Abstract
Reversible protein phosphorylation catalyzed by kinases and phosphatases is a major form of posttranslational regulation that plays a central role in regulating many signaling pathways. While large families of both protein kinases and protein phosphatases have been identified in plants, kinases outnumber phosphatases. This raises the question of how a relatively limited number of protein phosphatases can maintain protein phosphorylation homeostasis in a cell. Recent studies have shown that Arabidopsis FyPP1 (Phytochrome-associated serine/threonine protein phosphatase 1) and FyPP3 encode the catalytic subunits of protein phosphatase 6 (PP6), and that they directly binds to the A subunits of protein phosphatase 2A (PP2AA proteins), and SAL (SAPS domain-like) proteins to form the heterotrimeric PP6 holoenzyme complex. Emerging evidence is suggesting that PP6, acts in opposition with multiple classes of kinases, to regulate the phosphorylation status of diverse substrates and subsequently numerous developmental processes and responses to environmental stimuli.
Keywords: :
Protein phosphorylation and dephosphorylation mediated by kinases and phosphatases are major reversible post-translational modifications used to regulate many cellular processes. In eukaryotes, up to a third of proteins are regulated by protein phosphorylation. The genomes of eukaryotes encode a large number of kinases and phosphatases. For example, the human genome encodes at least 518 putative protein kinases that can be generally classified into two families: 90 protein tyrosine (Tyr) kinases (PTKs) and 428 protein serine/ threonine (Ser/Thr) kinases (PSKs).Citation1-Citation3 As the exquisite specificity of signaling and the reversible nature of phosphorylation, it would be expected that there are similar numbers of protein phosphatases in the human genome. Surprisingly, however, there are only 107 putative protein Tyr phosphatases (PTPs)Citation4 and fewer protein Ser/Thr phosphatases (PSPs) (~30) encoded by the human genome.Citation5 In Arabidopsis, there are more than 1000 kinases,Citation6 of which the PTK-like kinases are around 20 and the PSKs are most abundant.Citation7 However, the Arabidopsis genome only encodes 150 phosphatases, which can be classified into PSPs (125) and PTPs (25).Citation8 Whereas the numbers of PTKs and PTPs roughly match each other in both the human and plant genome, the number of catalytic subunits of PSPs is greatly lower than that of PSKs. This raises a question of how limited phoshatases, especially PSPs, can balance the phosphorylation activity in a cell. As will be discussed below, the recent advance in the holoenzyme assembly and functional information of PSPs could explain this dichotomy.
The PSPs can be divided into three major families: the large phosphoprotein phosphatase (PPP) family, the Mg2+ or Mn2+ dependent phosphatase PPM family and the Asp-based protein phosphatases.Citation9 The PPPs perform most phosphatase activities in eukaryotes.Citation10 Arabidopsis genome encodes 26 PPP catalytic subunits related to type 1 (PP1), type 2A (PP2A), and the so-called “novel phosphatases,” including PP4 (protein phosphatase 4), PP5, PP6 and PP7.Citation11 PP2B (also known as calcineurin), an important Ca2+-regulated PPP in other eukaryotes, is absent in plants,Citation12 while the PPKL (PP1 and kelch-like) PPP phosphatases found in plants are absent from mammals. The PPKL enzyme family, which is most close to the PP1 family, is defined by their large kelch repeat containing N-terminal extensions and C-terminal PP1-like phosphatase domain.Citation13 Some catalytic subunits of these PSPs, especially the protein serine/threonine phosphatases of PP1 and PP2A, can interact with a large variety of regulatory subunits and are therefore present in multiple holoenzymes.Citation14 For example, functional PP1 consists of a catalytic subunit (C) and a regulatory subunit (R). The catalytic domains of PPPs are highly conserved among all eukaryotes, thus support a conserved fold and similarly positioned active site for all members of the PPP family.Citation15 In human, there are more than 100 PP1-binding R subunits.Citation9 Thus combination of different C and R subunits will generate a large amount of PP1 holoenzymes, each of which could target certain substrates and have a distinct function in regulating cell development and signaling.
