Abstract
Signs of abiotic toxicity often appear first at the margins of leaves and gradually spread toward the midrib. It has been suggested that the bundle sheath tissue surrounding the shoot vascular system acts as a solute transport-regulating barrier that prevents excessive quantities of toxic ions from entering the leaf and pushes them toward the hydathodes. We examined this hypothesis by examining the distribution of toxic boron (B) in mutant Arabidopsis leaves with flooded mesophyll and comparing it with that observed in control leaves that exuded guttation drops. As opposed to the control plants, which showed classical symptoms of B toxicity (necrosis starting at the leaf margins), in the mutants, necrosis was first observed inside the leaf. We will discuss this result and how it supports the hypothesis that the bundle sheath serves as a selective barrier filtering the xylem-to-leaf radial transport flow and pushing toxic solutes toward the hydathodes.
Keywords: :
Regulation of the water balance of vascular plants is based on the counterbalancing of two systems: 1) stomatal gas conductance (gs) and 2) the radial hydraulic conductivity of the vascular system, which is entirely surrounded by the parenchymal envelope. The root endodermis is thought to be a selective barrier to the uptake of water, solutes and nutrients by the roots. The role of this tissue as a diffusion barrier has been demonstrated by observations of the transport of dyes, ions and heavy metals, all of which were prevented from entering the stele (reviewed in ref. Citation1). As for the selectivity of the endodermal plasma membrane (PM), localization studies have identified several transporters in the PM that face the cortex, endodermis or the stele.Citation2-Citation5 Despite the fact that the root endodermis acts to prevent excessive amounts of ions from entering the vascular system, some ions do pass through this filtration process and are carried with the transpiration stream to the aerial part of the plant.
The bundle sheath surrounds the vascular tissue in the shoot and is made up of dense parenchymal cells. The entire vascular system in the shoot is tightly enclosed by the bundle sheath, except for the ends of the vessel elements supplying the hydathodesCitation6 and the marginal vessels of the leaf blades of particular species, such as banana (Musa sp, Musaceae;Citation7). Therefore, the bundle sheath might function as the defense-regulator tissue for xylem sap coming out of the veins, to protect the sensitive, photosynthetic mesophyll tissue.
Similar to the root endodermis and in order to function as the xylem regulatory barrier, the bundle sheath should have the following two regulatory characteristics: (a) highly regulated apoplastic transport capacity and (b) cell membrane selectivity. Anatomical studies conducted in several plant species have revealed the presence of lipophilic components in the cell walls of the bundle sheath (reviewed in refs. Citation8, Citation9). This structure is similar to the endodermal Casparian strips in the root and acts as an apoplastic barrier against the xylem sap. An additional indication of the blocking of the apoplast is the guttation process. During the night, the presence of pressurized xylem sap leads to the release of water as guttation drops, which prevents this excess water from flooding the air spaces within the mesophyll. Thus, the radial apoplast pathway through the bundle sheath is negligible.
Bundle sheath cells have expressed unique solute transporters, such as siliconCitation10,Citation11 and K+ Na+ transporters.Citation7,Citation12 This suggests that the bundle sheath serves as ion-selective barrier surrounding the xylem. A paper recently published by our groupCitation12 described the hydraulic isolation of the bundle sheath and its role in converting chemical signal into hydraulic signal. In the presence of abiotic stress, the bundle sheath senses the increased concentration of ABA in the xylem sap. This leads to downregulation of aquaporin activity, which decreases the water permeability (Pf) of the cell membranes of bundle-sheath cells. This change in the permeability of the cell membranes is followed by a decrease in leaf water conductance and leaf water potential. In this manner, the bundle sheath functions as a hydraulic regulatory barrier to reduce the loss of water from the veins.
