The constructs GFP-SP1, GFP-H3M and SP1-GFP, all of which include the TMD, were associated with membrane fractions, whereas SP2-GFP, GFP-SP2 and GFP were found in the cytosol (). All full-length fusions showed degradation products. It was possible to deduce which part of the polypeptide was degraded by comparing the immunolabeling with two different antibodies: anti-GFP serum and anti-NtSyr1 serum. GFP-SP1 was degraded at the N-terminus since one degradation product was detected only by anti-SP1 serum. SP1-GFP chimera was also degraded at the N-terminus but smaller bands were obtained by both anti-GFP and anti-SP1 serum ().
Effect on a secretory marker.
It is known that SP2, the soluble variant of SP1, affects secretionCitation4 because of the competition with the endogenous protein for the SNARE complex interactors. An overexpression of SP1, the functional membrane protein, masks this negative effect.Citation4 Starting from these evidences, we have tested the effect of our chimeras on the secretory marker secRGUSCitation19 obtained with the deletion of the last 15 amino acids from the C-terminus of the rat preputial β-glucuronidase (RGUS).Citation20 The enzymatic activity of secRGUS inside the cells, after 18 hours of transient expression of the marker, was compared with the enzymatic activity in the medium, obtaining a percentage value of the secretion efficiency. The contamination of the medium with the intracellular fraction was corrected to evaluate the contamination of the intracellular endogenous marker α-mannosidase.
The fusions GFP-SP1 and SP1-GFP had no effect on secRGUS secretion, indicating that these chimeras were functional. GFP-H3M showed an inhibitory effect comparable to SP2, suggesting that these fusions competed with the endogenous protein SP1 (). GFP-H3M lacks the regulatory domain (Ha/b/c) that allows the formation of the functional SNARE complex only when the syntaxin reaches the target membrane. Therefore it was not surprising to evidence the competitive effect as observed with SP2. Two different proteins were used as control: a cytosolic GFP and a PM targeted protein, named PMA4-GFP.Citation21
Distribution patterns of chimeras.
A few hours after transient expression, GFP-SP1 was clearly visible, uniformly distributed on the PM (). The distribution pattern was comparable to the distribution of PMA4-GFP PM markerCitation21 (). This pattern did not change in time, even after 30 hours after transformation. Only protoplasts which were incubated at 12°C for 12 hours showed fluorescence in internal compartments. In such conditions cellular compartments were not well defined but ER-like network may be recognized (data not shown).
SP2-GFP as well as GFP-SP2, labelled all cytosol and the nuclear space () with the typical fluorescent pattern of soluble GFPs. GFP-H3M exhibited a complex pattern evolving in time. At the beginning of transgene expression it was possible to observe small GFP labelled compartments (). This pattern was called “early pattern” (E.P.) because, after the peak of expression at 18 hours, it became rare and a new pattern, called “late pattern” (L.P.), appeared. The L.P. was characterized by the concentration of the protein in patches on the cell surface () and internal compartments larger than 2µm appeared with time. The compartments labelled by this fusion protein varied in size and showed a nonfluorescent lumen since GFP was on the cytoplasmic face of the membrane ( and enlarged view), appearing similar to abnormal endosomes.Citation23
SP1-GFP fluorescent pattern, in the early stages of expression, labelled ER and many small compartments () similar to the fluorescent pattern observed in the E.P. of GFP-H3M. With time, the protein reached the cell surface labelling the PM more or less homogeneously (). Later, in a restricted percentage of protoplasts, it was internalized in compartments () similar to L.P. of GFP-H3M. The lumen of such compartments was often fluorescent probably because GFP was on the luminal face of the membrane (, enlarged view). Anyhow SP1-GFP pattern showing a labelled PM was the most common and many cells exhibited a fluorescent pattern very similar to that of GFP-SP1.
Since GFP-H3M and SP1-GFP were transported to the PM and showed a complex fluorescent pattern with a clear transition from an E.P. to a L.P., we continued our analysis on these chimeras to reveal different steps of the protein sorting. With GFP-H3M it was possible to follow the progression of the appearing of large compartments, typical of the L.P. Eight hours after transformation, the L.P. was visible in less than 10% of protoplasts; 22 hours later it was common to about 30% of the cells ().
GFP-chimeras were sensitive to SP2 competition.
