Abstract
Chloroplasts and their surrounding cell are highly interdependent. One example is lipid metabolism, where the cell depends on its chloroplasts to provide fatty acids for lipid synthesis in the endoplasmic reticulum (ER) and in turn, chloroplasts rely on import of lipid precursors from the ER. Despite its fundamental importance, the route for lipid trafficking into and out of chloroplasts remains unknown. Biochemical studies of plant membrane lipid metabolism have suggested the possibility of lipid transport at membrane contact sites (MCSs) between the ER and chloroplasts. With the aid of optical manipulation, we recently could present physical evidence for this association. Leaf protoplasts isolated from Arabidopsis thaliana expressing green fluorescent protein (GFP) in the ER lumen were observed by confocal microscopy. A laser scalpel was used to rupture the protoplasts. ER fragments associated with the released chloroplasts could be stretched out by optical tweezers but remained attached to the chloroplast surface, even when a stretching force of 400 pN was applied. We thus provided the first physical evidence for MCSs between two membranes and we propose for the ER-chloroplast pair, that such tight associations are involved in bidirectional lipid trafficking between the two compartments.
Chloroplast-ER Associations
The spatial relationship between the ER and chloroplasts was studied by confocal microscopy, using transgenic Arabidopis thaliana stably expressing GFP in the ER lumen.Citation1 The ER formed a branched network in the cells and ER branch end-points appeared to locate at chloroplast surfaces. Corresponding images have been published previously,Citation2,Citation3 but actual physical connections between the ER and the chloroplast surface remained to be established. We then isolated chloroplasts from protoplasts obtained from transgenic A. thaliana leaves, using Percoll™ gradient centrifugation.Citation4 The majority of the isolated chloroplasts retained GFP-fluorescing bodies at their surface (), which demonstrates ER-chloroplast attachments strong enough to withstand the isolation procedure. We denoted the chloroplast-associated ER PLAM, for PLastid Associated Membranes, analogous to the terminology used for mitochondria- and plasma membrane-associated ER in yeast: MAMCitation5 and PAM,Citation6 respectively. We also presented the initial biochemical characterization of a PLAM fraction, obtained from isolated pea chloroplasts.Citation7
Optical Manipulation Reveal MCSs
To obtain information on the attachment between PLAM and chloroplasts, a confocal microscope equipped with a laser scalpel and optical tweezers was used for simultaneous imaging and optical manipulationCitation8 of leaf protoplasts and chloroplasts from transgenic A. thaliana. In the first set of experiments, one protoplast at a time was ruptured by a single pulse from the laser scalpel and a chloroplast was captured with the optical tweezers just after its release from the cell. When this chloroplast was pulled free of the other chloroplasts, a strand of GFP-fluorescent ER was stretched out between this and the other chloroplasts, demonstrating a strong attracting force between PLAM and chloroplasts. In the second experimental set-up, the PLAM attached to a chloroplast was captured by optical tweezers and stretched out. When the PLAM was released from the optical trap, it instantly relaxed back towards the chloroplast surface (see supplemental movies in ref. Citation7). We could never break the PLAM or pull it free of the chloroplast with the applied 400 pN optical force, a magnitude relevant for protein-protein interactions.Citation9 It should be noted that when the PLAM was stretched out, we never observed any deformation of the chloroplasts or any increase in the distance between the GFP-fluorescing PLAM and the red fluorescing chloroplasts, indicating that membrane stretching was restricted to the PLAM but that the outer chloroplast envelope remained physically intact. This observation strongly suggests some mechanism for the control and maintenance of chloroplast shape. The components involved remain to be identified, but it is known that a dynamin-related protein, localized in the perifery inside chloroplasts, affects thylakoid as well as chloroplast morphology.Citation10 Actin is known to interact with chloroplasts, but since it was recently shown that the association is lost during chloroplast isolation,Citation11 actin is probably not responsible for maintaining chloroplast shape in our experimental system.
Lipid Trafficking At MCSs?
