Abstract
Plant mitochondria are proposed to act as signaling organelles in the orchestration of defense responses to biotic stress and acclimation responses to abiotic stress. However, the primary signal(s) being generated by mitochondria and then interpreted by the cell are largely unknown. Recently, we showed that mitochondria generate a sustained burst of superoxide (O2-) during particular plant-pathogen interactions. This O2- burst appears to be controlled by mitochondrial components that influence rates of O2- generation and scavenging within the organelle. The O2- burst appears to influence downstream processes such as the hypersensitive response, indicating that it could represent an important mitochondrial signal in support of plant stress responses. The findings generate many interesting questions regarding the upstream factors required to generate the O2- burst, the mitochondrial events that occur in support of and in parallel with this burst and the downstream events that respond to this burst.
Plant mitochondria play a central role in carbon and energy metabolism, but have also been suggested to generate “signals” that influence processes such as nuclear gene expression and resistance to biotic and abiotic stress.Citation1-Citation6 Certainly, mitochondria must be capable of retrograde regulation, whereby their function generates signals able to control the expression of nuclear genes encoding mitochondrial proteins.Citation7,Citation8 However, the primary signals responsible for these phenomenon remain largely unknown, and there is only a fragmented knowledge of any signal transduction pathways or cellular processes under mitochondrial influence.
Reactive oxygen species (ROS) and reactive nitrogen species (RNS) have become increasingly recognized as signal molecules involved in controlling a range of plant processes.Citation9-Citation12 The signaling function of these reactive molecules presumably requires specific mechanisms to control their synthesis, degradation and localization, all in response to particular conditions. Our knowledge of these mechanisms is generally quite limited, although substantial progress has been made in some plant systems.Citation13-Citation16
ROS and RNS Generation by Plant Mitochondria
Mitochondria are a source of ROS. This is due to “single electron leak” from electron transport chain (ETC) components to O2 producing superoxide (O2-). In both plants and animals, complexes I and III are proposed to be major sites of such electron leak.Citation17,Citation18 (). Once produced, matrix O2- can be further converted to H2O2 by a matrix-localized manganese superoxide dismutase (MnSOD).Citation19 The generation of RNS has also been linked to mitochondria although, in this case, the mechanism and site(s) of generation are less well understood, particularly in plants.Citation18,Citation20
Figure 1. The plant mitochondrial ETC includes two terminal oxidases able to catalyze the 4-electron reduction of O2 to H2O, the usual cyt oxidase (complex IV) and AOX. Electron transport from the ubiquinone pool (Q) to complex IV is coupled to the generation of a membrane potential that is subsequently dissipated by ATP synthase (complex V) to produce ATP. However, electron flow from Q to AOX is non-energy conserving. When the ability of an ETC component to transport electrons is reduced and/or membrane potential is high, electron transport can slow, leading to an over-reduction of the ETC. Under these conditions, single electron leak to O2 or nitrite increases, producing O2- and NO, respectively. In plants, the specific sites and mechanisms of O2- and NO generation are not yet well understood. See text for further details. I, II, III, IV, V: complexes I to V.
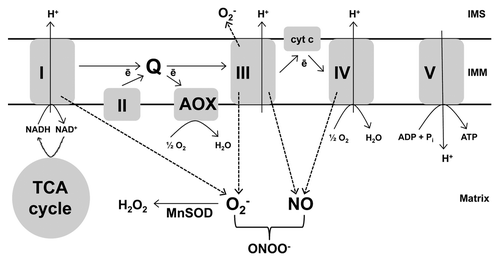
The rate of ROS generation by mitochondria depends upon the reduction state of ETC components. In animals, this reduction state is generally dependent upon the rate of electron transport and the membrane potential, which in turn are primarily dependent upon the rate of dissipation of membrane potential, particularly by oxidative phosphorylation. Hence, when ADP is readily available and being actively phosphorylated to ATP, dissipation of the proton gradient lowers membrane potential and O2- generation is less than when ADP is limiting. In plants, however, the relationship between electron transport, oxidative phosphorylation and ROS generation is more complex due to the presence of alternative oxidase (AOX), an additional ETC component (). AOX catalyzes the oxidation of ubiquinol and reduction of O2 to H2O, effectively acting as a branch in the ETC whereby electron flow bypasses proton-pumping complex III, cytochrome (cyt) c and proton-pumping complex IV (cyt oxidase).Citation21 Significantly, electron flow from ubiquinol to AOX is not proton-pumping and hence does not contribute to membrane potential (). Hence, AOX could provide a means to maintain electron flow while still preventing the over-reduction of the ETC.
