Abstract
StREM1.3 Remorin is a well-established plant raftophilic protein, predominantly associated with sterol- and sphingolipid-rich membrane rafts. We recently identified a C-terminal domain (RemCA) required and sufficient for StREM1.3 anchoring to the plasma membrane. Here, we report a search for homologs and analogs of RemCA domain in publicly available protein sequence and structure databases. We could not identify RemCA homologous domains outside the Remorin family but we identified domains sharing bias in amino-acid composition and predicted structural fold with RemCA in bacterial, viral and animal proteins. These results suggest that RemCA emerged by convergent evolution among unrelated membrane binding domain.
Membrane rafts are plasma membrane (PM) microdomains acting as signaling platforms and pathogens entry sites.Citation1 The biological importance of membrane rafts has been suggested by the accumulation of specific transmembrane, membrane-anchored and soluble proteins to these focal sites at the cell periphery,Citation2 sometimes designated as “raftophilic” proteins. While post-translational protein modifications can increase membrane raft affinity of anchored proteinsCitation3 the mechanisms of protein anchoring to membrane rafts remain largely unknown.
Plant raftophilic proteins include members of the Remorin family. Remorins are plant-specific oligomeric proteins that have frequently been reported to localize to the PM despite their overall hydrophilic nature.Citation4,Citation5 Membrane association of Arabidopsis AtREM1.4, Potato StREM1.3 and Medicago MtSYMREM1 Remorins is resistant to solubilization by mild detergent at low temperature defining detergent insoluble membranes (DIMs).Citation6,Citation7 In addition, a combination of biochemistry and cell biology approaches demonstrated that StREM1.3 forms ~75 nm wide clusters in the inner leaflet of the plasma membrane in a sterol-dependent manner, demonstrating the existence of remorin-containing membrane microdomains.Citation6
There is nevertheless no recognizable membrane targeting domain in Remorin sequences. In a recent publication, we showed that a domain of 28 residues at the C-terminus of the potato StREM1.3 (RemCA) is required and sufficient for anchoring to the PM.Citation8 This domain adopts an alpha-helical conformation in polar environments. It directly binds to lipids and biological membrane, with higher affinity for negatively charged lipids abundant in DIMs. Mutations in RemCA abolish StREM1.3 PM binding and the function of StREM1.3 in the suppression of PVX virus propagation.
With an aim to use RemCA sequence to identify new raftophilic proteins targeted to the PM by similar domains, we conducted in this work a search for homologs and analogs of RemCA domain in publicly available protein sequence and structure databases. Our approaches failed to identify any RemCA homologous domain outside the Remorin family, suggesting that RemCA does not originate from an ancestral membrane binding domain shared with lineages beyond the plant kingdom. However, we provide evidence that RemCA domain shares bias in amino-acid composition and predicted structural fold with membrane binding domains of bacterial, viral and animal proteins. These results point to the existence of RemCA analogs outside the plant kingdom, supporting the hypothesis of an emergence of RemCA by convergent evolution among unrelated membrane binding domain.
RemCA Homologs are Restricted to the Remorin Family
To identify domains homologous to RemCA, we built a hidden Markov model (HMM) using the sequences of the RemCA peptides from StREM1.3, MtSYMREM1 and AtREM1.4, all reported to localize to DIMs. HMM searches with this model retrieved 117 RemCA-like peptides in Remorins from 26 plant species. The sequence logo obtained with the alignment of these 117 peptides revealed highly conserved residues such as E9 (97% conserved), R15 (98% conserved) and P21 (100% conserved, ). No significant hits were found outside the plant kingdom or outside the Remorin family.
Figure 1. Diversity of RemCA domains across the plant kingdom and the identification of analogous membrane binding domains. (A) Natural diversity and conservation in the RemCA sequence illustrated by a sequence logo based on the alignment of 117 RemCA peptides from 26 plant species. (B) Analysis of bias in amino acid composition found in RemCA sequences. Frequencies are given as the average number of amino acids per 28 amino-acid long peptides in RemCA (Y-axis) or random 28- amino-acid long peptides extracted from the Uniprot database (Y-axis). (C) Two examples of membrane binding domains analogs of RemCA domain. The domains are shown in the context of the full-length protein structure (left) and superimposed with StREM1.3 RemCA model (right).
