Abstract
Phytochromes (PHYs) are photoreceptors of the red (R ~660 nm) and far-red (FR ~730 nm) light, and they control a wide range of responses affecting crucial aspects of plant life. There are five genes PHYA-PHYE encoding for phytochromes of different but overlapping function. One of these, PHYA has the unique function controlling specific responses in high irradiance far-red, as well as in very weak light. Appropriate PHYA functioning requires not only the photoreversibility of molecule but also the proper nuclear localization and degradation of receptor. Recently, we identified and described a mutant PHYA allele (phyA-5) in Arabidopsis thaliana, which showed reduced binding affinity to FHY1/FHL, the proteins regulating its nuclear transport, resulting in impaired nuclear localization and altered signaling under certain conditions. We present here a hypothesis to explain how the identified amino acid substitution may lead to structural changes manifested as altered signaling and phenotype displayed by the phyA-5 mutant.
A functional PHY molecule is a dimer of two ~120 kDa monomers, and each of the monomers contains the N-terminal photosensory and the C-terminal regulatory domains (see ). The N-terminal part is necessary for the photosensory function, and it contains the NTE, PAS, GAF and PHY domains. The GAF domain harbors the linear tetrapyrrol chromophore attached to a conserved cysteine residue via thioether bond.Citation1,Citation2 The C-terminal part, containing two PAS domains and a region similar to the histidine kinases (HKRD)Citation1 is essential for the nuclear translocation and dimerization of molecule.Citation3
Figure 1. Location and nature of the phyA-5 mutation. (A) Schematic arrangements of the phytochrome domains (not to scale). The position of phyA-5 mutation is indicated. NTE, N-terminal extension; PAS, PER/ARNT/SIM domain; GAF, cGMP phosphodiesterase, adenylate cyclase, FhlA domain; PHY, phytochrome domain; HKRD, histidine kinase-related domain; black rectangle represents the chromophore. (B) Backbone conformation of the predicted structure, based on the sequence including the residues 2050 for the PHYA protein. The helical segment is represented by yellow ribbon, while the other parts of molecule by blue ribbon. (C) Backbone conformation of the predicted structure, based on the sequence covering the residues 2050 for the PHYA-5 protein. The helical segment is represented by yellow ribbon, while the other parts of molecule by blue ribbon. (D) Predicted probabilities regarding the α-helical structure for the amino acids, on the basis of sequence including the residues 168 for the PHYA (blue line) and PHYA-5 (red line) proteins.
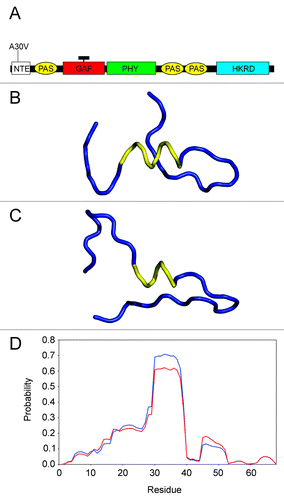
PHYs are synthesized in Pr form which can be converted by R photon absorption to the Pfr form which can be transformed back to Pr by FR photon absorption. The biologically active phyA Pfr conformer binds directly to nuclear import shuttle proteins, such as FHY1 (for FAR-RED ELONGATED HYPOCOTYL1) and FHL (for FHY1-LIKE)Citation4,Citation5 and it is transported to the nucleus. PHYA Pfr is ubiquitinated and undergoes rapid degradation.Citation6-Citation8 Recent results, supported with mathematical modeling prove that not only the unperturbed Pr/Pfr photoconversion but also the nuclear import and degradation of photoreceptor are required for the normal PHYA functioning. These events are tightly linked to each other like interlacing “cycles”.Citation9 Very recently, we identified and described a mutant (phyA-5) expressing PHYA with a missense mutation.Citation10 This mutant line shows a fairly complex phyA-specific phenotype, including a reduced nuclear import at low intensities of FR indicating that the point mutation identified alters the FHY1/FHL-phyA Pfr association/dissociation “cycle”. The fact that PHYA-5 governs wild-type-like signaling when it is fused to a nuclear localization signal (NLS), strongly supported this conclusion.Citation10