Using fluorescent confocal microscopy, we recently showed that tobacco leaves with suppressed levels of AOX, due to RNA interference, have increased concentrations of O2- in their mitochondria.Citation22 This provides direct in planta evidence that one function of AOX is to prevent the over-reduction of ETC components that leads to single electron leak. Interestingly, the same study also found higher levels of nitric oxide (NO), a RNS, in the leaves lacking AOX and this appeared to be due, at least in part, to higher levels of NO in mitochondria. One means by which mitochondria may generate NO is via single electron leak from the ETC to nitrite.Citation18,Citation20 Hence our results suggest that AOX, by controlling the reduction state of the ETC, also dampens the generation of NO. Our conclusions were further supported by experiments with antimycin A (AA), a complex III inhibitor that, by restricting electron flow, causes an over-reduction of ETC components. In wild-type plants, both mitochondrial O2- and NO increased dramatically in response to AA. However, these increases were not seen in plants overexpressing AOX and hence able to maintain high rates of electron flow, even in the presence of AA.Citation23
Plant Mitochondria as Signaling Organelles During Biotic Stress
We hypothesized that plants may use mitochondrial-derived ROS and RNS as signaling molecules, and further that AOX could provide a means to modulate their generation, particularly during stress.Citation24 Below we describe recent evidence supporting this hypothesis.
It is well known that plant infection by a pathogen can lead to the rapid activation of a plasma membrane-localized NADPH oxidase.Citation10,Citation14 This activation results in an apoplastic “oxidative burst” that is closely associated with subsequent intracellular signaling events that culminate in defense responses such as the hypersensitive response (HR), a programmed cell death (PCD) at the site of infection.Citation25,Citation26 Beside this well-characterized apoplastic source of ROS, it is possible that intracellular sources of ROS (and RNS) are also important in orchestrating defense responses to pathogens. The mitochondrion is one such potential source that has been implicated.Citation27-Citation29
Recently, we examined the interaction of Nicotiana tabacum with the bacterial pathogen Pseudomonas syringae.Citation30 We showed that the incompatible P. syringae pv maculicola induced defense responses that included the HR, which was preceded by an early and persistent increase of O2- in the mitochondrial matrix (). This “O2- burst” was specific to the interaction with the HR-inducing pv maculicola, not being seen in response to a compatible (i.e., disease-causing) pv or in response to the incompatible pv phaseolicola that induced well-known defense responses but not including the HR. The disparate effect of the two incompatible pv’s appears to be due to a coordinated response of AOX (as a means to modulate the rate of O2- generation) and MnSOD (the sole enzymatic means to scavenge matrix O2-). While pv phaseolicola infection resulted in a strong induction of AOX and a maintenance of high MnSOD activity, pv maculicola infection failed to induce AOX and was accompanied by a loss of MnSOD activity.Citation30 (). We further established that, in transgenic AOX knockdown plants unable to induce AOX in response to pv phaseolicola, a O2- burst was now generated in response to infection.Citation23 Further, AOX knockdown plants infected with pv maculicola displayed a delayed O2- burst that manifested itself in a delayed HR. These results place AOX as a potential key regulator of a mitochondrial O2--based signaling pathway that subsequently impacts plant responses to biotic stress.Citation23,Citation30
Figure 2. The impact of two incompatible pv’s of P. syringae on the mitochondria of tobacco leaf mesophyll cells. (A) Infection with the HR-inducing pv maculicola results in an early and persistent burst of O2- in the mitochondrial matrix that may have a signaling role in support of the HR. (B) Infection with pv phaseolicola, that causes induction of plant defenses but not including the HR, lacks a matrix O2- burst. The differential effect of the two pv’s is supported by a coordinated response of the major ETC mechanism to avoid O2- generation (AOX) and the sole enzymatic means to scavenge matrix O2- (MnSOD). In response to pv phaseolicola, AOX is strongly induced and MnSOD activity remains high, while in response to pv maculicola MnSOD activity declines and AOX remains low. As a result, the two bacterial pv’s each generate distinct mitochondrial ROS signatures that may impact defense responses and cell fate.