To estimate the extent of apoplastic isolation of the bundle sheath, we traced the distribution pattern of the microelement B. B is an essential microelement for plants, but there is only a narrow range between optimal and toxic levels. In vascular plants, B is transported from roots to shoots with the transpiration stream.Citation13 B is absorbed by the plant mainly as uncharged boric acid B(OH)3 and it is found in this form in the apoplast.Citation14 Boric acid is a small, uncharged molecule that can easily cross an artificial membrane designed to desalinate seawater.Citation15 Based on this observation, we would expect that it can also easily pass through the apoplastic barriers of the parenchymal bundle sheath cells.
Excessive levels of B in crops cause chlorosis that appears first at the tips and margins of mature leaves.Citation16,Citation17 Arabidopsis is a B-sensitive plant with a similar outside-in B-necrosis phenotype.Citation18 These symptoms suggest that B is able to cross the endodermal barrier, enter the vascular system and be carried by the transpiration stream to the leaves. Furthermore, we hypothesized that the selectivity of the bundle sheath prevents B from entering the leaf and pushes it toward the hydathodes, in an attempt to excrete the excessive B through the guttation drops. To find support for this hypothesis, we screened Arabidopsis WT population for leaves with impaired guttation (i.e., displaying flooded mesophyll instead of guttation drops). We found that about 3% of the screened leaves had flooded mesophyll (15 out of 540 leaves out of 54 plants). To examine the apoplastic movement through the bundle sheath, we first followed the distribution of Calcofluor White (CW) in control and mutant leaves. After 3 h, the CW spread through the veins of the control leaves that had excreted guttation drops () without leaking to the mesophyll (). In contrast, in the mutant leaves that had flooded mesophyll (), the CW leaked from the veins and spread through the mesophyll tissue (), suggesting damage to the apoplastic pathway of the bundle sheath.
Figure 1. Distribution of Calcofluor White (CW) and boron (B) toxicity in WT vs. mesophyll-flooded mutants. (A) WT leaf showing guttation picked just before dawn and immersed upon excision (petiole deep) in concentrated (1g*l−1) CW, an apoplastic fluorescent marker that stains the cellulose in cell walls. (B) The same leaf with CW fluorescence (380-nm excitation; 475-nm emission) 3 h after staining. (C) A closer look reveals that there was no staining outside the veins. (D) Mutant leaf in which mesophyll flooding symptoms were treated with CW, as mentioned above, revealing (E) CW leakage from the veins to the mesophyll tissue clearly visible as (F) CW staining in the area of the flooded mesophyll. (G) In WT Arabidopsis plants irrigated with 5 mM B for 14 d, necrosis first appeared at the leaf margins and then spread inward toward the middle of the leaf. Extraction of B from the leaf margins (M), the midrib (MR) and the intermediate zone (IM) revealed B concentrations that increased from the midrib to the margins; presented in table (H), values are the mean B concentration ± SE (mg/Kg dry tissue). This outside-in profile suggests the exclusion of B via predawn guttation and suggests tight apoplastic isolation of the bundle sheath. In addition, irrigating plants with 5 mM boron for 5 d caused (I) a mesophyll-flooded mutant to develop (J) necrosis at the leaf center (the flooded area). (K) The same leaf after 12 d of treatment. Pictures were taken of both the abaxial (I) and adaxial (J-K) sides of leaves to better present the differences in the patterns of flooding and necrosis in the different leaves. Bars in A-B, D-E, G, I-K = 0.5 cm; Bars in C, f = 0.5 mm.
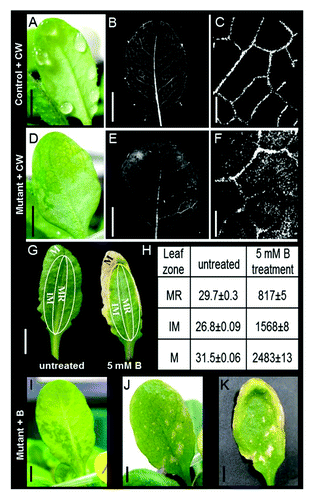
To follow the B distribution pattern, we performed a B irrigation experiment in which plants with mutant and controlled leaves were grown in soil and irrigated with 5 mM B. After 14 d of B treatment, necrosis gradually spread in the control leaves from the leaf margins inward (), in direct relation to B concentration in the leaves as measured using B extraction and ICP detection (i.e., from 817 mg/kg in the leaf central vein to 2483 mg/kg at the leaf margins) (). No necrosis was observed around the midrib despite the high B concentration. In contrast to the control leaves, the first signs of necrosis on the flooded leaves appeared as spots inside the leaf located at points corresponding to the flooded mesophyll ().