In addition, PP2A functions as a heterotrimer in cells. A conserved 65-kD A subunit containing 15 HEAT [Huntingtin, elongation factor 3 (EF3), protein phosphatase 2A (PP2A), and yeast PI3-kinase TOR1]-repeats which fold into a horseshoe-shaped scaffold that tethers the catalytic C subunit (PP2Ac) to form the PP2A dimer (PP2AD). An additional B-type regulatory subunit (PP2A B) associates with this PP2AD to form the PP2A holoenzyme complex, which determines the enzyme’s specific cellular localization and substrate specificity.Citation11 In Arabidopsis, there are 5 loci encode C subunits, 17 loci found to encode B subunits, whereas 3 loci code for A subunits.Citation11 Different combination of these subunits will generate at least 255 PP2A holoenzymes, each of which could have distinct function. PP2A, PP4 and PP6 are closely related based on high sequence identity among the catalytic subunits and considered to be PP2A-like phosphatases.Citation9 However, PP2A, PP4 and PP6 have diverse functions in vivo, as they are controlled by different regulatory subunits. Except that PP2A holoenzyme consists of different A, B and C subunits, PP4c binds to four unique proteins (PP4R1–4) and additional partners while PP6c binds to SAPS domain proteins (PP6R1, PP6R2 or PP6R3) and ankyrin repeat-containing proteins (ARS: Ankrd28, Ankrd44 and Ankrd52) to generate various PP4 and PP6 holoenzymes respectively and acquire their functional specificity.Citation15-Citation18 Thus considering the PSP holoenzymes composed of different regulatory subunits, the numbers of protein kinases and phosphatases could be more or less balanced.
In mammals, PP6 phosphatase holoenzymes have been identified (including a PP6c, a PP6R and an ARS protein) and found to regulate a broad range of signaling processes by targeting various substrates.Citation19-Citation21 In Arabidopsis, there are two loci encoding the catalytic subunits of PP6, FyPP1 (Phytochrome-associated serine/threonine protein phosphatase 1) and FyPP3.Citation11,Citation22 The FyPP1 and FyPP3 proteins are almost identical, with only three differences out of 303 amino acid residues.Citation23 We recently showed that in Arabidopsis (and perhaps in other plant species), the PP6 holoenzyme consists of a FyPP1 (or FyPP3) protein, a PP2A A protein (PP2AA1/RCN1, PP2AA2 or PP2AA3) and a SAPS domain-like protein (SAL1–4). Although PP2A A and SAL proteins are involved in the same PP6 holoenzyme assembly, FyPP1 interacts with both RCN1 and SAL1, and the N-terminal region of FyPP1 is required for these interactions. FyPP1 is necessary for the association of RCN1 and SAL1, indicating that FyPP1 (or FyPP3) functions as a bridge in forming the PP6 holoenzyme.Citation23 These data illustrate the differences between mammalian and plant PP6 holoenzymes in both their components and the way they are assembled.
