Abstract
Regulator of G-protein signaling (RGS) proteins are a family of highly diverse, multifunctional proteins that function primarily as GTPase accelerating proteins (GAPs). RGS proteins increase the rate of GTP hydrolysis by Gα proteins and essentially regulate the duration of active signaling. Recently, we have identified two chimeric RGS proteins from soybean and reported their distinct GAP activities on individual Gα proteins. A single amino acid substitution (Alanine 357 to Valine) of RGS2 is responsible for differential GAP activity. Surprisingly, most monocot plant genomes do not encode for a RGS protein homolog. Here we discuss the soybean RGS proteins in the context of their evolution in plants, their relatedness to non-plant RGS protein homologs and the effect they might have on the heterotrimeric G-protein signaling mechanisms. We also provide experimental evidence to show that the interaction interface between plant RGS and Gα proteins is different from what is predicted based on mammalian models.
Heterotrimeric G-proteins are important signal tranducers in all eukaryotes. This protein complex consists of three dissimilar subunits: Gα, Gβ and Gγ. The Gα subunit is the enzymatically active protein of the complex which can exist in GTP-bound monomeric or GDP-bound trimeric conformation. In the classic paradigm of G-protein signaling, GDP-bound Gα remains associated with the non-dissociable βγ subunits and, in combination with a G-protein coupled receptor (GPCR), represents the inactive state of the signaling pathway. Signal perception by GPCR facilitates the exchange of GTP for bound GDP on Gα. The GTP-bound Gα dissociates from the Gβγ dimer and both entities can interact with specific downstream effectors to transduce the signal.Citation1-Citation3 Gα is restored to its GDP-bound conformation by its own intrinsic GTPase activity which leads to its reassociation with the Gβγ dimer and GPCR. This elegant switch-like signaling mechanism thus has two distinct regulatory steps: the rate of GDP-GTP exchange facilitated by a cognate GPCR, which involves GDP release and GTP binding; and the rate of GTP hydrolysis by the Gα protein.Citation3,Citation4
The signaling paradigm detailed in the previous section is mostly based on studies of metazoan systems in which heterotrimeric G-proteins have been characterized in great detail. The investigation of the mechanism(s) of plant G-protein signaling is only beginning but already studies are suggesting that even though the overall mechanism of this signaling pathway is conserved between plants and mammalian systems, important differences exist in the regulation of the G-protein cycle.Citation5-Citation7
One of the most well-characterized regulatory proteins of G-protein signaling complex is the “regulator of G-protein signaling” or RGS protein. RGS protein, also known as the GTPase-activating protein (GAP), accelerates the inherent GTPase activity of Gα protein, facilitating its return to the GDP-bound, inactive trimeric state. RGS proteins therefore can accelerate both signal onset and signal decay by promoting continuous G-protein cycling.Citation8
Plant RGS proteins are unique due to the presence of a 7-transmembrane domain at their N terminus which is reminiscent of a typical GPCR.Citation9,Citation10 None of the 37 different RGS proteins encoded by the human genome have a 7-transmembrane domain, although similar domains have been identified in many lower organisms.Citation11 Until recently, the RGS protein identified from Arabidopsis thaliana, AtRGS1, remained the sole representative of plant RGS proteins, but we have now identified and characterized two RGS proteins from the soybean genome, GmRGS1 and GmRGS2.Citation9,Citation10,Citation12 These proteins share a high degree of sequence similarity with AtRGS1 and have a similar chimeric domain structure with N-terminal GPCR-like domain and C-terminal RGS box. Similar to AtRGS1, the two GmRGS proteins act as GAPs, with different activities for each of the four Gα proteins of soybean. This differential activation adds a further level of complexity to the regulation of G-protein signaling in soybean and, by extension, to other plant species that have a larger repertoire of heterotrimeric G protein subunits.Citation10-Citation15 It is possible that each of the soybean Gα proteins exists in three different states of activity depending on cell type or signal: regulated by GmRGS1, GmRGS2 or non-RGS regulated. This multi-level regulation possibly provides greater biochemical diversity to the G-protein signaling in plants so as to compensate for their relative lack of quantitative diversity compared with metazoans.