Phospholipid transport to animal and yeast mitochondriaCitation5,Citation12 and transport of certain phospholipids to the plant plasma membraneCitation13 occurs directly from the site of synthesis, the ER, to the respective target membrane. The transport mechanisms are poorly understood, but MCSs are probably involved with specific proteins shuttling lipids between the closely associated membranes.Citation14 Our demonstration of zones of strong physical attachment between chloroplasts and a suggested specific region of the ER, the PLAM, fits with the well-established fact that chloroplasts import lipid precursors from the ER.Citation13,Citation15–Citation17 We proposed that at these MCSs, lipid trafficking occurs also in the opposite direction, of for example acyl-CoA and the galactolipid digalactosyldiacylglycerol (DGDG).Citation7
Fatty acids are exported from the chloroplast as water soluble acyl-CoAs, and measured rates of lipid synthesis in the ER suggest that efficient channelling of acyl-CoAs from chloroplasts to ER is needed.Citation18 Perhaps MCSs between PLAMs and chloroplasts provide the physical framework for this acyl-CoA transfer. The galactolipid DGDG could also be transported at such MCSs. During phosphate-limiting growth conditions, phospholipids are to a large extent replaced by DGDG.Citation19 Previously, this lipid was considered to be restricted to plastids, but phosphate deficiency has been reported to cause phospholipids-to-DGDG replacement in plasma membranes,Citation20,Citation21 mitochondriaCitation22 and tonoplasts.Citation21 This DGDG is synthesized in the outer plastid envelope.Citation23 The phospholipid replacement during phosphate deficiency thus reveals novel routes for lipid trafficking in plant cells.
The present working hypothesis is that DGDG transport to non-plastid membranes occurs via the ERCitation16 and we propose that the PLAM-chloroplast MCSs we have observed are involved. Further transport of DGDG from the ER to the plasma membrane or tonoplast could occur at MCSs between these membranes or as vesicles via the Golgi apparatus in the secretory pathway (see ). Electron microscopy has provided numerous images of chloroplasts closely aligned with the plasma membrane and the tonoplast. The recognition that specific lipids play crucial roles for membrane identification during membrane traffickingCitation24 would argue against a model of MCSs between chloroplasts and these membranes. For the cell to maintain internally recognizable membrane surfaces, all membranes are probably not able to exchange lipids directly with each other. Rather, a specific membrane, the ER, has a lipid sorting and transfer role.
Further StudieS of MCSs
Our report that optical manipulation could be used to demonstrate physical associations between an ER region and chloroplastsCitation7 opens up the possibility to answer other questions relating to the internal organization of the eukaryote cell: are there corresponding strong associations between regions of the ER and other membranes in plant cells and at the previously reported MCSsCitation14 in animal and yeast cells? Are there strong associations between chloroplasts and other membranes than the ER (plasma membrane, tonoplast, mitochondria)? The recent development of microfluidic sample chambers has introduced the possibility to manipulate the sample environment during the observation period under the microscope.Citation25 In combination with biochemical and molecular tools, it should thus be possible to identify and study the role of specific components for the associations at MCSs.
To end on a more speculative note: as the ER forms a network throughout the cytosol and is continuous with the nuclear envelope, are chloroplast-PLAM associations involved in the exchange of signals between the chloroplast and the surrounding cell?
Figures and Tables
Figure 1 A confocal microscopy image (Z-stack projection) of an isolated chloroplast from transgenic A. thaliana, expressing GFP in the ER lumen. Thylakoid (stack)s appear red and the associated PLAM appears green.
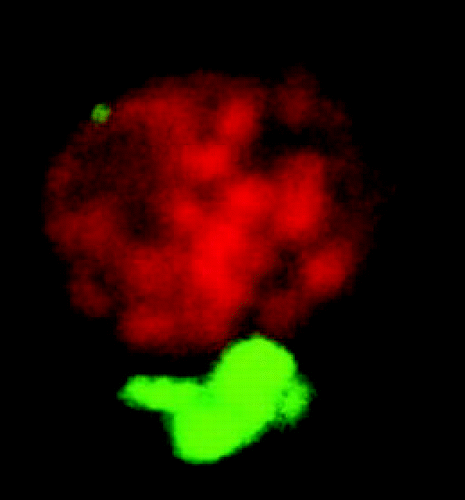
Figure 2 Possible routes for lipid trafficking from chloroplasts to plasma membrane, at MCSs in a plant cell. (A–D) denote proposed contact zones for DGDG trafficking between membranes, as described in the text: (A), chloroplast-ER (PLAM) contact; (B), plasma membrane-ER contact; (c), ER in contact with both chloroplast and plasma membrane; and (d), contact between chloroplast and plasma membrane. Other possible MCSs pictured are between the ER and mitochondria, trans-Golgi and tonoplast, respectively.

