Abstract
Plants can be classified in two groups based on Iron (Fe) acquiring strategy. Graminaceous plants (Strategy II) acquire iron through mugineic acid family phytosiderophores (MAs). All MAs are synthesized from L-Met, sharing the same pathway from L-Met to 2'-deoxymugineic acid (DMA) and the subsequent steps may differ depending on plant specie and cultivar. DMA is synthesized through the reduction of a 3"-keto intermediate by deoxymugineic acid synthase (DMAS). Previously, all the genes involved in the synthesis of DMA have been cloned with the exception of DMAS. Recently we have reported the isolation of DMAS genes from rice, wheat, maize and barley. The DMAS belongs to aldo-keto reductase superfamily (AKR). The expression of each of the above DMAS genes is upregulated under Fe-deficient conditions in root tissue, and that of OsDMAS1 and TaDMAS1 are upregulated in shoot tissue. It seems that the expression of DMAS is not regulated at posttranscriptional level. Analysis of OsDMAS1 promoter-GUS transgenic rice suggested that DMA may have role in Fe homeostasis in rice. The cloning of DMAS genes is an important step to develop transgenic rice with increased biosynthesis and section of DMA and ultimately resistant to Fe-deficiency in calcareous soils.
Iron (Fe) is essential for various cellular events such as respiration and photosynthetic electron transport. Fe is also essential for the synthesis of heme and chlorophyll and low chlorophyll content (chlorosis) of young leaves is the most obvious visible symptom of Fe-deficiency. As Fe is sparingly soluble in neutral to alkaline soils,Citation1 plants have developed sophisticated and tightly regulated mechanisms to acquire Fe from the soil, which can be grouped into two strategies.Citation2 Graminaceous plants (Strategy II plants) solubilize soil Fe by secreting mugineic acid family phytosiderophores (MAs), from their roots.Citation3,Citation4 The resulting Fe(III)-MA complexes are then reabsorbed into the roots through a specific transporter. The production and secretion of MAs markedly increases in response to Fe-deficiency, and tolerance to Fe-deficiency is strongly correlated with the quantity and quality of the MAs secreted. For example, rice, wheat, and maize secrete only 2′-deoxymugineic acid (DMA) in relatively low amounts and are thus susceptible to low Fe availability. In contrast, barley secretes large amounts of many types of MAs, and is therefore more tolerant to low Fe availability.Citation5,Citation6
The biosynthetic pathway for MAs has been characterized.Citation7–Citation10 All MAs are synthesized from L-Met, sharing the same pathway from L-Met to DMA and the subsequent steps may differ depending on the plant specie and cultivar (). We adopted different strategies to clone DMAS. Finally, OsDMAS1 was identified as a member of aldo-keto reductase superfamily (AKR) and its orthologs from barley, wheat and maize were cloned.Citation11 With the isolation of graminaceous DMAS, all the genes of MAs biosynthetic pathway have been isolated, including S-adenosylmethionine synthetase (SAMS),Citation12 nicotianamine synthase (NAS),Citation13 nicotianamine aminotransferase (NAAT),Citation14 IDS2, and IDS3.Citation15–Citation16 Moreover, we have proved that 3″-keto acid is an intermediate in the pathway from NA to DMA.
The DMAS genes belong to AKR and have homology to Papaver somniferum codeinone reductases (AKR4B2-3) and Medicago sativa (AKR4A2) and Glycine max (AKR4A1) chalcone polyketide reductases (). AKR have 15 families (AKR1–AKR15)Citation17 and the criteria for classification of AKR is that members within a family have less than 40% amino acid sequence identity with other families and that members within a subfamily have greater than 60% sequence identity.Citation18 Based on this criterion, DMAS proteins do not fall in existing subfamilies of AKR4.Citation11 The sequence of DMAS was submitted to AKR database maintained at http://www.med.upenn.edu/akr/.Citation18 After introduction of conservative substitutions, the identity with existing members of AKR4B reached up to 65% and ZmDMAS1, OsDMAS1, HvDMAS1 and TaDMAS1 were assigned the numbers from AKR4B5 to AKR4B8 respectively. The substrate-binding domain was identified as described.Citation19 In rice, the putative substrate binding site was identified as A(50)-HY(52–53)-W(85)-HW(116–17)-V(119)-KA(127–28)-G(305)-Y(307)-S(309). The NADPH-binding domain is also conserved, which is a striking feature of the AKRs.Citation19 As members of AKR family are functionally redundant, there are chances that other members of AKR family may show the DMAS activity. The existence of multiple genes for NAS and NAAT in riceCitation20 and barleyCitation13–Citation14,Citation21 also supports this hypothesis. Although the closest paralog of OsDMAS1 did not show enzyme activity in our hands, the existence of more than one gene can not be ruled out.