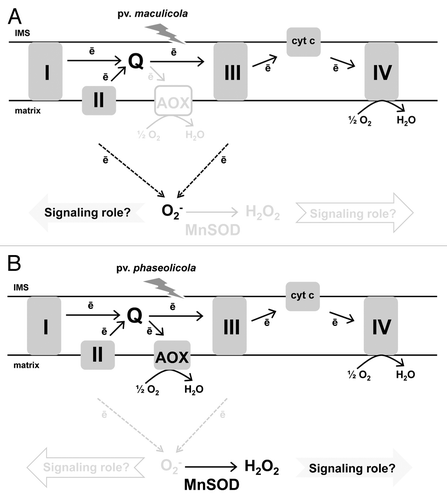
It is worth emphasizing that the O2- burst we have reported is clearly distinct from “mitochondrial O2- flashes” that have been detected, mostly in animals but also in plants.Citation31,Citation32 Those apparent flashes in O2- are short-term (seconds) in duration and have been detected using circularly permuted yellow fluorescent protein as the O2- sensor. Recently, it has been strongly argued that these O2- flashes are in fact an artifact of the detection system.Citation33 On the other hand, the O2- bursts we have reported are long-lived (hours) and have been measured using a mitochondrion-localizing version of hydroethidine (MitoSOX; Molecular Probes), a well-established small-molecule fluorescent O2- probe.Citation23,Citation30 As controls, we showed that the fluorescence signal observed with this probe could be strongly attenuated by the O2- scavenger SOD-PEG (a membrane-permeable SOD) or strongly amplified by AA.
Characterization and Significance of the Mitochondrial O2- Burst
Our resultsCitation23,Citation30 generate many interesting questions about mitochondrial signaling in general and the O2- burst, in particular. Some of these questions are summarized in and further discussed below.
Figure 3. Simple cartoon of a plant cell and mitochondrion. This figure is meant to highlight some key questions regarding the upstream factors required to generate a mitochondrial O2- burst, the mitochondrial events that occur in support of or in parallel with this burst, and the downstream events that may be responsive to this burst. See text for further discussion of these aspects. PM, plasma membrane; OMM, outer mitochondrial membrane; IMS, intermembrane space; IMM, inner mitochondrial membrane.
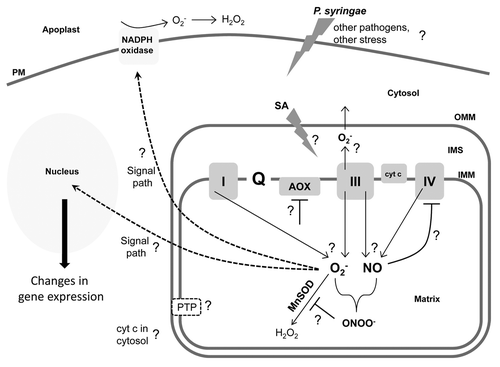
First, work to date has been done exclusively with P. syringae. Hence, it will be interesting to establish whether the HR induced by viruses and fungi is also preceded by a O2- burst. Changes in mitochondrial ROS have been implicated as important during such pathogen interactions.Citation3,Citation4,Citation34,Citation35 Further, AOX overexpression did result in smaller TMV-induced HR lesions.Citation36 However, definitive experiments to test for the appearance of a O2- burst in response to such pathogens are now needed.
If the mitochondrion has a signaling role during biotic stress, it would be interesting to establish whether this role extends to abiotic stresses as well. A common theme among several disparate mitochondrial mutants is their increased or decreased stress tolerance.Citation2,Citation4,Citation37,Citation38 Are specific mitochondrial ROS and/or RNS signatures responsible for this altered tolerance state? Disparate mitochondrial mutants also exhibit changes in stomatal aperture, particularly under stress conditions.Citation39-Citation41 An intriguing possibility is that changes in mitochondrial-derived ROS and/or RNS are impacting the signal path(s) that control stomatal movement.
Previous work shows that AOX can provide a level of protection against PCD.Citation24 Since the HR is a natural example of PCD that is likely of benefit to the plant, it is perhaps not surprising that AOX would be kept suppressed after pv maculicola infection. An interesting question is how this suppression is achieved since infection results in the rapid elevation of several molecules previously described as inducers of AOX synthesis such as salicylic acid (SA), NO and H2O2. We provided evidence that SA levels above a threshold amount might be responsible suppressing AOX induction, but this is clearly an area that requires further study. Whether AOX activity is also being suppressed by some post-translational mechanism is also relevant. In this regard, it is intriguing that AOX has been identified as a target of tyrosine nitration, albeit the study was not in relation to biotic stress and how the modification impacted AOX activity is not known.Citation42 Suppression of AOX activity could perhaps also occur by oxidation of regulatory cysteine thiolsCitation43 but again, whether this occurs in response to pathogen is not yet known.