We showed that GFP-SP1 chimera labelled exclusively the PM (), confirming the expected localization of SP1.Citation2 In the presence of SP2, after more than 24 hours of transient expression, the distribution pattern of GFP-SP1 was altered and fluorescence appeared concentrated in patches on the cell PM and internalized in large compartments (), very similar to those observed with GFP-H3M and SP1-GFP ( and I). A sensitive increase of fluorescence in the ER and other internal compartments was also detected after coexpression of SP1-GFP and SP2 ().
Progressing with time, GFP-H3M accumulated into endosome-like structures in a larger number of cells (). To test if this chimera was sensitive to SP2 and correlated to ABA signalling,Citation1 the transition from E.P. to L.P. was measured within the protoplasts population in different conditions.
We followed the evolution of the pattern of GFP-H3M in the presence of either SP2 or ABA. SP2 slowed the formation of internal compartments and ABA (40 µM) modified the kinetic of their formation as well as their number ().
Colocalization of chimeric GFPs with the dye FM4-64.
Although both GFP-H3M and SP1-GFP showed a time related transition from an E.P. to a L.P., they behaved differently. To examine the endocytotic origin of L.P. compartments labelled by these chimeras, cells were stained by FM4-64, a marker for endosome or putative endosome compartments.Citation24,Citation25 The dye labelled the PM and was rapidly internalized by the endocytic pathway. Between 30 and 60 minutes after staining, most of labelling was intracellular. The small punctuate structures labelled by GFP-H3M in the E.P. did not fully colocalize with FM4-64 as shown in ), but the large structures observed with the L.P. did show co localization with FM4-64 (). The small punctate structures labelled by SP1-GFP in the E.P. did not fully colocalize with FM4-64 (), neither did the large fluorescent bodies of the L.P. ().
SP1 fusions are inserted first in the ER membrane.
The only evidence that GFP-SP1 could be present on the ER membrane, derived from the observation of ER-like structures after incubation at 12°C for about 8 hours (not shown). The other chimeras were more informative on this regard. The distribution of the small compartments and structures observed in the E.P., suggested that GFP-H3M and SP1-GFP first labelled the ER. These proteins were inserted into the ER and then transported to the PM. We tested this assumption by treating the transformed protoplasts with high doses of Brefeldin A (BFA; 50–100 µg/ml) to block transport from ER to PM through the Golgi. Some fluorescent BFA bodies rapidly appeared but a complete reorganization of the normal pattern was not observed.
We observed that the lumen of the fluorescent compartments of the L.P. of SP1-GFP was fluorescent while that of GFP-H3M was not ( and ). This was likely due to a different exposition of the GFP-containing portion into membrane compartment. In the SP1-GFP constructs, GFP seemed exposed to the luminal face of ER membrane. To support this idea, we designed three variants of SP1-GFP. At the GFP C-terminus of the first variant, an ER retention signal KDEL was added (SP1-GFPKDEL). In the second variant, to GFP C-terminus a vacuolar sorting determinant (ctVSD) (Di Sansebastiano et al., 1998) (SP1-GFPChi) was inserted and, in the third variant, GFP was preceded by a sequence specific VSD (ssVSD)Citation9 (SP1-AleuGFP).
The SP1-GFP characteristic patterns () were modified by the presence of the KDEL signal. The sorting information of this signal became dominant and all cells showed a fluorescent ER (). The ctVSD did not have significant effects on the fluorescent pattern till the appearance of the L.P. (). On the other hand the ssVSD completely over run the sorting of SP1-GFP deviating all fluorescence in small punctuate structures similar to prevacuolar compartments (PVC)Citation9 (). These fusions were also broken down by releasing soluble GFPs, so we cannot exclude that what we observed was due to cleaved free GFPs observed as degradation product in western-blots (data not shown). Nevertheless, the signals on the fusion proteins with GFP were efficiently recognized by specific receptors present within the secretory system and, in the case of KDEL, retained in the ER lumen.
GFP-SP1 and GFP-H3M transient expression in leaves.
GFP-SP1 and GFP-H3M constructs were transiently expressed in the leaf tissues of N. tabacum after agroinfiltration. The sub-axial epidermis of the leaf was infiltrated with an Agrobacterium suspension carrying one of the GFP-construct. GFP fluorescence was well visualized in the epidermal cells of fresh tissue by confocal microscopy 12 hours after infiltration. All confocal observations and images were performed within the first 24 hours, since longer expression periods turned out to be lethal for GFP-H3M expressing tissues. The entire area infiltrated with Agrobacterium carrying GFP-H3M turned to be unable to maintain turgor and the tissue died ().