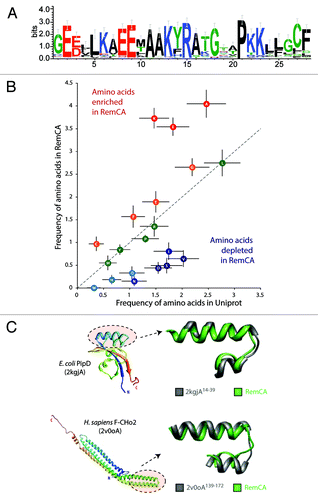
RemCA Domain Shows Amino Acid Composition Bias
The limited similarity of RemCA to sequences in protein databases suggests that it does not derive from an ancestral membrane anchor domain but rather represent a case of convergent evolution of a functional membrane anchor domain. Domains resulting from convergent evolution of proteins under enforced physico-chemical constraints often show a clear amino acid compositional bias.Citation9,Citation10 The most abundant amino acids in the 117 RemCA peptides were Alanine, Lysine and Glutamic acid that represent respectively 4.04, 3.73 and 3.54 residues in average out the 28 comprised in RemCA (). By comparison, Alanine, Lysine and Glutamic acid were found in average 2.47, 1.47 and 1.83 times per peptide in a set of 100 000 peptides randomly extracted from Uniprot. Conversely, Valine, Serine and Aspartic acid were found in average 0.64, 0.49 and 0.44 times in RemCA peptides but 2.03, 1.71 and 1.55 times respectively in random peptides. This shows that the RemCA domain harbors a clear composition bias toward enrichment in Glutamic acid, Lysine and Alanine. This observation supports the prevalence of physical and chemical constraints in the evolution of RemCA domain.
Unrelated Membrane Binding Domains are RemCA Analogs
To identify protein domain analogous to RemCA, we used StREM1.3 RemCA predicted structure as a template in a fold recognition search through the PDB database. The aligned segments were then classified based on a test for correlation between their amino-acid composition and the average composition of 117 RemCA peptides. A total of 215 domains were selected after fold recognition search, with Pearson correlation coefficient for amino acid composition (ρ) ranging from -0.25 to 0.84. Among those, 32 belonged to annotated proteins and showed ρ > 0.5 (). The peptide with the best composition correlation (ρ = 0.84) corresponds to the N-terminal inner membrane anchor of the PpiD periplasmic chaperone from E. coli ().Citation11 Another peptide with high composition correlation (ρ = 0.65) corresponds to the kink region between alpha-helices 3 and 4 sensing membrane curvature in BAR proteins from human ().Citation12 The viral flavin-dependant thymidylate synthase ThyX was retrieved with ρ = 0.80. Domains found in membrane-bound proteins from mammals, bacteria and viruses therefore appear as RemCA analogs. These results suggest that the evolution of membrane binding peptides has converged toward tight hairpin of helices with amino acid composition biased toward alanine and charged amino acids in multiple lineages.