Our knowledge about the interaction of phyA with the FHY1 and FHL proteins is rather limited. Yeast two-hybrid assays showed that the Pfr form of the phyA406 (1–406 amino acids) specifically binds the FHY1/FHL transporter molecules.Citation5,Citation11 However, the exact three-dimensional (3D) structure of plant PHYs in contrast to microbial PHYs is not yet resolvedCitation12 and most of the published studies on microbial PHYs focused on the structural changes of Pr/Pfr conformation.Citation2,Citation12-Citation15 The phyA-5 mutation locates in the NTE domain, thus it is unlikely that it alters the structure of the chromophore bearing GAF domain (see ). Functional analyses of the NTE domain revealed that the serine residues located here are necessary for the PHY functionality.Citation16-Citation22 These studies focused on the possible function of the phosphorylation of serine residues, examining mutant PHYs with serine-to-alanine substitutions or with deletion of certain residues. Interestingly, the alanine-to-valine replacement in the phyA-5 mutant receptor results in a complex PHYA-specific phenotype,Citation10 despite the similar characteristics of these amino acids. To reveal the possible structural changes caused by this mutation, we performed protein structure prediction by the RaptorX program,Citation23 applying single- and multiple-template threading methods. For both PHYA and PHYA-5, we have chosen three sequences consisting of various numbers (from 30 to 140) of residues, in order to predict the 3D structural features of the N-terminal parts of proteins. Interestingly, the results indicate that the substitution of alanine by valine in position 30 results in shortening of the α-helical segments for all sequences, as it is demonstrated in the case of two representative structures in and . Nevertheless, based on the predicted distributions of different secondary structures calculated for each residue, an additional observation can be made, concerning the effects of alanine-to-valine replacement. The predicted probabilities with regard to the α-helical structure for the amino acids are presented in the case of two representative samples in . Considering these data, it can be seen that for the PHYA-5, the amino acids located in the region covering the residues 2838 can be characterized by a lower propensity to occupy the α-helical conformation, as compared with that found in the case of PHYA. These results suggest that the shorter α-helical segment, as well as the lower propensity to form this helical structure, in the NTE domain can disturb the binding of PHYA to FHY1/FHL. We note, that the data obtained by this modeling study make feasible to determine, by targeted and judicious substitution of amino acids located between 2838, to what extent this short region of the phyA N-terminal domain affects protein-protein interactions crucial for proper phyA signaling.
Acknowledgments
Work in Szeged, Hungary was supported by the Hungarian Scientific Research Fund [OTKA-81399] to A.V., and by the János Bolyai Research Scholarship of the Hungarian Academy of Sciences to B.L. Work in Freiburg, Germany was supported by an Sonderforschungsbereich [SFB 592] grant to E.S.
References
- Rockwell NC, Su YS, Lagarias JC. Phytochrome structure and signaling mechanisms. Annu Rev Plant Biol 2006; 57:837 - 58; http://dx.doi.org/10.1146/annurev.arplant.56.032604.144208; PMID: 16669784
- Ulijasz AT, Cornilescu G, Cornilescu CC, Zhang J, Rivera M, Markley JL, et al. Structural basis for the photoconversion of a phytochrome to the activated Pfr form. Nature 2010; 463:250 - 4; http://dx.doi.org/10.1038/nature08671; PMID: 20075921
- Nagatani A. Phytochrome: structural basis for its functions. Curr Opin Plant Biol 2010; 13:565 - 70; http://dx.doi.org/10.1016/j.pbi.2010.07.002; PMID: 20801708
- Hiltbrunner A, Viczín A, Bury E, Tscheuschler A, Kircher S, Tóth R, et al. Nuclear accumulation of the phytochrome A photoreceptor requires FHY1. Curr Biol 2005; 15:2125 - 30; http://dx.doi.org/10.1016/j.cub.2005.10.042; PMID: 16332538
- Hiltbrunner A, Tscheuschler A, Viczín A, Kunkel T, Kircher S, Schäfer E. FHY1 and FHL act together to mediate nuclear accumulation of the phytochrome A photoreceptor. Plant Cell Physiol 2006; 47:1023 - 34; http://dx.doi.org/10.1093/pcp/pcj087; PMID: 16861711
- Hennig L, Büche C, Eichenberg K, Schäfer E. Dynamic properties of endogenous phytochrome A in Arabidopsis seedlings. Plant Physiol 1999; 121:571 - 7; http://dx.doi.org/10.1104/pp.121.2.571; PMID: 10517849