An important question is the subcellular localization of DMAS. In silico analysis showed that DMAS may be localized to cytoplasm or microbody. On the other hand, MAs are thought to be synthesized in vesicles derived from rough ER.Citation22 Based on optimum pH (pH 9), NASCitation23 and NAATCitation24 are thought to be localized to these vesicles. The optimum pH for enzymatic activity of DMAS (pH 8–9) suggests that the enzyme localizes to some subcellular organelle(s) similar to NAS and NAAT where DMA is synthesized and stored until secretion.
Like all other genes involved in the MA biosynthetic pathway, DMAS genes are upregulated under Fe-deficient conditions in root tissue. Comparison of Northern and Western blot analysis revealed that DMAS is not regulated posttranscriptionally. DMA is detected in Fe-sufficient shoot in rice, which is possibly translocated from roots in a complex with Fe.Citation25 This hypothesis is strengthened by the fact that under Fe-sufficient conditions, OsDMAS1 expression was only observed in the portions of roots that are involved in long-distance transport. Under Fe-deficient conditions, the amount of DMA increases in rice shoots, and the expression of DMAS suggest that DMA is at least partially synthesized in shoot tissue. This DMA is thought to be involved in Fe homeostasis and does not participate in the acquisition of Fe from the soil.
Alkaline soils cover approximately 30% of world's arable land and rice grown on such soils is prone to Fe-deficiency. Our laboratory is engaged in the development of transgenic rice plants that are tolerant of alkaline soils with low Fe availability. Previously a genomic fragment containing two NAAT genes from barley were introduced in rice and the resulting transformants produced four times more grains as compared with control, although the production of DMA was only 1.8 times higher than control.Citation26 The introduction of HvDMAS1, alone or in combination with NAS, NAAT, IDS2 and IDS3, into rice would be a good strategy to develop transgenic rice plants tolerant to Fe-deficiency in calcareous soils. On the other hand the plants lacking or over expressing DMAS will reveal the role of DMA in iron homeostasis.
Abbreviations
AKR | = | Aldo-Keto reductase superfamily |
DMA | = | 2′-deoxymugineic acid |
Fe | = | iron |
MAs | = | mugineic acid family phytosiderophores |
Figures and Tables
Figure 1 Biosynthetic pathway of mugineic acid family phytosiderophores. Three molecules of S-adenosyl methionine are combined by NA synthase (NAS) to form nicotianamine (NA). The amino group of NA is transferred by NA aminotransferase (NAAT), and the resultant 3″-keto intermediate is reduced to 2′-deoxymugineic acid (DMA) by deoxymugineic acid synthase (DMAS). The subsequent steps differ with the plant species and cultivar.
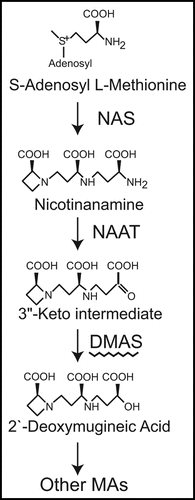
Figure 2 Phylogenetic characterization of DMAS genes. Unrooted phylo-genetic tree of the aldo-keto reductase superfamily (AKR4). The details and accession numbers of the AKR proteins are at www.med.upenn.edu/akr/members.html. AK102609 is a homolog of OsDMAS1 that lacks DMAS activity.
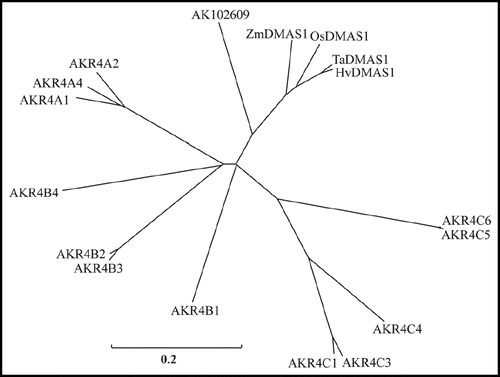
Acknowledgements
We are thankful to Dr. Takanori Kobayashi for critically reading the manuscript. K. Bashir is a Ph.D. student supported by ministry of Education, Culture, Sports, Science and Technology, Japan.