As discussed earlier, membrane potential is typically a key factor determining the rate of O2-, and perhaps NO, generation by the ETC. A persistent O2- burst implies that membrane potential remains high and perhaps even increases after infection with the HR-inducing pv maculicola. A few studies have reported increases in membrane potential as an early event preceding various sorts of plant PCD, but more often it has been reported that loss of membrane potential is an early event.Citation44 Loss of membrane potential is sometimes attributed to the opening of a permeability transition pore (PTP), which in turn is often implicated to result in the release of cyt c to the cytosol. Unfortunately, the cause-effect relationship of these mitochondrial events, how they relate to O2- generation and how they impact cell fate is still largely unknown and is an important area for continued study.Citation44 One possible means to promote a high membrane potential, beside keeping AOX activity low, would be disabling of the adenine nucleotide translocator, which could restrict oxidative phosphorylation and hence dissipation of membrane potential.Citation45
While AOX knockdown plants have constitutive higher amounts of O2- than wild-type, this amount is nonetheless further strongly enhanced by the interaction with incompatible P. syringae indicating that, in addition to keeping AOX low, additional factor(s) associated with infection are required for the O2- burst to fully manifest itself. These factors are unknown but a prime candidate may be SA. Studies with plant suspension cells and isolated mitochondria strongly suggest that SA can target mitochondrial function.Citation46 However, its mechanism and site of action are not well understood, and the reported effects of SA on energy metabolism need further confirmation in intact leaf. Interestingly, our results hinted that complex III might be the mitochondrial target of biotic stress,Citation23 and studies with isolated mitochondria have implicated SA to impact the function of this complex.Citation47 The mode of action of SA on mitochondria may also differ depending upon SA concentration.Citation46 This provides a potential mechanism by which the different incompatible pv’s, which did elicit different SA amounts,Citation30 could have impacted mitochondria in disparate ways. Besides SA acting on the mitochondrion, another possibility is that increased NO inhibits cyt oxidase, which could then promote further generation of NO and O2- due to over-reduction of the ETC. NO is a potent inhibitor of cyt oxidase, but AOX is NO-resistant,Citation48 which further illustrates how AOX amount could strongly impact mitochondrial function during biotic stress.
Beside suppressing AOX induction, pv maculicola infection was associated with a loss of MnSOD activity, which likely supported the O2- burst by lessening the conversion of O2- to H2O2 in the matrix. The mechanism by which MnSOD activity is lost is not known. Interestingly, animal MnSOD is a major target of tyrosine nitration, resulting in a loss of activity.Citation49 Tyrosine nitration requires peroxynitrite (ONOO-), the product of the reaction of O2- and NO (). As MnSOD activity is lost, it promotes still higher O2-, which in turn promotes further ONOO- formation (if NO is available) and further MnSOD inactivation. It would be interesting to see whether a similar vicious circle is responsible for the loss of MnSOD after pv maculicola infection, which certainly was associated with increased levels of ONOO-.Citation23
Another important area for future research will be to establish the site(s) and topology of O2- generation by the ETC, particularly in relation to it acting as a signal molecule. For example, while complex I is thought to release O2- exclusively to the matrix side of the inner mitochondrial membrane (IMM), complex III may release O2- to both sides of the IMM (). Further studies should establish whether the matrix O2- burst measured by MitoSOX in our experiments was accompanied by additional O2- release to the inner membrane space (IMS).
Several studies have linked specific mitochondrial events with subsequent downstream changes in gene expression.Citation44 For example, Maxwell et al.Citation1 identified a group of typically biotic stress-responsive genes whose expression in response to mitochondrial dysfunction could be blocked by bongkrekic acid, an inhibitor of the PTP. Collectively, such studies provide a list of candidate genes that may be responsive to a mitochondrial O2- burst. Plants with modified AOX expression provide a means to manipulate the O2- burst and hence will be a useful tool to link the burst with specific gene expression profiles.