- Seo HS, Watanabe E, Tokutomi S, Nagatani A, Chua NH. Photoreceptor ubiquitination by COP1 E3 ligase desensitizes phytochrome A signaling. Genes Dev 2004; 18:617 - 22; http://dx.doi.org/10.1101/gad.1187804; PMID: 15031264
- Saijo Y, Zhu D, Li J, Rubio V, Zhou Z, Shen Y, et al. Arabidopsis COP1/SPA1 complex and FHY1/FHY3 associate with distinct phosphorylated forms of phytochrome A in balancing light signaling. Mol Cell 2008; 31:607 - 13; http://dx.doi.org/10.1016/j.molcel.2008.08.003; PMID: 18722184
- Rausenberger J, Tscheuschler A, Nordmeier W, Wüst F, Timmer J, Schäfer E, et al. Photoconversion and nuclear trafficking cycles determine phytochrome A’s response profile to far-red light. Cell 2011; 146:813 - 25; http://dx.doi.org/10.1016/j.cell.2011.07.023; PMID: 21884939
- Sokolova V, Bindics J, Kircher S, Ádám E, Schäfer E, Nagy F, et al. Missense mutation in the amino terminus of phytochrome A disrupts the nuclear import of the photoreceptor. Plant Physiol 2012; 158:107 - 18; http://dx.doi.org/10.1104/pp.111.186288; PMID: 21969386
- Pfeiffer A, Kunkel T, Hiltbrunner A, Neuhaus G, Wolf I, Speth V, et al. A cell-free system for light-dependent nuclear import of phytochrome. Plant J 2009; 57:680 - 9; http://dx.doi.org/10.1111/j.1365-313X.2008.03721.x; PMID: 18980642
- Ulijasz AT, Vierstra RD. Phytochrome structure and photochemistry: recent advances toward a complete molecular picture. Curr Opin Plant Biol 2011; 14:498 - 506; http://dx.doi.org/10.1016/j.pbi.2011.06.002; PMID: 21733743
- Wagner JR, Brunzelle JS, Forest KT, Vierstra RD. A light-sensing knot revealed by the structure of the chromophore-binding domain of phytochrome. Nature 2005; 438:325 - 31; http://dx.doi.org/10.1038/nature04118; PMID: 16292304
- Yang X, Kuk J, Moffat K. Conformational differences between the Pfr and Pr states in Pseudomonas aeruginosa bacteriophytochrome. Proc Natl Acad Sci U S A 2009; 106:15639 - 44; http://dx.doi.org/10.1073/pnas.0902178106; PMID: 19720999
- Rockwell NC, Shang L, Martin SS, Lagarias JC. Distinct classes of red/far-red photochemistry within the phytochrome superfamily. Proc Natl Acad Sci U S A 2009; 106:6123 - 7; http://dx.doi.org/10.1073/pnas.0902370106; PMID: 19339496
- Cherry JR, Hondred D, Walker JM, Vierstra RD. Phytochrome requires the 6-kDa N-terminal domain for full biological activity. Proc Natl Acad Sci U S A 1992; 89:5039 - 43; http://dx.doi.org/10.1073/pnas.89.11.5039; PMID: 1594611
- Stockhaus J, Nagatani A, Halfter U, Kay S, Furuya M, Chua NH. Serine-to-alanine substitutions at the amino-terminal region of phytochrome A result in an increase in biological activity. Genes Dev 1992; 6:12A 2364 - 72; http://dx.doi.org/10.1101/gad.6.12a.2364; PMID: 1459458
- Lapko VN, Jiang XY, Smith DL, Song PS. Posttranslational modification of oat phytochrome A: phosphorylation of a specific serine in a multiple serine cluster. Biochemistry 1997; 36:10595 - 9; http://dx.doi.org/10.1021/bi970708z; PMID: 9265641
- Lapko VN, Jiang XY, Smith DL, Song PS. Mass spectrometric characterization of oat phytochrome A: isoforms and posttranslational modifications. Protein Sci 1999; 8:1032 - 44; http://dx.doi.org/10.1110/ps.8.5.1032; PMID: 10338014
- Casal JJ, Davis SJ, Kirchenbauer D, Viczian A, Yanovsky MJ, Clough RC, et al. The serine-rich N-terminal domain of oat phytochrome a helps regulate light responses and subnuclear localization of the photoreceptor. Plant Physiol 2002; 129:1127 - 37; http://dx.doi.org/10.1104/pp.010977; PMID: 12114567
- Trupkin SA, Debrieux D, Hiltbrunner A, Fankhauser C, Casal JJ. The serine-rich N-terminal region of Arabidopsis phytochrome A is required for protein stability. Plant Mol Biol 2007; 63:669 - 78; http://dx.doi.org/10.1007/s11103-006-9115-x; PMID: 17160561
- Han YJ, Kim HS, Kim YM, Shin AY, Lee SS, Bhoo SH, et al. Functional characterization of phytochrome autophosphorylation in plant light signaling. Plant Cell Physiol 2010; 51:596 - 609; http://dx.doi.org/10.1093/pcp/pcq025; PMID: 20203237
- Peng J, Xu J. RaptorX: exploiting structure information for protein alignment by statistical inference. Proteins 2011; 79:Suppl 10 161 - 71; http://dx.doi.org/10.1002/prot.23175; PMID: 21987485