The same fluorescent patterns described in protoplasts were also visible in epidermal cells. GFP-SP1 was visible in a sharp line around the cell; interpreted as PM and analogous to the pattern observed in protoplasts, this pattern was common to all observed cells. Occasionally few punctuate structures were also visible inside the cells labelled by the fluorescent PM (). Transient transformation with a cytosolic GFP was performed in parallel as a control () obtaining similar transformation efficiency when fluorescence was observed within the first 18–24 hours.
GFP-H3M had various fluorescent patterns: it was accumulated in ER associated to small compartments () or distributed in the cell's periphery associated to the PM (). When observed in protoplasts, this distribution was called E.P. In other cells it was accumulated in endosome-like compartments () similar to those observed in protoplasts L.P. In epidermal cells, it was not possible to describe a transition from one pattern to another, so that the definition of an early or late pattern was limited to the protoplasts. In leaves the pattern described were present one next to the other, depending just by the metabolism of the single cell.
Discussion
SNAREs are considered as typical TA proteins that reach their target membranes post-translationally.Citation6 It has also been reported that, to reach compartments of the secretory pathway, TA proteins are firstly inserted into the ER membrane and then delivered to their final destination by vesicular transport.Citation26,Citation27 In this study we report the sorting and the final localization of the syntaxin NtSyr1, also known as SYP121 and named SP1 when expressed from its cDNA, by using three different fusions with GFP. Immunocytochemical studies showed that NtSyr1 is mainly localized on the plasma membrane.Citation1,Citation2 Here we confirm this localization in vivo. The chimera obtained by fusing the GFP tag to the N-terminus of SP1 (GFP-SP1) showed a fluorescent pattern localised on the PM, but it did not allow to visualize any intermediate steps of its sorting. On the contrary, the two other constructs described in this work, GFP-H3M and SP1-GFP, showed a complex distribution pattern on cell endomembranes and provide useful models for the investigation of SP1 sorting within the endomembrane system.
For different reasons, the chimeras GFP-H3M and SP1-GFP were not expected to be functional: GFP-H3M because it lacked of the regulatory domains (Ha/b/c); SP1-GFP because its membrane anchoring tail would have been altered. The two different constructs showed analogies between the fluorescent patterns. Both GFP-H3M and SP1-GFP exhibited a fluorescent distribution evolving from an early (E.P.) to a late pattern (L.P.). In both cases, the large internal compartments characteristic of the L.P. appeared only after the arrival of the chimeras to the PM, so that they could be reasonably due to endocytosis events. The labelling of the characteristic structures of the L.P with the dye FM4-64 evidenced their endocytotic origin. FM4-64 also showed that ER associated structures related to E.P. of both constructs were not endosomes. Some of the compartments labelled by SP1-GFP showed a fluorescent lumen so that SP1-GFP seemed to be inserted into the endomembrane system with GFP resided into the lumen. The exposition of the GFP in the lumen of pre-Golgi compartments was proved by the efficient interaction of modified SP1-GFP with the KDEL signal receptor ERD2Citation28 and the ssVSD signal receptor BP-80.Citation29 The signal recognised by these receptors influenced drastically the distribution of the fluorescent protein. Interestingly, a ctVSD, for which a receptor has never been identified because of the low specificity of the binding, did not show an interaction to be able to alter the normal sorting of the chimeric protein.
The appearing of an E.P. for SP1-GFP, similar to that of GFP-H3M, could be due to a delay of the protein to transit through the ER membranes for the presence of GFP in the lumen. It has been reported that not only TMD plays a key role in TA protein trafficking, but also that the cytosolic domain often contributes to their sorting. This is the case for the Golgi localised TA proteins. Likewise the determinants responsible for SNARE trafficking reside both in the TMD and in the cytosolic domain.Citation30 We have verified that the presence of a luminal domain can also contribute to the sorting of the protein; this is the case of SP1-GFP whose secretion has slowed down by the presence of luminal GFP. The L.P. could be due in part to the recycling of not functional proteins and in part to the degraded forms of the chimera showing GFP, retained in the endomembrane system before the targeting to the PM. In fact the L.P. of this construct was characterised by the usual, large endocytotic compartments but also by other unidentified vacuolar structures not labelled by FM4-64; cells showing such fluorescent patterns represented a very low percentage in the protoplast population.