Another interesting area of study would be to establish the relationship between the mitochondrial O2- burst and the oxidative burst generated in the apoplast. As expected, we found that the HR-inducing pv maculicola generated a typical oxidative burst (measured as whole leaf H2O2) that was not seen with pv phaseolicola. However, when we elicited a mitochondrial O2- burst in pv phaseolicola using the AOX knockdown plants, this was now accompanied by a large and sustained increase in whole leaf H2O2.Citation23 While this could perhaps be explained by the mitochondrial O2- burst providing all the substrate for this H2O2 rise, another intriguing possibility is that the mitochondrial O2- burst lead to activation of NADPH oxidase. In animals, there are several model systems in which activation of NADPH oxidase depends upon intracellular ROS.Citation50 Interestingly, it is reported in tobacco that SA potentiates the oxidative burstCitation51 and that intracellular ROS rises prior to the oxidative burst.Citation29
Acknowledgments
G.C.V. is grateful to the Natural Sciences and Engineering Research Council of Canada (NSERC) and the University of Toronto Scarborough for their support of his research program on the alternative oxidase of plant mitochondria.
Disclosure of Potential Conflicts of Interest
No potential conflicts of interest were disclosed.
References
- Maxwell DP, Nickels R, McIntosh L. Evidence of mitochondrial involvement in the transduction of signals required for the induction of genes associated with pathogen attack and senescence. Plant J 2002; 29:269 - 79; http://dx.doi.org/10.1046/j.1365-313X.2002.01216.x; PMID: 11844105
- Dutilleul C, Garmier M, Noctor G, Mathieu C, Chétrit P, Foyer CH, et al. Leaf mitochondria modulate whole cell redox homeostasis, set antioxidant capacity, and determine stress resistance through altered signaling and diurnal regulation. Plant Cell 2003; 15:1212 - 26; http://dx.doi.org/10.1105/tpc.009464; PMID: 12724545
- Gilliland A, Singh DP, Hayward JM, Moore CA, Murphy AM, York CJ, et al. Genetic modification of alternative respiration has differential effects on antimycin A-induced versus salicylic acid-induced resistance to Tobacco mosaic virus.. Plant Physiol 2003; 132:1518 - 28; http://dx.doi.org/10.1104/pp.102.017640; PMID: 12857832
- Gleason C, Huang S, Thatcher LF, Foley RC, Anderson CR, Carroll AJ, et al. Mitochondrial complex II has a key role in mitochondrial-derived reactive oxygen species influence on plant stress gene regulation and defense. Proc Natl Acad Sci U S A 2011; 108:10768 - 73; http://dx.doi.org/10.1073/pnas.1016060108; PMID: 21670306
- Rosenwasser S, Rot I, Sollner E, Meyer AJ, Smith Y, Leviatan N, et al. Organelles contribute differentially to reactive oxygen species-related events during extended darkness. Plant Physiol 2011; 156:185 - 201; http://dx.doi.org/10.1104/pp.110.169797; PMID: 21372201
- Schwarzländer M, König AC, Sweetlove LJ, Finkemeier I. The impact of impaired mitochondrial function on retrograde signalling: a meta-analysis of transcriptomic responses. J Exp Bot 2012; 63:1735 - 50; http://dx.doi.org/10.1093/jxb/err374; PMID: 22131156
- Ho LHM, Giraud E, Uggalla V, Lister R, Clifton R, Glen A, et al. Identification of regulatory pathways controlling gene expression of stress-responsive mitochondrial proteins in Arabidopsis. Plant Physiol 2008; 147:1858 - 73; http://dx.doi.org/10.1104/pp.108.121384; PMID: 18567827
- Giraud E, Van Aken O, Ho LHM, Whelan J. The transcription factor ABI4 is a regulator of mitochondrial retrograde expression of ALTERNATIVE OXIDASE1a.. Plant Physiol 2009; 150:1286 - 96; http://dx.doi.org/10.1104/pp.109.139782; PMID: 19482916