Addendum to:
References
- Marschner H. Mineral nutrition of higher plants 1995; New York Academic Press
- Marschner H, Römheld V, Kissel M. Different strategies in higher plants in mobilization and uptake of iron. J Plant Nutr 1986; 9:695 - 713
- Takagi S. Naturally occurring iron-chelating compounds in oat and rice root washing. I. Activity measurement and preliminary characterization. Soil Sci Plant Nutr 1976; 22:423 - 433
- Takagi S, Nomoto K, Takemoto S. Physiological aspect of mugineic acid, a possible phytosiderophore of graminaceous plants. J Plant Nutr 1984; 7:469 - 477
- Römheld V, Marschner H. Evidence for a specific uptake system for iron phytosiderophores in roots of grasses. Plant Physiol 1986; 70:175 - 180
- Singh K, Chino M, Nishizawa NK, Ohata T, Mori S. Randall RJ, Delhaize E, Richards RA, Munns R. Genetic aspects of plant mineral nutrition 1993; Kluwer Academic 335 - 339
- Mori S, Nishizawa NK. Methionine as a dominant precursor of phytosiderophores in Graminaceae plants. Plant Cell Physiol 1987; 28:1081 - 1092
- Ma JF, Shinada T, Matsuda T, Nomoto K. Biosynthesis of phytosiderophores, mugineic acids, associated with methionine cycling. J Biol Chem 1995; 270:16549 - 16554
- Kawai S, Takagi S, Sato Y. Mugineic acid-family phytosiderophores in root-secretions of barley, corn and sorghum varieties. J Plant Nutr 1988; 11:633 - 642
- Shojima S, Nishizawa NK, Fushiya S, Nozoe S, Irifune T, Mori S. Biosynthesis of phytosiderophores in vitro biosynthesis of 2′-deoxymugineic acid from L-methionine and nicotianamine. Plant Physiol 1990; 93:1497 - 1503
- Bashir K, Inoue H, Nagasak S, Takahashi M, Nakanishi H, Mori S, Nishizawa NK. Cloning and characterization of deoxymugineic acid synthase genes in graminaceous plants. J Biol Chem 2006; 281:32395 - 32402
- Takizawa R, Nishizawa NK, Nakanishi H, Mori S. Effect of iron deficiency on S-adenosylmethionine synthetase in barley roots. J Plant Nutr 1996; 19:1189 - 1200
- Higuchi K, Suzuki K, Nakanishi H, Yamaguchi H, Nishizawa NK, Mori S. Cloning of nicotianamine synthase genes involved in the biosynthesis of phytosiderophores. Plant Physiol 1999; 119:471 - 480
- Takahashi M, Yamaguchi H, Nakanishi H, Shioiri T, Nishizawa NK, Mori S. Cloning two genes for nicotianamine aminotransferase, a critical enzyme in iron acquisition (Strategy II) in graminaceous plants. Plant Physiol 1999; 121:947 - 956
- Okumura N, Nishizawa NK, Umehara Y, Ohata T, Nakanishi H, Yamaguchi T, Chino M, Mori S. A dioxygenase gene (Ids2) expressed under iron deficiency conditions in the roots of Hordeum vulgare. Plant Mol Biol 1994; 25:705 - 719
- Nakanishi H, Yamaguchi H, Sasakuma T, Nishizawa NK, Mori S. Two dioxygenase genes, Ids3 and Ids2, from Hordeum vulgare are involved in the biosynthesis of mugineic acid family phytosiderophores. Plant Mol Biol 2000; 44:199 - 207
- Yokochi N, Yoshikane Y, Trongpanich Y, Ohnishi K, Yagi T. Molecular cloning, expression, and properties of an unusual aldo-keto reductase family enzyme, pyridoxal 4-dehydrogenase, that catalyzes irreversible oxidation of pyridoxal. J Biol Chem 2004; 279:37373 - 37384
- Hyndman D, Bauman DR, Heredia VV, Penning TM. The aldo-keto reductase superfamily homepage. Chemico-Biol Interactions 2003; 143-144:621 - 631
- Jez JM, Bennett MJ, Schlegel BP, Lewis M, Penning TM. Comparative anatomy of the aldo-keto reductase superfamily. Biochem J 1997; 326:625 - 636
- Inoue H, Higuchi K, Takahashi M, Nakanishi H, Mori S, Nishizawa NK. Three rice nicotianamine synthase genes, OsNAS1, OsNAS2, and OsNAS3 are expressed in cells involved in long-distance transport of iron and differentially regulated by iron. Plant J 2003; 36:366 - 381
- Herbik A, Koch G, Mock HP, Dushkov D, Czihal A, Thielmann J, Stephan UW, Bäumlein H. Isolation, characterization and cDNA cloning of nicotianamine synthase from barley. Eur J Biochem 1999; 265:231 - 239
- Nishizawa N, Mori S. The particular vesicle appearing in barley root cells and its relation to mugineic acid secretion. J Plant Nutr 1987; 10:1012 - 1020
- Higuchi K, Nishizawa NK, Yamaguchi H, Römheld V, Marschner H, Mori S. Response of nicotianamine synthase activity to Fe-deficiency in tobacco plants as compared with barley. J Exp Bot 1995; 46:1061 - 1063
- Shojima S, Nishizawa NK, Fushiya S, Nozoe S, Irifune T, Mori S. In vitro biosynthesis of 2′-deoxymugineic acid from L-methionine and nicotianamine. Plant physiol 1990; 93:1497 - 1503
- Mori S, Nishizawa NK, Hayashi H, Chino M, Yoshimura E, Ishihara J. Why are young rice plants highly susceptible to iron deficiency?. Plant Soil 1991; 130:143 - 156
- Takahashi M, Nakanishi H, Kawasaki S, Nishizawa NK, Mori S. Enhanced tolerance of rice to low iron availability in alkaline soils using barley nicotianamine aminotransferase genes. Nat Biotechnol 2001; 19:466 - 469