As SP1, the chimera GFP-SP1 did not affect the marker secRGUS secretion. The constructs GFP-H3M and SP1-GFP showed different effects on secRGUS indicating that, despite the analogies in their sorting events, the two chimeras behaved differently. GFP-H3M interacts with the partners of the SNARE complex but cannot be regulated by Sec-like proteins. GFP-H3M inhibition effect on the secretion system could have various targets on vesicles or on the target membrane. SP1-GFP, on the contrary, was probably down regulated normally since it possess the regulatory sequences which is free to interact with Sec-like proteins that keep the syntaxin inactive until it reaches the PM. SP1-GFP problem could be just a spatial obstacle during anchoring. For this reason, the effect of this chimera on secRGUS secretion was not relevant as it was for GFP-H3M.
As previously observed with an overexpression of SP1, the protein can be found on internal membranes other than on plasma membrane, but this does not disturb protein secretion.Citation2 To disturb the endogenous NtSyr1 dependent membrane traffic, we used SP2 (the soluble form of SP1) that cannot be tail anchored to the membranes because of the lack of the TMD.Citation4 SP2 still possess all interacting domains of SP1 and competes with the endogenous form for the interacting proteins. This competition has an inhibitory effect on secretion.Citation2,Citation31 If competition involved normal partners of SP1/NtSyr1 and such partners also interacted with GFP chimeras, it was reasonable to obtain an effect of SP2 on their fluorescent pattern distribution. Coexpression of SP2 with GFP-SP1 induced relocation of this chimera from the PM to internal compartments similar to endosomes. This pattern was similar to the L.P. of GFP-H3M and SP1-GFP, confirming some steps of the protein normal sorting. Data about coexpression of SP2 with GFP-H3M have been useful to clarify some aspects of the chimera distribution. In this protein the N-terminal regulatory domains of syntaxin were deleted, a fine regulation of SNARE complex assembling was probably prevented. The fluorescence associated to ER and punctuates structures, typical of the E.P., was likely related to aspecific interactions of the protein with other SNARE on the wrong membranes, which may delay the normal sorting speed. The internal compartment, characteristic of the L.P., and very similar to abnormal endosomes,Citation23 may be due to the recycling of non-functioning proteins at the end of its sorting. We suggest that the big size of such compartments was due to the overloading with GFP chimeras. Tw o considerations make us prefer this interpretation to the possibility that NtSyr1 regulates only the endocytic pathway: first, GFP- H3M and SP2 reduced the secretion of secRGUS marker; second, GFP-H3M and SP2 coexpression reduced the formation of the L.P., demonstrating that GFP-H3M sorting is related to specific interactions before the arrival to the PM. SP2 induced endosomes formation for GFP-SP1 but prevented their formation with GFP-H3M. This is probably due to the fact that GFP-SP1 is inactive because of the link with a Sec-like protein till its arrival to the PM, then the visible alteration of the pattern was due to an abnormal recycling in endosomes because of the lack of interactors. On the other hand, GFP-H3M was always sensitive to competition for interactors, even before its arrival on the PM.
The correlation between GFP-H3M sorting and both the dominant negative effect of SP2 and the positive ABA stimulus proved that this chimera was sorted by the same machinery of SP1 and interacted with the membrane similarly to the endogenous protein. So that, even if GFP-SP1 did not reveal intermediate steps of its sorting, the visualization of ER during E.P. of GFP-H3M and SP1-GFP identified the initial steps of such sorting. Brefeldin A (BFA), traditionally used to dissect the secretory pathway was not very effective on its sorting, nevertheless, formation of some BFA bodies containing GFP-SP1 (data not shown), confirmed the association of membranes anchored GFP-chimeras with the secretory pathway. Our interpretation of the data indicates that SP1, and consequently NtSyr1, is anchored on preGolgi membranes and then sorted to the PM.
Premature interaction of syntaxin and its proteic partners on the ER must be strictly prevented for normal exocytosis.Citation32,Citation33 So that, if SP1/NtSyr1 is anchored to the ER membrane, regulation of its sorting becomes an important process to investigate.
NtSyr1 is candidate to regulate exocytosis post-transcriptionally, but recently it has been also described as a regulatory element of endocytosis.Citation34 Our observations on SP2 effect on the sorting of different chimeras and their effect on exocytosis, support the first hypothesis and provide molecular tools for further investigations, nevertheless the second hypothesis cannot be ruled out and the two functions may coexist.