- Gaupels F, Kuruthukulangarakoola GT, Durner J. Upstream and downstream signals of nitric oxide in pathogen defence. Curr Opin Plant Biol 2011; 14:707 - 14; http://dx.doi.org/10.1016/j.pbi.2011.07.005; PMID: 21816662
- Suzuki N, Miller G, Morales J, Shulaev V, Torres MA, Mittler R. Respiratory burst oxidases: the engines of ROS signaling. Curr Opin Plant Biol 2011; 14:691 - 9; http://dx.doi.org/10.1016/j.pbi.2011.07.014; PMID: 21862390
- Baudouin E. The language of nitric oxide signalling. Plant Biol (Stuttg) 2011; 13:233 - 42; http://dx.doi.org/10.1111/j.1438-8677.2010.00403.x; PMID: 21309969
- Mittler R, Vanderauwera S, Suzuki N, Miller G, Tognetti VB, Vandepoele K, et al. ROS signaling: the new wave?. Trends Plant Sci 2011; 16:300 - 9; http://dx.doi.org/10.1016/j.tplants.2011.03.007; PMID: 21482172
- Epple P, Mack AA, Morris VRF, Dangl JL. Antagonistic control of oxidative stress-induced cell death in Arabidopsis by two related, plant-specific zinc finger proteins. Proc Natl Acad Sci U S A 2003; 100:6831 - 6; http://dx.doi.org/10.1073/pnas.1130421100; PMID: 12732715
- Yun B-W, Feechan A, Yin M, Saidi NBB, Le Bihan T, Yu M, et al. S-nitrosylation of NADPH oxidase regulates cell death in plant immunity. Nature 2011; 478:264 - 8; http://dx.doi.org/10.1038/nature10427; PMID: 21964330
- Pattanayak GK, Venkataramani S, Hortensteiner S, Kunz L, Christ B, Moulin M, et al. Accelerated cell death 2 suppresses mitochondrial oxidative bursts and modulates cell death in Arabidopsis. Plant J 2012; 69:589 - 600; http://dx.doi.org/10.1111/j.1365-313X.2011.04814.x; PMID: 21988537
- Simková K, Moreau F, Pawlak P, Vriet C, Baruah A, Alexandre C, et al. Integration of stress-related and reactive oxygen species-mediated signals by Topoisomerase VI in Arabidopsis thaliana.. Proc Natl Acad Sci U S A 2012; 109:16360 - 5; http://dx.doi.org/10.1073/pnas.1202041109; PMID: 22988090
- Møller IM. Plant mitochondria and oxidative stress: electron transport, NADPH turnover, and metabolism of reactive oxygen species. Annu Rev Plant Physiol Plant Mol Biol 2001; 52:561 - 91; http://dx.doi.org/10.1146/annurev.arplant.52.1.561; PMID: 11337409
- Poyton RO, Ball KA, Castello PR. Mitochondrial generation of free radicals and hypoxic signaling. Trends Endocrinol Metab 2009; 20:332 - 40; http://dx.doi.org/10.1016/j.tem.2009.04.001; PMID: 19733481
- Morgan MJ, Lehmann M, Schwarzländer M, Baxter CJ, Sienkiewicz-Porzucek A, Williams TCR, et al. Decrease in manganese superoxide dismutase leads to reduced root growth and affects tricarboxylic acid cycle flux and mitochondrial redox homeostasis. Plant Physiol 2008; 147:101 - 14; http://dx.doi.org/10.1104/pp.107.113613; PMID: 18337490
- Gupta KJ, Fernie AR, Kaiser WM, van Dongen JT. On the origins of nitric oxide. Trends Plant Sci 2011; 16:160 - 8; http://dx.doi.org/10.1016/j.tplants.2010.11.007; PMID: 21185769
- Millar AH, Whelan J, Soole KL, Day DA. Organization and regulation of mitochondrial respiration in plants. Annu Rev Plant Biol 2011; 62:79 - 104; http://dx.doi.org/10.1146/annurev-arplant-042110-103857; PMID: 21332361
- Cvetkovska M, Vanlerberghe GC. Alternative oxidase modulates leaf mitochondrial concentrations of superoxide and nitric oxide. New Phytol 2012; 195:32 - 9; http://dx.doi.org/10.1111/j.1469-8137.2012.04166.x; PMID: 22537177
- Cvetkovska M, Vanlerberghe GC. Alternative oxidase impacts the plant response to biotic stress by influencing the mitochondrial generation of reactive oxygen species. Plant Cell Environ (in press; http://onlinelibrary.wiley.com/doi/10.1111/pce.12009/pdf).