Intriguingly, recent studies have begun to shed lights on the role of PP6 in regulating multiple signaling pathways in plant cells. Kim, et al.Citation22 showed that recombinant FyPP3 physically interacts with phytochromes (PhyA and PhyB) and they efficiently dephosphorylate auto-phosphorylated oat phytochrome A (PhyA) in a spectral form–dependent manner. Further, transgenic Arabidopsis plants with elevated or reduced FyPP levels exhibited delayed or accelerated flowering, respectively. Thus, PP6 phosphatase, coupled with the light-regulated kinase activities and autophosphorylation of phytochromes (PhyA and PhyB), represents a key regulatory mechanism in the photoperiodic control of flowering.Citation11,Citation22
More recently, we showed that PP6 also functions in auxin signaling. We showed that mutations simultaneously disrupting the function of Arabidopsis FyPP1 and FyPP3 result in severe defects in a wide range of developmental processes, including shorter roots, fewer lateral roots, defective collumella cells, root meristem collapse, abnormal cotyledons (small, cup-shaped or fused cotyledons), and altered leaf venation. The severe developmental defects resulted from increased basipetal auxin transport. Further analysis showed elevated accumulation of phosphorylated PIN-FORMED (PIN) proteins, the auxin efflux transporters, correlating with a basal-to-apical shift in subcellular PIN localization in the FyPP loss-of-function mutants. We also showed that PP2AA1, FyPP1 (or FyPP3), SAL1, the A, C and B subunits of PP6 holoenzyme respectively, function synergistically in regulating auxin transport and PIN de-phosphorylation and localization. Notably, although the single sal mutant (sal1–4) did not show obvious phenotype changes compared with wild type, the amiRNA transgenic lines with reduced expression of all four SAL genes displayed a phenotype resemble that of the fypp1 fypp3 double mutants, including shortened root and agravitropic root growth. These results indicate that there is function redundancy among the SAL genes and that the in vivo function of PP6 requires SALs’ function. Additionally, PP6 and PID/AGC3 kinase function antagonistically in regulating auxin transport and PIN phosphorylation and localization. These data indicate that the PP6 holoenzyme complex and PID/AGC3 kinases function as a molecular switch mediating auxin efflux through direct regulation of PIN phosphorylation in Arabidopsis.Citation23 In support of our notion, a recent report also showed that FyPP1 regulates PIN1 polar localization in Arabidopsis pavement cell development.Citation24
In addition, our preliminary analyses showed that the adults of FyPP1 and FyPP3 overexpression lines (F1OE and F3OE)Citation23 were dwarf (). The dwarf phenotypes of F1OE and F3OE plants may be an effect of altered auxin signaling, as auxin also plays roles in regulating plant height and shape.Citation25 Intriguingly, we also observed that the F1OE and F3OE adults showed early senescence phenotypes (). Consistently, the expression of the senescence-related marker genes, such as SAG12 and SAG13,Citation26 were upregulated in F1OE and F3OE leaves as compared with Col controls (), suggesting that PP6 also play a role in regulating plant aging. Perhaps PP6 might target the regulators in aging process. As hormones, such as abscisic acid (ABA) and ethylene, were believed to play critical roles in regulating plant programmed senescence,Citation27,Citation28 it is possible that PP6 could also mediate ABA or ethylene signaling, thus further regulate plant aging or other related development processes. A summary of the current understanding of the function of PP6 phosphatase is shown in . As kinases greatly outnumber phosphatases in plants,Citation8,Citation11 these studies also suggest that a single phosphatase may function in multiple signaling pathways in concert with multiple kinases, thus explaining in a certain way of how a limited number of phosphatases are maintaining protein phosphorylation homeostasis.