The punctuate structures appearing when GFP-H3M or SP1-GFP are expressed, have not been identified yet and may represent an intermediate accumulation site before exocytosis. In fact, if NtSyr1 is involved in post-transcriptionally regulated secretory events, ABA dependent, and the pool of secretory vesicles, ready to answer ABA stimulus, may also assemble in structures similar to the secretory granulesCitation35 described in animal systems.
The use of GFP chimeras may help in the identification of such transitory structures. We correlated these structures with a phase of sorting and verified their presence also in leaf cells. Leaf cells represent a better material to apply more advanced microscopy techniques. Next step will be the observation of these structures by electron microscopy techniques.
Figures and Tables
Figure 1 Schematic representation of the used GFP constructs. White color indicates GFP gene, different grey tones indicate NtSyr1 cDNA coding regions. Amino acidic sequences at the fusion points are indicated.
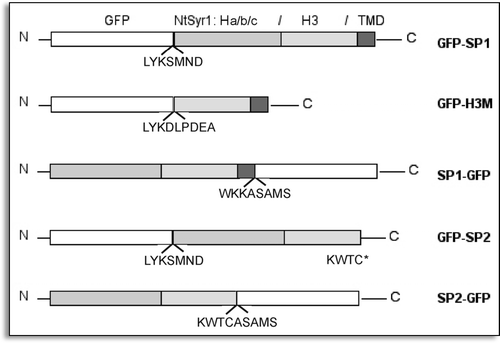
Figure 2 Distribution of GFP fusion proteins between soluble (S) and membrane (M) fractions from transiently transformed protoplasts. GFP fused with none or short fragments from NtSyr1 are labelled by anti-GFP antiserum immunostaining; GFP fused to full length NtSyr1 can be immunolabelled also by anti-NtSyr1 serum.
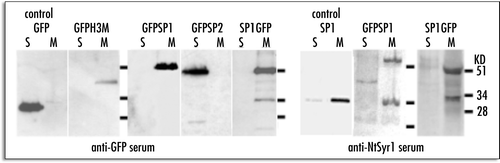
Figure 3 Representative fluorescent pattern of GFP fusion proteins. (A) GFP-SP1 labelled a defined ring analogous to PM proteins distribution; (B) PM typical pattern exhibited by PMA4GFP and used here as a control; (C) SP2-GFP pattern was cytosolic. (D) GFP-H3M early pattern; (E) GFP-H3M intermediate pattern with a not homogeneous distribution of fluorescence at the cell's periphery; (F) GFP-H3M late pattern, internal compartments are visible as rings (enlargement); (G) SP1-GFP early pattern; (H) SP1-GFP PM labelling common to the majority of cells; (I) SP1- GFP late pattern, internal compartments have a fluorescent lumen (enlargement). Scale bar = 20 µ.
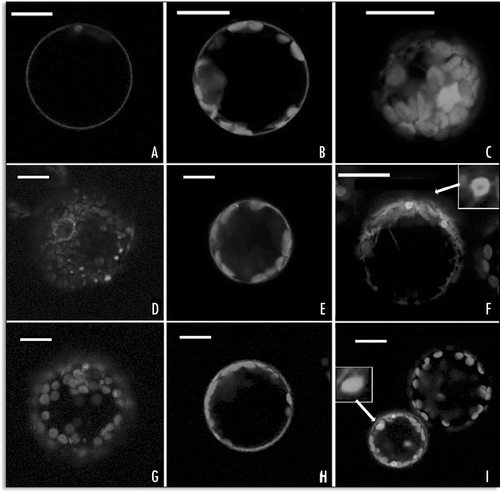
Figure 4 Fluorescent patterns altered by SP2 coexpression. (A) GFP-SP1 normal localization on the PM; (B) distribution of GFP-SP1 coexpressed with SP2 for 24 hours; (C) distribution of SP1-GFP coexpressed with SP2. Scale bar = 20 µ.
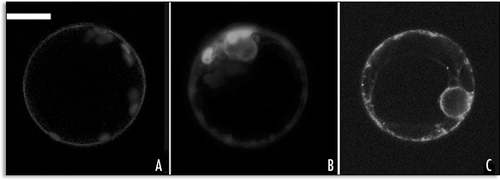
Figure 5 Graphic representation of time-dependent distribution of fluorescent patterns of chimeras: (A) percentage of cells, indicated as partial areas of the graphic, showing an E.P. (blue), a L.P. (yellow) or a PM (red) fluorescent pattern when SP1-GFP, GFP-H3M or GFP-SP1 were expressed; (B) graphic representation of internalization of GFP-H3M in different conditions: SP2 coexpression and ABA stimulation. It is reported the percentage of cells showing endocytotic fluorescent endosomes (L.P.).