- Vanlerberghe GC, Cvetkovska M, Wang J. Is the maintenance of homeostatic mitochondrial signaling during stress a physiological role for alternative oxidase?. Physiol Plant 2009; 137:392 - 406; http://dx.doi.org/10.1111/j.1399-3054.2009.01254.x; PMID: 19549065
- Mur LAJ, Kenton P, Lloyd AJ, Ougham H, Prats E. The hypersensitive response; the centenary is upon us but how much do we know?. J Exp Bot 2008; 59:501 - 20; http://dx.doi.org/10.1093/jxb/erm239; PMID: 18079135
- van Doorn WG, Beers EP, Dangl JL, Franklin-Tong VE, Gallois P, Hara-Nishimura I, et al. Morphological classification of plant cell deaths. Cell Death Differ 2011; 18:1241 - 6; http://dx.doi.org/10.1038/cdd.2011.36; PMID: 21494263
- Modolo LV, Augusto O, Almeida IMG, Magalhaes JR, Salgado I. Nitrite as the major source of nitric oxide production by Arabidopsis thaliana in response to Pseudomonas syringae.. FEBS Lett 2005; 579:3814 - 20; http://dx.doi.org/10.1016/j.febslet.2005.05.078; PMID: 15978583
- Yao N, Greenberg JT. Arabidopsis ACCELERATED CELL DEATH2 modulates programmed cell death. Plant Cell 2006; 18:397 - 411; http://dx.doi.org/10.1105/tpc.105.036251; PMID: 16387834
- Ashtamker C, Kiss V, Sagi M, Davydov O, Fluhr R. Diverse subcellular locations of cryptogein-induced reactive oxygen species production in tobacco Bright Yellow-2 cells. Plant Physiol 2007; 143:1817 - 26; http://dx.doi.org/10.1104/pp.106.090902; PMID: 17277088
- Cvetkovska M, Vanlerberghe GC. Coordination of a mitochondrial superoxide burst during the hypersensitive response to bacterial pathogen in Nicotiana tabacum.. Plant Cell Environ 2012; 35:1121 - 36; http://dx.doi.org/10.1111/j.1365-3040.2011.02477.x; PMID: 22211396
- Wang W, Fang H, Groom L, Cheng A, Zhang W, Liu J, et al. Superoxide flashes in single mitochondria. Cell 2008; 134:279 - 90; http://dx.doi.org/10.1016/j.cell.2008.06.017; PMID: 18662543
- He J, Duan Y, Hua D, Fan G, Wang L, Liu Y, et al. DEXH box RNA helicase-mediated mitochondrial reactive oxygen species production in Arabidopsis mediates crosstalk between abscisic acid and auxin signaling. Plant Cell 2012; 24:1815 - 33; http://dx.doi.org/10.1105/tpc.112.098707; PMID: 22652060
- Schwarzländer M, Murphy MP, Duchen MR, Logan DC, Fricker MD, Halestrap AP, et al. Mitochondrial ‘flashes’: a radical concept repHined. Trends Cell Biol 2012; 22:503 - 8; http://dx.doi.org/10.1016/j.tcb.2012.07.007; PMID: 22917552
- Yao N, Tada Y, Sakamoto M, Nakayashiki H, Park P, Tosa Y, et al. Mitochondrial oxidative burst involved in apoptotic response in oats. Plant J 2002; 30:567 - 79; http://dx.doi.org/10.1046/j.1365-313X.2002.01314.x; PMID: 12047631
- Kuźniak E, Skłodowska M. The effect of Botrytis cinerea infection on the antioxidant profile of mitochondria from tomato leaves. J Exp Bot 2004; 55:605 - 12; http://dx.doi.org/10.1093/jxb/erh076; PMID: 14966215
- Ordog SH, Higgins VJ, Vanlerberghe GC. Mitochondrial alternative oxidase is not a critical component of plant viral resistance but may play a role in the hypersensitive response. Plant Physiol 2002; 129:1858 - 65; http://dx.doi.org/10.1104/pp.003855; PMID: 12177499
- Lee BH, Lee H, Xiong L, Zhu JK. A mitochondrial complex I defect impairs cold-regulated nuclear gene expression. Plant Cell 2002; 14:1235 - 51; http://dx.doi.org/10.1105/tpc.010433; PMID: 12084824
- Kim M, Lee U, Small I, des Francs-Small CC, Vierling E. Mutations in an Arabidopsis mitochondrial transcription termination factor-related protein enhance thermotolerance in the absence of the major molecular chaperone HSP101. Plant Cell 2012; 24:3349 - 65; http://dx.doi.org/10.1105/tpc.112.101006; PMID: 22942382