Table 1. RemCA analogous peptides identified by fold recognition searches showing a Pearson correlation coefficient for amino acid composition > 0.5
Helical peptides can be anchored in biological membranes perpendicularly (transmembrane α-helices), tangent to the membrane plane (in plane membrane anchors), with an oblique orientation (tilted peptides) or oblique and forming a kink or a hairpin (fusion peptides).Citation13 alpha;-helical membrane proteins insertion is assisted by translocons at synthesis, resulting in intrinsic membrane proteins, the other topologies of membrane anchors allow otherwise soluble proteins to associate with membranes dynamically, like in the case of Tail-anchored proteins.Citation14
Kinked membrane anchors were notably found in viral fusion proteins, such as the gp32 fusion protein of the SIV or Influenza virus hemagglutinin.Citation15,Citation16 where they mediate the first steps of the fusion between the host cell and the viral membrane. They were also detected in proteins involved in lipid metabolism, in signal sequences, in membrane proteins and toxic proteins that must cross the membrane to exert their activity such as E. coli Colicins.Citation17 In good agreement, using fold recognition and compositional bias analysis, we identified membrane-bound enzymes (e.g., PrsA isomerase; ρ = 0.78), membrane structural proteins (F-CHO2 BAR domain containing protein ρ = 0.65, tubulin folding cofactor A, ρ = 0.67) and other membrane-associated proteins (e.g., the peptidoglycan-associated lipoprotein from Hemophilus influenza, ρ = 0.61) among proteins presenting a hairpin of alpha-helices with a composition close to RemCA. Because of the strong physico-chemical constraints acting on transmembrane helices, similarities in such segments are more likely to result from convergent evolution than from common ancestry.Citation10 In the present case, this interpretation is reinforced by the fact that the proteins identified generally do not share extensive similarities in the rest of their sequence. Segment of proteins involved in nucleic acids binding (e.g., RuvA helicase, ρ = 0.66; KorB helix-turn-helix DNA binding protein, ρ = 0.75) were also retrieved, suggesting that domains sharing properties with RemCA might have evolved for binding other negatively charged polymers.
Material and Methods
Identification of RemCA-like peptides by HMM searches
HMM model analyses were performed using tools from the EMBOSS package, with searches against local sequence databases and local instances of the Uniprot SProt and Trembl databases. The RemCA bait model was built using the sequences: GEDLLKAEELAAKYRATGTAPKKILGIF, GEEILKAEELAAKYRATGTAPKKLFGCM and GEEILKVEETAAKFRTRGYQPRRLLGCF, corresponding to the RemCA domains of StREM1.3, AtREM1.4 and MtSYMREM respectively.
Amino acids composition bias analysis
Amino acids composition bias analysis is based on a comparison between the average number of each amino acid in 28 amino-acid long peptides derived from the 117 identified RemCA sequences and the average number in a set of 100,000 peptides of 28 amino acids randomly extracted from SProt database using homemade scripts.
Identification of StREM1.3 RemCA analogs by fold recognition searches
Fold recognition searches were performed online with the FATCAT serverCitation18 against the PDB database. A total of 3576 structures were retrieved with e-value < 5.10-2. To increase the likelihood of identifying functionally relevant analogs, we limited results to hits with no gaps, chain RMSD < 1.7Å and alignment length equal to the length of RemCA ± 5 amino acids, leading to a list of 215 analogs.
Amino acid composition correlation analysis
The correlation of amino acid composition was estimated using Pearson correlation coefficients calculated in R. The frequency of each amino acid in each RemCA analog was compared with the average frequency of each amino acid in the 117 RemCA-like peptides identified by HMM search.
Acknowledgments
This work was supported by the French Agence Nationale pour la Recherche (contract no. NT09_517917 PANACEA) to S.M.
Disclosure of Potential Conflicts of Interest
No potential conflicts of interest were disclosed.