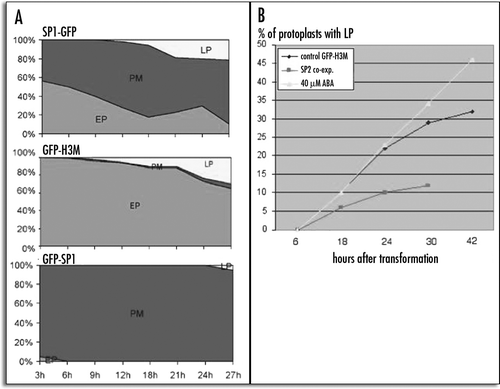
Figure 6 GFP-H3M fluorescence colocalised with FM4-64 staining: (A–D) protoplast expressing the GFP-H3M for 6 hours, (A) chlorophyll auto fluorescence, (B) FM4-64 fluorescence, (C) GFP fluorescence, (D) merge of the four channels; (E–H) protoplast expressing the GFP-H3M for 24 hours, (E) chlorophyll auto fluorescence, (F) FM4-64 fluorescence, (G) GFP fluorescence, (H) merge of the four channels. Scale bar = 20µ.
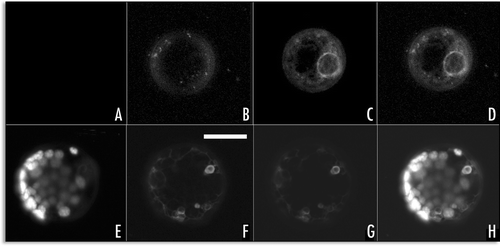
Figure 7 SP1-GFP fluorescence colocalised with FM4-64 staining: (A–D) protoplast expressing the SP1-GFP for 6 hours, (A) chlorophyll auto fluorescence, (B) FM4-64 fluorescence, (C) GFP fluorescence, (D) merge of the four channels; (E–H) protoplast expressing the SP1-GFP for 24 hours, (E) chlorophyll auto fluorescence, (F) FM4-64 fluorescence, (G) GFP fluorescence, (H) merge of the four channels. Scale bar = 20µ.
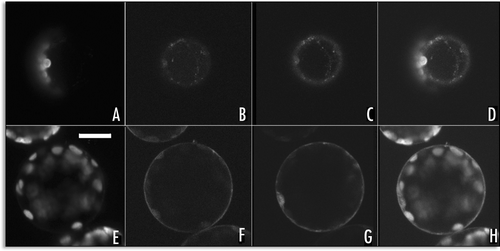
Figure 8 Representative fluorescent pattern of SP1-GFP variants. (A) SP1-GFPKDEL remained distributed within the ER; (B) SP1-GFPChi pattern was similar to SP1-GFP with no signal, peripheral ER and small compartments were labelled; (C) SP1-AleuGFP was mostly visible in small compartments similar to prevacuoles. Scale bar = 20 µ.
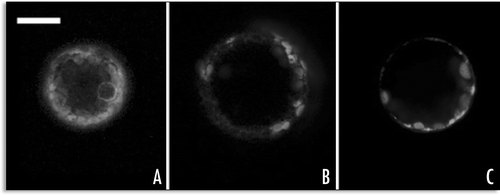
Figure 9 Agroinfiltration of N. tabacum leaves with GFP-SP1, GFP-H3M and GFP. (A) 48 hours after transient transformation with GFP-H3M, leaf tissue was unable to maintain turgor; (B) epidermal cell accumulating GFP-SP1 on the PM and in some internal areas; (C) when cytosolic GFP is accumulated, fluorescence appears uniformly distributed in cytosol and nucleus; (D) epidermal cell accumulating GFP-H3M in the ER (the nuclear membrane is labelled) and associated to small compartments; (E) GFP-H3M distributed in the cell's periphery probably associated to the PM (no ER labelling is clearly visible); (F) GFP-H3M accumulated in small endosomes-like spherical bodies with a non-fluorescent lumen; all fluorescent images are confocal projection of 6–8 m, scale bar = 20 µ.