Figure 1. The phenotypes of F1OE and F3OE adult plants at 36-d old stage. F1OE (B, C) and F3OE (D, E) plants are dwarf as compared with Col controls (A). Statistical data of the plant height are shown in (K). Values are means ± SD (standard deviations), n = 20. F1OE (G, H) and F3OE (I, J) plants show early senescence as compared with Col controls (F). The senescence-related marker genes, such as SENESCENCE-ASOCIATE GENE 12 (SAG12, AT5G45890) and SAG13 (AT2G29350) were upregulated in F1OE and F3OE leaves (L). The RNA for RT-PCR was extracted from the leaves of 36-d old plants. The primers used in PCR are: SAG12-F 5′- TCATGCAAAAGAGGCACATC-3′, SAG12-R 5′- TATCCATTAAACCGCC TTCG-3′; SAG13-F 5′-CAACAATGTGGGAACGTCAA-3′, SAG13-R 5′- GCGGTGAGA CTTCATTTGCT-3′; Actin-F 5′-CACAATGTTTGGCGGGATTGGTGA-3′, Actin-R 5′-TGTACTTCCTTTCCGGTGGAGCAA-3′. The reverse transcription was performed as described.Citation23
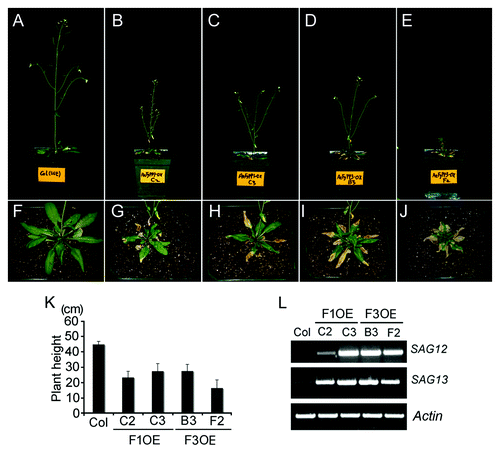
Figure 2. A model showing that PP6 functions as a molecular hub mediating multiple signaling pathways in plants. PP2AA and FyPP proteins interact together to form the PP6 phosphatase dimmer (PP6D). An additional regulatory subunit, SAL, interacts with PP6D to form the PP6 heterotrimeric holoenzyme. In auxin signaling, PP6 directly dephosphorylates the phosphorylated PIN proteins, while PID/AGC3 kinases are responsible to phosphorylate PIN. PP6 phosphatase and PID/AGC3 kinase thus keep the PINs’ phosphorylation homeostasis to direct auxin transport. In light signaling, PP6 directly targets autophosphorylated PhyA and PhyB to regulate light responses. PP6 may also functions antagonistically with unknown kinases to mediate the phosphorylation of some regulators in plant aging process. Question marks indicate unknown factors. p, phosphate.
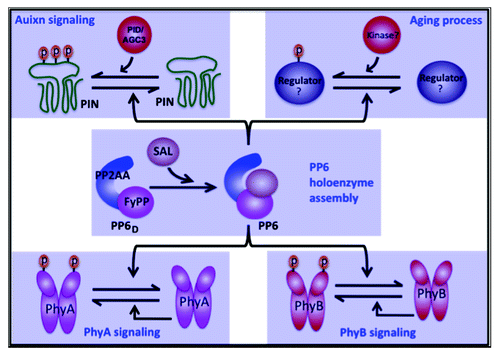
Our studies opened a gate to understand of how a plant PPP holoenzyme assembles and its broad function in regulating plant development. Further studies on PPPs need to be focused on how these phosphatases are regulated and more substrates of these PPPs. For example in PP6 case, as there are three A subunits (PP2AA1–3), four B subunits (SAL1–4) and two C subunits (FyPP1/3), a total of at least 24 hetertrimeric PP6 holoenzymes can be generated in Arabidopsis. Investigation of how a specific PPP holoenzyme composition is localized and function in different tissues and cellular compartments will undoubtedly unveil more insights of its function. In yeast, the SAPS domain proteins (B-type subunits of yeast PP6) were regulated by phosphorylation.Citation16 In addition, methylation and phosphorylation of the C-terminal tail of yeast and mammalian PP2Ac play critical roles in regulating PP2A activity and holoenzyme assembly.Citation29 As PP2A-like phosphatases (including PP2A, PP4 and PP6) have conserved C-terminal tails,Citation23 it is worthy to investigate how posttranslational modifications regulate the PPP activity and the regulators involved in these processes. Although ours and other’s recent studies shed light on PP6 novel mechanisms, many more regulatory interactions of PP6 phosphatase are probably still hidden. The main reason is that the regulatory interactions of PPPs are dynamic in vivo at different development stages and in response to various environmental stimuli. One way to investigate these interactions is to use system biology approaches to identify the PPP genome-wide interaction partners and targets during development and in response to environmental stimuli.