- Nunes-Nesi A, Carrari F, Gibon Y, Sulpice R, Lytovchenko A, Fisahn J, et al. Deficiency of mitochondrial fumarase activity in tomato plants impairs photosynthesis via an effect on stomatal function. Plant J 2007; 50:1093 - 106; http://dx.doi.org/10.1111/j.1365-313X.2007.03115.x; PMID: 17461782
- Begcy K, Mariano ED, Mattiello L, Nunes AV, Mazzafera P, Maia IG, et al. An Arabidopsis mitochondrial uncoupling protein confers tolerance to drought and salt stress in transgenic tobacco plants. PLoS One 2011; 6:e23776; http://dx.doi.org/10.1371/journal.pone.0023776; PMID: 21912606
- Djebbar R, Rzigui T, Pétriacq P, Mauve C, Priault P, Fresneau C, et al. Respiratory complex I deficiency induces drought tolerance by impacting leaf stomatal and hydraulic conductances. Planta 2012; 235:603 - 14; http://dx.doi.org/10.1007/s00425-011-1524-7; PMID: 22002624
- Chaki M, Valderrama R, Fernández-Ocaña AM, Carreras A, Gómez-Rodríguez MV, López-Jaramillo J, et al. High temperature triggers the metabolism of S-nitrosothiols in sunflower mediating a process of nitrosative stress which provokes the inhibition of ferredoxin-NADP reductase by tyrosine nitration. Plant Cell Environ 2011; 34:1803 - 18; http://dx.doi.org/10.1111/j.1365-3040.2011.02376.x; PMID: 21676000
- Vanlerberghe GC, Yip JYH, Parsons HL. In organello and in vivo evidence of the importance of the regulatory sulfhydryl/disulfide system and pyruvate for alternative oxidase activity in tobacco. Plant Physiol 1999; 121:793 - 803; http://dx.doi.org/10.1104/pp.121.3.793; PMID: 10557227
- Amirsadeghi S, Robson CA, Vanlerberghe GC. The role of the mitochondrion in plant responses to biotic stress. Physiol Plant 2007; 129:253 - 66; http://dx.doi.org/10.1111/j.1399-3054.2006.00775.x
- Swidzinski JA, Sweetlove LJ, Leaver CJ. A custom microarray analysis of gene expression during programmed cell death in Arabidopsis thaliana.. Plant J 2002; 30:431 - 46; http://dx.doi.org/10.1046/j.1365-313X.2002.01301.x; PMID: 12028573
- Norman C, Howell KA, Millar AH, Whelan JM, Day DA. Salicylic acid is an uncoupler and inhibitor of mitochondrial electron transport. Plant Physiol 2004; 134:492 - 501; http://dx.doi.org/10.1104/pp.103.031039; PMID: 14684840
- de Souza WR, Vessecchi R, Dorta DJ, Uyemura SA, Curti C, Vargas-Rechia CG. Characterization of Rubus fruticosus mitochondria and salicylic acid inhibition of reactive oxygen species generation at Complex III/Q cycle: potential implications for hypersensitive response in plants. J Bioenerg Biomembr 2011; 43:237 - 46; http://dx.doi.org/10.1007/s10863-011-9357-4; PMID: 21611778
- Millar AH, Day DA. Nitric oxide inhibits the cytochrome oxidase but not the alternative oxidase of plant mitochondria. FEBS Lett 1996; 398:155 - 8; http://dx.doi.org/10.1016/S0014-5793(96)01230-6; PMID: 8977097
- Castro L, Demicheli V, Tórtora V, Radi R. Mitochondrial protein tyrosine nitration. Free Radic Res 2011; 45:37 - 52; http://dx.doi.org/10.3109/10715762.2010.516254; PMID: 20942571
- Daiber A. Redox signaling (cross-talk) from and to mitochondria involves mitochondrial pores and reactive oxygen species. Biochim Biophys Acta 2010; 1797:897 - 906; http://dx.doi.org/10.1016/j.bbabio.2010.01.032; PMID: 20122895
- Mur LAJ, Brown IR, Darby RM, Bestwick CS, Bi Y-M, Mansfield JW, et al. A loss of resistance to avirulent bacterial pathogens in tobacco is associated with the attenuation of a salicylic acid-potentiated oxidative burst. Plant J 2000; 23:609 - 21; http://dx.doi.org/10.1046/j.1365-313x.2000.00825.x; PMID: 10972887