These results support our assumption of tightly controlled apoplastic permeability of the bundle sheath cells and the fact that the bundle sheath serves as a barrier, filtering the xylem-to-leaf radial transport of sap and conducting the unabsorbed solutes toward the hydathodes. Another interesting finding is the fact that the bundle sheath cells appear to be more tolerant of B than the mesophyll tissue. Bundle sheath cells have also been shown to tolerate higher concentrations of Na+ 7 and HgCl2.Citation12 It has been suggested that this tissue plays a role in refilling cavitated veins via their AQPsCitation19-Citation22 and is also involved in conducting water from the mesophyll to the xylem (the “novel refilling” theoryCitation23). These findings suggest that bundle sheath cells play an important role in plant resistance to abiotic stress.
Acknowledgments
This study was partially supported by grant #2010–320 from the Binational science foundation (BSF).
Disclosure of Potential Conflicts of Interest
No potential conflicts of interest were disclosed.
References
- Alassimone J, Roppolo D, Geldner N, Vermeer JE. The endodermis-development and differentiation of the plant's inner skin. Protoplasma 2012; 249:433 - 43; http://dx.doi.org/10.1007/s00709-011-0302-5; PMID: 21735349
- Alassimone J, Naseer S, Geldner N. A developmental framework for endodermal differentiation and polarity. Proc Natl Acad Sci USA 2010; 107:5214 - 9; http://dx.doi.org/10.1073/pnas.0910772107; PMID: 20142472
- Takano J, Tanaka M, Toyoda A, Miwa K, Kasai K, Fuji K, et al. Polar localization and degradation of Arabidopsis boron transporters through distinct trafficking pathways. Proc Natl Acad Sci USA 2010; 107:5220 - 5; http://dx.doi.org/10.1073/pnas.0910744107; PMID: 20194745
- Ma JF, Tamai K, Yamaji N, Mitani N, Konishi S, Katsuhara M, et al. A silicon transporter in rice. Nature 2006; 440:688 - 91; http://dx.doi.org/10.1038/nature04590; PMID: 16572174
- Ma JF, Yamaji N, Mitani N, Tamai K, Konishi S, Fujiwara T, et al. An efflux transporter of silicon in rice. Nature 2007; 448:209 - 12; http://dx.doi.org/10.1038/nature05964; PMID: 17625566
- Aloni R, Schwalm K, Langhans M, Ullrich CI. Gradual shifts in sites of free-auxin production during leaf-primordium development and their role in vascular differentiation and leaf morphogenesis in Arabidopsis. Planta 2003; 216:841 - 53; PMID: 12624772
- Shapira O, Khadka S, Israeli Y, Shani U, Schwartz A. Functional anatomy controls ion distribution in banana leaves: significance of Na+ seclusion at the leaf margins. Plant Cell Environ 2009; 32:476 - 85; http://dx.doi.org/10.1111/j.1365-3040.2009.01941.x; PMID: 19183293
- Sack L, Holbrook NM. Leaf hydraulics. Annu Rev Plant Biol 2006; 57:361 - 81; http://dx.doi.org/10.1146/annurev.arplant.56.032604.144141; PMID: 16669766
- Nardini A, Raimondo F, Lo Gullo MA, Salleo S. Leafminers help us understand leaf hydraulic design. Plant Cell Environ 2010; 33:1091 - 100; PMID: 20199625