Studies in Arabidopsis and soybean have proven that RGS proteins are physiologically important in plants and contribute to the signaling pathways regulated by different stimuli. The enigma, however, is the lack of RGS proteins in almost the entire monocot plant lineage. Setaria italica is the only monocot species known so far that encodes for a RGS protein homolog. This protein, however, lacks the 7-transmembrane domain found in all other plant RGS proteins. The Setaria italica genome also encodes for a canonical Gα protein-like sequence, although it has not been characterized to date. Interestingly, the club moss Selaginella moellendorffii has a homolog of the RGS gene in its genome, suggesting that the protein is lost during evolution in most monocots. No RGS protein homologs were detected in the genome of green algae Volvox carteri or Chlamydomonas reinhardtii which also does not contain a Gα protein; however, a RGS protein-like sequence was detected in the brown alga Ectocarpus siliculosus. While Phycsomitrella patens does contain a non-canonical Gα protein sequence in its genome, it does not encode a RGS protein. Due to the presence of RGS box containing sequences in many lower organisms in addition to the major animal and plant lineages, we performed an evolutionary relationship analysis of selected proteins representing various taxa. For the sake of simplicity, we have only included the representatives of the four conventional RGS protein families from human in this analysis: RGS2 from R4 family, RGS11 from R7 family, RGS12 from RGS12 family and RGS17 from RZ family (). The evolutionary study showed a clear distinction between the plant and non-plant sequences even when using only RGS box for this analysis. Similarly, the representative sequences from lower organisms also form a different clade than both plant and animal sequences.
Figure 1. Evolutionary relationship analysis of RGS proteins. Phylogenetic analysis of RGS proteins was performed with the RGS domain protein sequences from Glycine max (GmRGS, Glyma18 g01490.1 and GmRGS2, Glyma11g37540.1); Arabidopsis thaliana (AtRGS1, NP_189238); Ricinus communis (RcRGS, 29929.m004761); Manihot esculenta (MeRGS, cassava4.1_006961 min); Populus trichocarpa (PtRGS, POPTR_0008s18180.1); Citrus sinensis (CsRGS, Orange1.1g018201 min); Medicago truncatula (MtRGS, Medtr3g102890.1); Aquilegia coerulea (AcRGS, Aquca_056_00079.1); Vitis vinifera (VvRGS, GSVIVT01010292001); Setaria italica (SiRGS, Si031119 min); Mimulus guttatus (MgRGS, mgv1a005744 min); Selaginella moellendorffii (SmRGS, XP_002978651); Ectocarpus siliculosus (EsRGS, CBJ31534); Trichomonas vaginalis (TvRGS, XP_001311154); Saccharomyces cerevisiae (ScRGS2p, NP_014750 and Sst2p, NP_013557); Homo sapiens (HsRGS17, Q9UGC6; HsRGS2, CAG33691; HsRGS11, O94810 and HsRGS12, AAC39835); Brugia malayi (BmRGS, XP_001897289); Danio rerio (DrRGS, AAI54781); Xenopus tropicalis (XtRGS, NP_989295); Mus musculus (MmRGS, NP_075019); Rattus norvegicus (RnRGS, EDM09596); Anoplopoma fimbria (AfRGS, ACQ58658); Ascaris suum (AsRGS, ADY48881); Osmerus mordax (OmRGS, ACO09333); Ictalurus furcatus (IfRGS, ADO27836); Cricetulus griseus (CgRGS, EGW06162); Sus scrofa (SsRGS, NP_001230822); Acromyrmex echinatior (AeRGS, EGI69832); Camponotus floridanus (CfRGS, EFN71926) using the MEGA4.0 program (www.megasoftware.net/mega.html). The figure shows a non-rooted, bootstrap consensus plot, generated using the Neighbor-Joining method.
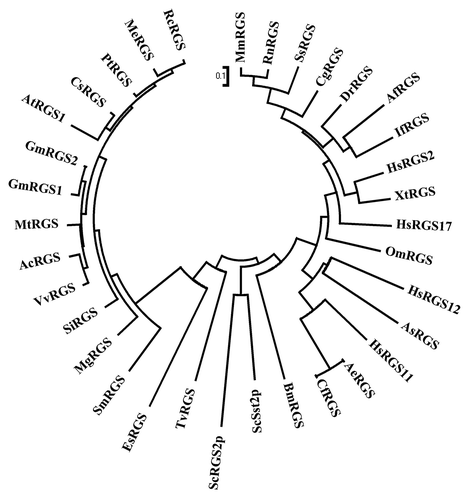
The physiological consequences of the absence of RGS protein homologs from the monocot lineage remain to be evaluated. It is not known whether the RGS protein from Setaria is an active GAP for the Setaria Gα protein or whether the presence of a Gα protein in an organism without the presence of its cognate regulatory protein suggests a completely different regulation of G-protein signaling. This different mechanism could explain the very different phenotypes for Gα mutant in rice, which is dwarf, and Arabidopsis, which is not.Citation16,Citation17 Biochemical characterization of RGS proteins from plants at different evolutionary timescales will help answer some of the questions related to their role in regulation of the plant G-protein cycle.