References
- Johnson SA, Hunter T. Kinomics: methods for deciphering the kinome. Nat Methods 2005; 2:17 - 25; http://dx.doi.org/10.1038/nmeth731; PMID: 15789031
- Lander ES, Linton LM, Birren B, Nusbaum C, Zody MC, Baldwin J, et al, International Human Genome Sequencing Consortium. Initial sequencing and analysis of the human genome. Nature 2001; 409:860 - 921; http://dx.doi.org/10.1038/35057062; PMID: 11237011
- Venter JC, Adams MD, Myers EW, Li PW, Mural RJ, Sutton GG, et al. The sequence of the human genome. Science 2001; 291:1304 - 51; http://dx.doi.org/10.1126/science.1058040; PMID: 11181995
- Alonso A, Sasin J, Bottini N, Friedberg I, Friedberg I, Osterman A, et al. Protein tyrosine phosphatases in the human genome. Cell 2004; 117:699 - 711; http://dx.doi.org/10.1016/j.cell.2004.05.018; PMID: 15186772
- Shi Y. Serine/threonine phosphatases: mechanism through structure. Cell 2009; 139:468 - 84; http://dx.doi.org/10.1016/j.cell.2009.10.006; PMID: 19879837
- Wang H, Chevalier D, Larue C, Ki Cho S, Walker JC. The Protein Phosphatases and Protein Kinases of Arabidopsis thaliana. Arabidopsis Book 2007; 5:e0106; PMID: 22303230
- Luan S. Tyrosine phosphorylation in plant cell signaling. Proc Natl Acad Sci U S A 2002; 99:11567 - 9; http://dx.doi.org/10.1073/pnas.182417599; PMID: 12195018
- Kerk D, Templeton G, Moorhead GB. Evolutionary radiation pattern of novel protein phosphatases revealed by analysis of protein data from the completely sequenced genomes of humans, green algae, and higher plants. Plant Physiol 2008; 146:351 - 67; http://dx.doi.org/10.1104/pp.107.111393; PMID: 18156295
- Moorhead GB, Trinkle-Mulcahy L, Ulke-Lemée A. Emerging roles of nuclear protein phosphatases. Nat Rev Mol Cell Biol 2007; 8:234 - 44; http://dx.doi.org/10.1038/nrm2126; PMID: 17318227
- Olsen JV, Blagoev B, Gnad F, Macek B, Kumar C, Mortensen P, et al. Global, in vivo, and site-specific phosphorylation dynamics in signaling networks. Cell 2006; 127:635 - 48; http://dx.doi.org/10.1016/j.cell.2006.09.026; PMID: 17081983
- Farkas I, Dombrádi V, Miskei M, Szabados L, Koncz C. Arabidopsis PPP family of serine/threonine phosphatases. Trends Plant Sci 2007; 12:169 - 76; http://dx.doi.org/10.1016/j.tplants.2007.03.003; PMID: 17368080
- Kerk D, Bulgrien J, Smith DW, Barsam B, Veretnik S, Gribskov M. The complement of protein phosphatase catalytic subunits encoded in the genome of Arabidopsis. Plant Physiol 2002; 129:908 - 25; http://dx.doi.org/10.1104/pp.004002; PMID: 12068129
- Moorhead GB, De Wever V, Templeton G, Kerk D. Evolution of protein phosphatases in plants and animals. Biochem J 2009; 417:401 - 9; http://dx.doi.org/10.1042/BJ20081986; PMID: 19099538
- Bollen M, Beullens M. Signaling by protein phosphatases in the nucleus. Trends Cell Biol 2002; 12:138 - 45; http://dx.doi.org/10.1016/S0962-8924(01)02247-4; PMID: 11859026
- Chen GI, Tisayakorn S, Jorgensen C, D’Ambrosio LM, Goudreault M, Gingras AC. PP4R4/KIAA1622 forms a novel stable cytosolic complex with phosphoprotein phosphatase 4. J Biol Chem 2008; 283:29273 - 84; http://dx.doi.org/10.1074/jbc.M803443200; PMID: 18715871