- Yamaji N, Mitatni N, Ma JF. A transporter regulating silicon distribution in rice shoots. Plant Cell 2008; 20:1381 - 9; http://dx.doi.org/10.1105/tpc.108.059311; PMID: 18515498
- Yamaji N, Ma JF. A transporter at the node responsible for intervascular transfer of silicon in rice. Plant Cell 2009; 21:2878 - 83; http://dx.doi.org/10.1105/tpc.109.069831; PMID: 19734433
- Shapira O, Khadka S, Israeli Y, Shani U, Schwartz A. Functional anatomy controls ion distribution in banana leaves: significance of Na+ seclusion at the leaf margins. Plant Cell Environ 2009; 32:476 - 85; http://dx.doi.org/10.1111/j.1365-3040.2009.01941.x; PMID: 19183293
- Shatil-Cohen A, Attia Z, Moshelion M. Bundle-sheath cell regulation of xylem-mesophyll water transport via aquaporins under drought stress: a target of xylem-borne ABA?. Plant J 2011; 67:72 - 80; http://dx.doi.org/10.1111/j.1365-313X.2011.04576.x; PMID: 21401747
- Blevins DG, Lukaszewski KM. Boron in plant structure and function. Annu Rev Plant Physiol Plant Mol Biol 1998; 49:481 - 500; http://dx.doi.org/10.1146/annurev.arplant.49.1.481; PMID: 15012243
- Woods WG. Review of possible boron speciation relating to its essentiality. J Trace Elem Exp Med 1996; 9:153 - 63; http://dx.doi.org/10.1002/(SICI)1520-670X(1996)9:4<153::AID-JTRA3>3.0.CO;2-S
- Nadav N. Boron removal from seawater reverse osmosis permeate utilizing selective ion exchange resin. Desalination 1999; 124:131 - 5; http://dx.doi.org/10.1016/S0011-9164(99)00097-1
- Nable R, Banuelos G, Paull J. Boron toxicity. Plant Soil 1997; 193:181 - 98; http://dx.doi.org/10.1023/A:1004272227886
- Reid RJ, Fitzpatrick KL. Redistribution of boron in leaves reduces boron toxicity. Plant Signal Behav 2009; 4:1091 - 3; http://dx.doi.org/10.4161/psb.4.11.9798; PMID: 20009556
- Lamdan NL, Attia Z, Moran N, Moshelion M. The Arabidopsis-related halophyte Thellungiella halophila: boron tolerance via boron complexation with metabolites?. Plant Cell Environ 2012; 35:735 - 46; http://dx.doi.org/10.1111/j.1365-3040.2011.02447.x; PMID: 21999349
- Barrieu F, Chaumont F, Chrispeels MJ. High expression of the tonoplast aquaporin ZmTIP1 in epidermal and conducting tissues of maize. Plant Physiol 1998; 117:1153 - 63; http://dx.doi.org/10.1104/pp.117.4.1153; PMID: 9701571
- Holbrook NM, Zwieniecki MA. Embolism repair and xylem tension: Do We need a miracle?. Plant Physiol 1999; 120:7 - 10; http://dx.doi.org/10.1104/pp.120.1.7; PMID: 10318678
- Kaldenhoff R, Ribas-Carbo M, Sans JF, Lovisolo C, Heckwolf M, Uehlein N. Aquaporins and plant water balance. Plant Cell Environ 2008; 31:658 - 66; http://dx.doi.org/10.1111/j.1365-3040.2008.01792.x; PMID: 18266903
- Sakr S, Alves G, Morillon RL, Maurel K, Decourteix M, Guilliot A, et al. Plasma membrane aquaporins are involved in winter embolism recovery in walnut tree. Plant Physiol 2003; 133:630 - 41; http://dx.doi.org/10.1104/pp.103.027797; PMID: 14526109
- Hacke UG, Sperry JS. Limits to xylem refilling under negative pressure in Laurus nobilis and Acer negundo.. Plant Cell Environ 2003; 26:303 - 11; http://dx.doi.org/10.1046/j.1365-3040.2003.00962.x