RGS proteins physically interact with Gα proteins to regulate their activity. The amino acids and domains involved in these interactions have been worked out in detail in the mammalian systems, and many Gα proteins have been crystallized with cognate RGS proteins that clearly decipher the interaction interface between them. Most of these amino acids are conserved in plant RGS proteins. However, the role of the unique 7-transmembrane domain in plant RGS-Gα interaction remained to be identified. We have shown that full-length RGS proteins interact with Gα proteins in soybean. Moreover, our data show that the amino acids predicted to be important for the GAP activity of mammalian RGS proteins based on their interaction interface with Gα proteins are also important for the GAP activity of soybean RGS proteins.Citation10,Citation12 However, in contrast to the mammalian systems, these amino acids do not seem to affect the interaction between the soybean RGS and Gα proteins. Yeast-based interaction assays using mating-based split-ubiquitin system show that not only WT-RGS proteins, but also the mutant versions of RGS proteins, E319Q, E319K and E319A, which have significantly compromised GAP activities, interact with Gα proteins with similar affinity (). These data are analogous to our results with the differences in activities of GmRGS1 and GmRGS2. The amino acids predicted to be involved in the interaction between RGS proteins and Gα proteins are identical in GmRGS1 and GmRGS2, but clear differences do exist between their activities as well as in their EC50 values which is an indirect measure of their interaction strength. Furthermore, the amino acid that we did identify as responsible for their differential activities (RGS1V357A; RGS2A357V) was not predicted to be a part of the interaction interface based on homology with mammalian models.Citation10 This suggests that novel amino acids and interaction interfaces might be involved in plant RGS-Gα protein interaction, distinct from what is known based on the mammalian systems. It is conceivable that such novel interactions would exist given the presence of the 7-transmembrane domain in plant RGS proteins as well as the unique structure of plant Gα proteins as deciphered by the recently solved crystal structure of AtGPA1. Comprehensive biochemical and biophysical characterization of RGS-Gα protein interaction will help discern the conserved as well as unique features of this signaling pathway in plants and may help explain the perplexing absence of RGS proteins in most monocot plants.
Figure 2. Interaction between wild-type (WT) and mutants (E319A, E319K, E319Q) GmRGS2 proteins (C-terminal RGS domain) with GmGα proteins using split-ubiquitin based interaction assay. The picture shows yeast growth on selective media with 1 mM methionine. In all cases RGS2 protein was used as CUb fusion (RGS2-CUb) and Gα proteins were used as NUb fusions in both orientations (NUb-Gα; N-terminal of ubiquitin fused at the N-terminal of Gα and Gα-NUb; N-terminal of ubiquitin fused at the C-terminal of Gα). NUbwt fusion constructs were used as positive controls for interaction and NUb-vector fusions were used as negative controls. Two biological replicates of the experiment were performed with identical results. The interaction combinations are: (1) RGS2-CUb+Gα1-NUb; (2) RGS2-CUb+Gα2-NUb; (3) RGS2-CUb+Gα3-NUb; (4) RGS2-CUb+Gα4-NUb; (5) RGS2-CUb+NUb-Gα1; (6) RGS2-CUb+NUb-Gα2; (7) RGS2-CUb+NUb-Gα3; (8) RGS2-CUb+NUb-Gα4; (9) RGS2-CUb+NUbwt-Gα3 (positive control). In A and B, (10) RGS2-CUb+Gα3-NUbwt (positive control); In C and D (10) RGS2-CUb+NUbwt vector (-ve control).
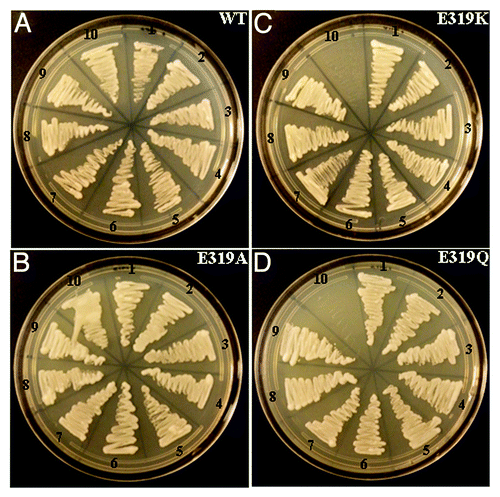
Disclosure of Conflict of Interest
No potential conflicts of interest were disclosed.
Acknowledgments
This work is supported by the Agriculture and Food Research Initiative Competitive Grants Program grant no. 2010–65116–20454 from the USDA National Institute of Food and Agriculture. C.S.W. was supported by a US. Department of Agriculture/Agriculture and Food Research Initiative pre-doctoral research fellowship (MOW-2010–05240).