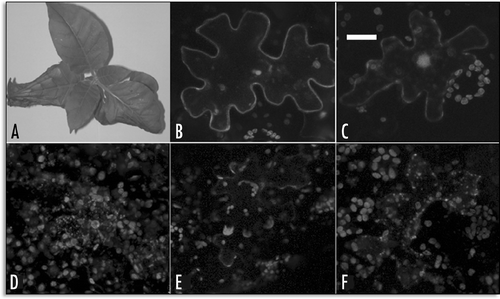
Table 1 Efficiency of secRGUS secretion when co-expressed with other constructs
Acknowledgements
A special thank to Professor M.R. Blatt for the gift of NtSyr1 cDNA and NtSyr1 anti-serum. Financial support for this work was in part (40%) due to FP6 EU project funding MTKD-CT-2004-509253.
References
- Leyman B, Geelen D, Quintero FJ, Blatt M. A tobacco syntaxin with a role in hormonal control of guard cell ion channels. Science 1999; 283:537 - 540
- Leyman B, Geelen D, Blatt M. Localization and control of expression of NtSyr1, a tobacco SNARE protein. Plant J 2000; 24:369 - 381
- Wu MN, Fergestad T, Lloyd TE, He Y, Broadie K, Bellen HJ. Syntaxin 1A interacts with multiple exocytic proteins to regulate neurotransmitter release in vivo. Neuron 1999; 23:593 - 605
- Geelen D, Leyman B, Batoko H, Di Sansabastiano GP, Moore I, Blatt MR. The abscisic acid-related SNARE homolog NtSyr1 contributes to secretion and growth: Evidence from competition with its cytosolic domain. Plant Cell 2002; 14:387 - 406
- Homann U, Tester M. Ca2+-independent and Ca2+/GTP-binding protein-controlled exocytosis in a plant cell. Proc Natl Acad Sci USA 1997; 94:6565 - 6570
- Borgese N, Colombo S, Pedrazzini E. The tale of tail-anchored proteins: Coming from the cytosol and looking for a membrane. J Cell Biol 2003; 161:1013 - 1018
- Kutay U, Ahnert-Hilgen G, Hartmann E, Wiedenmann B, Rapoport TA. Transport route for synaptobrevin via a novel pathway of insertion into the endoplasmic reticulum membrane. EMBO J 1995; 14:224 - 231
- Pedrazzini E, Villa A, Longhi R, Bulbarelli A, Borgese N. Mechanism of residence of cytochrome b(5), a tail-anchored protein, in the endoplasmic reticulum. J Cell Biol 2000; 148:899 - 914
- Di Sansebastiano GP, Paris N, Marc-Martin S, Neuhaus JM. Regeneration of a lytic central vacuole and of neutral peripheral vacuoles can be visualized by green fluorescent proteins targeted to either type of vacuoles. Plant Physiol 2001; 126:78 - 86
- Di Sansebastiano GP, Paris N, Marc-Martin S, Neuhaus JM. Specific accumulation of GFP in a nonacidic vacuolar compartment via a C-terminal propeptide-mediated sorting pathway. Plant J 1998; 15:449 - 457
- Frisch DA, Harris-Haller LW, Yokubaitis NT, Thomas TL, Hardin SH, Hall TC. Complete sequence of the binary vector Bin 19. Plant Mol Biol 1995; 27:405 - 409
- Haseloff J, Siemering KR, Prasher DC, Hodge S. Removal of a cryptic intron and subcellular localization of green fluorescent protein are required to mark transgenic Arabidopsis plants brightly. Proc Natl Acad Sci USA 1997; 94:2122 - 2127
- Fluckiger R, De Caroli M, Piro G, Dalessandro G, Neuhaus JM, Di Sansebastiano GP. Vacuolar system distribution in Arabidopsis tissues, visualized using GFP fusion proteins. J Experimental Botany 2003; 54:1 - 8
- Maliga P, Lazar G, Svab Z, Nagy F. Transient cycloheximide resistance in a tobacco cell line. Mol Gen Genet 1976; 149:267 - 271
- Freydl E, Meins F Jr, Boller T, Neuhaus JM. Kinetics of prolyl hydroxylation, intracellular transport and C-terminal processing of the tobacco vacuolar chitinase. Planta 1995; 147:250 - 256
- Negrutiu I, Shillito RD, Potrykus I, Biasini G, Sala F. Hybrid genes in the analysis of transformation conditions. I. Setting up a simple method for direct gene transfer in plant protoplasts. Plant Mol Biol 1987; 8:363 - 373
- Di Sansebastiano GP, Renna L, Piro G, Dalessandro G. Stubborn GFPs in Nicotiana tabacum vacuoles. Plant Biosystems 2004; 138:37 - 42