Addendum to:
References
- Mitsuhashi N, Shimada T, Mano S, Nishimura M, Hara-Nishimura I. Characterization of organelles in the vacuolar-sorting pathway by visualization with GFP in tobacco BY-2 cells. Plant Cell Physiol 2000; 41:993 - 1001
- Staehelin LA. The plant ER: A dynamic organelle composed of a large number of discrete functional domains. Plant J 1997; 11:1151 - 1165
- Hansson MR, Köhler RH. GFP imaging: Methodology and application to investigate cellular compartmentation in plants. J Exp Bot 2001; 52:529 - 539
- Räntfors M, Evertsson I, Kjellberg JM, Sandelius AS. Intraplastidial lipid trafficking: Regulation of galactolipid release from isolated chloroplast envelope. Phys Plant 2000; 110:262 - 270
- Gaigg B, Simbeni R, Hrastnik C, Paltauf F, Daum G. Characterization of a microsomal subfraction associated with mitochondria of the yeast, Saccharomyces cerevisiae: Involvement in synthesis and import of phospholipids into mitochondria. Biochim Biophys Acta 1995; 1234:214 - 220
- Pichler H, Gaigg B, Hrastnik C, Achleitner G, Kohlwein SD, Zellnig G, Perktold A, Daum G. A subfraction of the yeast endoplasmic reticulum associates with the plasma membrane and has a high capacity to synthesize lipids. Eur J Biochem 2001; 268:2351 - 2361
- Andersson MX, Goksör M, Sandelius AS. Optical manipulation reveals strong attracting forces at membrane contact sites between endoplasmic reticulum and chloroplasts. J Biol Chem 2007; 282:1170 - 1174
- Goksör M, Diez A, Enger J, Hanstorp D, Nystrom T. Analysis of molecular diffusion in ftsK cell-division mutants using laser surgery. EMBO Rep 2003; 4:867 - 871
- Florin EL, Moy VT, Gaub HE. Adhesion forces between individual ligand-receptor pairs. Science 1994; 264:415 - 417
- Gao H, Sage TL, Osteryoung KW. FZL, an FZO-like protein in plants, is a determinant of thylakoid and chloroplast morphology. Proc Nat Acad Sci USA 2006; 103:6750 - 6764
- Kumatani T, Sakurai-Ozato N, Miyawaki N, Yokota E, Shimmen T, Terashima I, Takagi S. Possible association of actin filaments with chloroplasts of spinach mesophyll cells in vivo and in vitro. Protoplasma 2006; 229:45 - 52
- Vance JE. Newly made phosphatidylserine and phosphatidylethanolamine are preferentially translocated between rat-liver mitochondria and endoplasmic-reticulum. J Biol Chem 1991; 266:89 - 97
- Moreau P, Bessoule JJ, Mongrand S, Testet E, Vincent P, Cassagne C. Lipid trafficking in plant cells. Prog Lipid Res 1998; 37:371 - 391
- Holthuis JCM, Levine TP. Lipid traffic: Floppy drives and a superhighway. Nature Rev 2005; 6:209 - 220
- Ohlrogge J, Browse J. Lipid biosynthesis. Plant Cell 1995; 7:957 - 970
- Kelly AA, Dörmann P. Green light for galactolipid trafficking. Curr Op Plant Biol 2004; 7:262 - 269
- Benning C, Xu C, Awai K. Non-vesicular and vesicular lipid trafficking involving plastids. Curr Opin Plant Biol 2006; 9:241 - 247
- Koo AJK, Ohlrogge JB, Pollard M. On the export of fatty acids from the chloroplast. J Biol Chem 2004; 279:16101 - 16110
- Härtel H, Dörmann P, Benning C. DGD1-independent biosynthesis of extraplastidic galactolipids after phosphate deprivation in Arabidopsis. Proc Nat Acad Sci USA 2000; 97:10649 - 10654
- Andersson MX, Stridh MH, Larsson KE, Liljenberg C, Sandelius AS. Phosphate-deficient oat replaces a major portion of the plasma membrane phospholipids with the galactolipid digalactosyldiacylglycerol. FEBS Lett 2003; 537:128 - 132
- Andersson MX, Larsson KE, Tjellstrom H, Liljenberg C, Sandelius AS. Phosphate-limited oat: The plasma membrane and the tonoplast as major targets for phospholipid-to-glycolipid replacement and stimulation of phospholipases in the plasma membrane. J Biol Chem 2005; 280:27578 - 27586
- Jouhet J, Maréchal E, Baldan B, Bligny R, Joyard J, Block MA. Phosphate deprivation induces lipid transfer of DGDG galactolipid from chloroplast to mitochondria. J Cell Biol 2004; 167:863 - 874
- Kelly AA, Froehlich JE, Dörmann P. Disruption of the two digalactosyldiacylglycerol synthase genes DGD1 and DGD2 in Arabidopsis reveals the existence of an additional enzyme for galactolipid synthesis. Plant Cell 2001; 15:2694 - 2706
- Behnia R, Munro S. Organelle identity and the signposts for membrane traffic. Nature 2005; 438:597 - 694
- Eriksson E, Enger J, Goksör M, Ramser K, Hanstorp D, Erjavec N, Nyström T, Hohmann S. A microfluidic system in combination with optical tweezers for analyzing rapid and reversible cytological alterations in single cells upon environmental changes. Lab Chip 2007; 7:71 - 76