References
- Gilman AG. G proteins: transducers of receptor-generated signals. Annu Rev Biochem 1987; 56:615 - 49; http://dx.doi.org/10.1146/annurev.bi.56.070187.003151; PMID: 3113327
- Cabrera-Vera TM, Vanhauwe J, Thomas TO, Medkova M, Preininger A, Mazzoni MR, et al. Insights into G protein structure, function, and regulation. Endocr Rev 2003; 24:765 - 81; http://dx.doi.org/10.1210/er.2000-0026; PMID: 14671004
- Offermanns S. G-proteins as transducers in transmembrane signalling. Prog Biophys Mol Biol 2003; 83:101 - 30; http://dx.doi.org/10.1016/S0079-6107(03)00052-X; PMID: 12865075
- McCudden CR, Hains MD, Kimple RJ, Siderovski DP, Willard FS. G-protein signaling: back to the future. Cell Mol Life Sci 2005; 62:551 - 77; http://dx.doi.org/10.1007/s00018-004-4462-3; PMID: 15747061
- Jones AM, Assmann SM. Plants: the latest model system for G-protein research. EMBO Rep 2004; 5:572 - 8; http://dx.doi.org/10.1038/sj.embor.7400174; PMID: 15170476
- Perfus-Barbeoch L, Jones AM, Assmann SM. Plant heterotrimeric G protein function: insights from Arabidopsis and rice mutants. Curr Opin Plant Biol 2004; 7:719 - 31; http://dx.doi.org/10.1016/j.pbi.2004.09.013; PMID: 15491922
- Chakravorty D, Trusov Y, Botella JR. Site-directed mutagenesis of the Arabidopsis heterotrimeric G protein β subunit suggests divergent mechanisms of effector activation between plant and animal G proteins. Planta 2012; 235:615 - 27; http://dx.doi.org/10.1007/s00425-011-1526-5; PMID: 22002625
- Siderovski DP, Willard FS. The GAPs, GEFs, and GDIs of heterotrimeric G-protein alpha subunits. Int J Biol Sci 2005; 1:51 - 66; http://dx.doi.org/10.7150/ijbs.1.51; PMID: 15951850
- Chen JG, Willard FS, Huang J, Liang J, Chasse SA, Jones AM, et al. A seven-transmembrane RGS protein that modulates plant cell proliferation. Science 2003; 301:1728 - 31; http://dx.doi.org/10.1126/science.1087790; PMID: 14500984
- Roy Choudhury S, Westfall CS, Laborde JP, Bisht NC, Jez JM, Pandey S. Two Chimeric Regulators of G-protein Signaling (RGS) Proteins Differentially Modulate Soybean Heterotrimeric G-protein Cycle. J Biol Chem 2012; 287:17870 - 81; http://dx.doi.org/10.1074/jbc.M112.353219; PMID: 22474294
- Anantharaman V, Abhiman S, de Souza RF, Aravind L. Comparative genomics uncovers novel structural and functional features of the heterotrimeric GTPase signaling system. Gene 2011; 475:63 - 78; http://dx.doi.org/10.1016/j.gene.2010.12.001; PMID: 21182906
- Jones JC, Temple BRS, Jones AM. DohlmanHG. Functional reconstitution of anatypical G protein heterotrimer and RGS protein from Arabidopsis thaliana. J Biol Chem 2011; 286:13143 - 50; http://dx.doi.org/10.1074/jbc.M110.190355; PMID: 21325279
- Bisht NC, Jez JM, Pandey S. An elaborate heterotrimeric G-protein family from soybean expands the diversity of plant G-protein networks. New Phytol 2010; 190:35 - 48; http://dx.doi.org/10.1111/j.1469-8137.2010.03581.x; PMID: 21175635
- Pandey S. More (G-proteins) please! Identification of an elaborate network of G-proteins in soybean. Plant Signal Behav 2011; 6:780 - 2; http://dx.doi.org/10.4161/psb.6.6.15145; PMID: 21543884
- Choudhury SR, Bisht NC, Thompson R, Todorov O, Pandey S. Conventional and novel Gγ protein families constitute the heterotrimeric G-protein signaling network in soybean. PLoS One 2011; 6:e23361; http://dx.doi.org/10.1371/journal.pone.0023361; PMID: 21853116
- Ueguchi-Tanaka M, Fujisawa Y, Kobayashi M, Ashikari M, Iwasaki Y, Kitano H, et al. Rice dwarf mutant d1, which is defective in the alpha subunit of the heterotrimeric G protein, affects gibberellin signal transduction. Proc Natl Acad Sci U S A 2000; 97:11638 - 43; http://dx.doi.org/10.1073/pnas.97.21.11638; PMID: 11027362
- Ullah H, Chen JG, Young JC, Im KH, Sussman MR, Jones AM. Modulation of cell proliferation by heterotrimeric G protein in Arabidopsis. Science 2001; 292:2066 - 9; http://dx.doi.org/10.1126/science.1059040; PMID: 11408654