- Laemmli UK, Favre M. Maturation of the head of bacteriophage T4. J Mol Biol 1972; 80:575 - 599
- Di Sansebastiano GP. Targeting of soluble proteins to two different vacuoles in plant cells 1999; Switzerland University of Neuchatel PhD thesis
- Powell PP, Kyle JW, Miller RD, Pantano J, Grubb JH, Sly WS. Rat liver beta-glucuronidase cDNA cloning, sequence comparisons and expression of chimeric protein in COS cells. Biochem J 1988; 250:547 - 555
- Lefebvre B, Batoko H, Duby G, Boutry M. Targeting of a Nicotiana plumbaginifolia H+-ATPase to the plasma membrane is not by default and requires cytosolic structural determinants. Plant Cell 2004; 16:1772 - 1789
- Lefebvre, et al. 2000;
- Geldner N, Anders N, Wolters H, Keicher J, Kornberger W, Muller P, Delbarre A, Ueda T, Nakano A, Jurgens G. The Arabidopsis GNOM ARF-GEF mediates endosomal recycling, auxin transport, and auxin-dependent plant growth. Cell 2003; 112:219 - 230
- Bolte S, Brown S, Satiat-Jeurnemaitre B. The N-myristolylated Rab-GTPase m-Rabmc is involved in post-Golgi trafficking events to the lytic vacuole in plant cells. J Cell Sci 2004; 117:943 - 954
- Zheng H, Camacho L, Wee E, Batoko H, Legen J, Leaver CJ, Malhó R, Hussey PJ, Moore I. A Rab-E GTPase mutant acts downstream of the Rab-D subclass in biosynthetic membrane traffic to the plasma membrane in tobacco leaf epidermis. Plant Cell 2005; 17:2020 - 2036
- Jantti J, Keranen S, Toikkanen J, Ehnholm C, Sodderlund H, Olkkonen VM. Membrane insertion and intracellular transport of yeast syntaxin Sso2p in mammalian cells. J Cell Sci 1994; 107:3623 - 3633
- Pedrazzini E, Villa A, Borgese N. A mutant cytochrome b5 with a lengthened membrane anchor escapes from the endoplasmic reticulum and reaches the plasma membrane. Proc Natl Acad Sci USA 1996; 93:4207 - 4212
- Bar-Peled M, Conceicao A, Frigerio L, Raikhel NV. Expression and regulation of aERD2, a gene encoding the KDEL receptor homolog in plants, and other genes encoding proteins involved in ER-Golgi vesicular trafficking. Plant Cell 1995; 7:667 - 676
- Paris N, Rogers SW, Jiang L, Kirsch T, Beevers L, Phillips TE, Rogers JC. Molecular cloning and further characterization of a probable plant vacuolar sorting receptor. Plant Physiol 1997; 115:29 - 39
- Joglekar AP, Xu D, Rigotti DJ, Fairman R, Hay JS. The SNARE motif contributes to rbet1 intracellular targeting and dynamics independently of SNARES interactions. J Biol Chem 2003; 278:14121 - 14133
- Kargul J, Gansel X, Tyrrell M, Sticher L, Blatt MR. Protein-binding partners of the tobacco syntaxin NtSyr1. FEBS Letters 2001; 508:253 - 258
- Rowe J, Corradi N, Malosio ML, Taverna E, Halban P, Meldolesi J, Rosa P. Blockade of membrane transport and disassembly of the Golgi complex by expression of syntaxin 1A in neurosecretion-independent cells: Prevention by rbSEC1. J Cell Sci 1999; 112:1865 - 1877
- Takuma T, Arakawa T, Okayama M, Mizoguchi I, Tanimura A, Tajima Y. Trafficking of green fluorescent protein-tagged SNARE proteins in HSY cells. J Biochem 2002; 132:729 - 735
- Uemura T, Ueda T, Ohniwa RL, Nakano A, Takeyasu K, Sato MH. Systematic analysis of SNARE molecules in Arabidopsis: Dissection of the post-Golgi network in plant cells. Cell Struct Funct 2004; 29:49 - 65
- Chieregatti E, Meldolesi J. Regulated exocytosis: New organelles for nonsecretory purposes. Nat Rev Mol Cell Biol 2005; 6:181 - 187