- Luke MM, Della Seta F, Di Como CJ, Sugimoto H, Kobayashi R, Arndt KT. The SAP, a new family of proteins, associate and function positively with the SIT4 phosphatase. Mol Cell Biol 1996; 16:2744 - 55; PMID: 8649382
- Stefansson B, Ohama T, Daugherty AE, Brautigan DL. Protein phosphatase 6 regulatory subunits composed of ankyrin repeat domains. Biochemistry 2008; 47:1442 - 51; http://dx.doi.org/10.1021/bi7022877; PMID: 18186651
- Slupe AM, Merrill RA, Strack S. Determinants for Substrate Specificity of Protein Phosphatase 2A. Enzyme Res 2011; 2011:398751; http://dx.doi.org/10.4061/2011/398751; PMID: 21755039
- Kajino T, Ren H, Iemura S, Natsume T, Stefansson B, Brautigan DL, et al. Protein phosphatase 6 down-regulates TAK1 kinase activation in the IL-1 signaling pathway. J Biol Chem 2006; 281:39891 - 6; http://dx.doi.org/10.1074/jbc.M608155200; PMID: 17079228
- Douglas P, Zhong J, Ye R, Moorhead GB, Xu X, Lees-Miller SP. Protein phosphatase 6 interacts with the DNA-dependent protein kinase catalytic subunit and dephosphorylates gamma-H2AX. Mol Cell Biol 2010; 30:1368 - 81; http://dx.doi.org/10.1128/MCB.00741-09; PMID: 20065038
- Zeng K, Bastos RN, Barr FA, Gruneberg U. Protein phosphatase 6 regulates mitotic spindle formation by controlling the T-loop phosphorylation state of Aurora A bound to its activator TPX2. J Cell Biol 2010; 191:1315 - 32; http://dx.doi.org/10.1083/jcb.201008106; PMID: 21187329
- Kim DH, Kang JG, Yang SS, Chung KS, Song PS, Park CM. A phytochrome-associated protein phosphatase 2A modulates light signals in flowering time control in Arabidopsis. Plant Cell 2002; 14:3043 - 56; http://dx.doi.org/10.1105/tpc.005306; PMID: 12468726
- Dai M, Zhang C, Kania U, Chen F, Xue Q, McCray T, et al. A PP6-type phosphatase holoenzyme directly regulates PIN phosphorylation and auxin efflux in Arabidopsis. Plant Cell 2012; 24:2497 - 514; http://dx.doi.org/10.1105/tpc.112.098905; PMID: 22715043
- Li H, Lin D, Dhonukshe P, Nagawa S, Chen D, Friml J, et al. Phosphorylation switch modulates the interdigitated pattern of PIN1 localization and cell expansion in Arabidopsis leaf epidermis. Cell Res 2011; 21:970 - 8; http://dx.doi.org/10.1038/cr.2011.49; PMID: 21423279
- Timpte C, Wilson AK, Estelle M. The axr2-1 mutation of Arabidopsis thaliana is a gain-of-function mutation that disrupts an early step in auxin response. Genetics 1994; 138:1239 - 49; PMID: 7896103
- Weaver LM, Gan S, Quirino B, Amasino RM. A comparison of the expression patterns of several senescence-associated genes in response to stress and hormone treatment. Plant Mol Biol 1998; 37:455 - 69; http://dx.doi.org/10.1023/A:1005934428906; PMID: 9617813
- Lee IC, Hong SW, Whang SS, Lim PO, Nam HG, Koo JC. Age-dependent action of an ABA-inducible receptor kinase, RPK1, as a positive regulator of senescence in Arabidopsis leaves. Plant Cell Physiol 2011; 52:651 - 62; http://dx.doi.org/10.1093/pcp/pcr026; PMID: 21382977
- Agarwal G, Choudhary D, Singh VP, Arora A. Role of ethylene receptors during senescence and ripening in horticultural crops. Plant Signal Behav 2012; 7:827 - 46; PMID: 22751331
- Janssens V, Longin S, Goris J. PP2A holoenzyme assembly: in cauda venenum (the sting is in the tail). Trends Biochem Sci 2008; 33:113 - 21; http://dx.doi.org/10.1016/j.tibs.2007.12.004; PMID: 18291659