References
- Lingwood D, Simons K. Lipid rafts as a membrane-organizing principle. Science 2010; 327:46 - 50; http://dx.doi.org/10.1126/science.1174621; PMID: 20044567
- DiNitto JP, Cronin TC, Lambright DG. Membrane recognition and targeting by lipid-binding domains. Sci STKE 2003; 2003:re16; http://dx.doi.org/10.1126/stke.2132003re16; PMID: 14679290
- Levental I, Grzybek M, Simons K. Greasing their way: lipid modifications determine protein association with membrane rafts. Biochemistry 2010; 49:6305 - 16; http://dx.doi.org/10.1021/bi100882y; PMID: 20583817
- Reymond P, Kunz B, Paul-Pletzer K, Grimm R, Eckerskorn C, Farmer EE. Cloning of a cDNA encoding a plasma membrane-associated, uronide binding phosphoprotein with physical properties similar to viral movement proteins. Plant Cell 1996; 8:2265 - 76; PMID: 8989883
- Raffaele S, Mongrand S, Gamas P, Niebel A, Ott T. Genome-wide annotation of remorins, a plant-specific protein family: evolutionary and functional perspectives. Plant Physiol 2007; 145:593 - 600; http://dx.doi.org/10.1104/pp.107.108639; PMID: 17984200
- Raffaele S, Bayer E, Lafarge D, Cluzet S, German Retana S, Boubekeur T, et al. Remorin, a solanaceae protein resident in membrane rafts and plasmodesmata, impairs potato virus X movement. Plant Cell 2009; 21:1541 - 55; http://dx.doi.org/10.1105/tpc.108.064279; PMID: 19470590
- Lefebvre B, Timmers T, Mbengue M, Moreau S, Hervé C, Tóth K, et al. A remorin protein interacts with symbiotic receptors and regulates bacterial infection. Proc Natl Acad Sci USA 2010; 107:2343 - 8; http://dx.doi.org/10.1073/pnas.0913320107; PMID: 20133878
- Perraki A, Cacas JL, Crowet JM, Lins L, Castroviejo M, German-Retana S, et al. Plasma membrane localization of StREM1. 3 Remorin is mediated by conformational changes in a novel C-terminal anchor and required for the restriction of PVX movement. Plant Physiol 2012; 160:624 - 37; http://dx.doi.org/10.1104/pp.112.200519; PMID: 22855937
- Paul S, Bag SK, Das S, Harvill ET, Dutta C. Molecular signature of hypersaline adaptation: insights from genome and proteome composition of halophilic prokaryotes. Genome Biol 2008; 9:R70; http://dx.doi.org/10.1186/gb-2008-9-4-r70; PMID: 18397532
- Wong WC, Maurer-Stroh S, Eisenhaber F. More than 1,001 problems with protein domain databases: transmembrane regions, signal peptides and the issue of sequence homology. PLoS Comput Biol 2010; 6:e1000867; http://dx.doi.org/10.1371/journal.pcbi.1000867; PMID: 20686689
- Weininger U, Jakob RP, Kovermann M, Balbach J, Schmid FX. The prolyl isomerase domain of PpiD from Escherichia coli shows a parvulin fold but is devoid of catalytic activity. Protein Sci 2010; 19:6 - 18; PMID: 19866485
- Henne WM, Kent HM, Ford MGJ, Hegde BG, Daumke O, Butler PJG, et al. Structure and analysis of FCHo2 F-BAR domain: a dimerizing and membrane recruitment module that effects membrane curvature. Structure 2007; 15:839 - 52; http://dx.doi.org/10.1016/j.str.2007.05.002; PMID: 17540576
- Lins L, Decaffmeyer M, Thomas A, Brasseur R. Relationships between the orientation and the structural properties of peptides and their membrane interactions. Biochim Biophys Acta 2008; 1778:1537 - 44; http://dx.doi.org/10.1016/j.bbamem.2008.04.006; PMID: 18501700
- Renthal R. Helix insertion into bilayers and the evolution of membrane proteins. Cell Mol Life Sci 2010; 67:1077 - 88; http://dx.doi.org/10.1007/s00018-009-0234-9; PMID: 20039094
- Han X, Bushweller JH, Cafiso DS, Tamm LK. Membrane structure and fusion-triggering conformational change of the fusion domain from influenza hemagglutinin. Nat Struct Biol 2001; 8:715 - 20; http://dx.doi.org/10.1038/90434; PMID: 11473264
- Tamm LK, Lai AL, Li Y. Combined NMR and EPR spectroscopy to determine structures of viral fusion domains in membranes. Biochim Biophys Acta 2007; 1768:3052 - 60; http://dx.doi.org/10.1016/j.bbamem.2007.09.010; PMID: 17963720
- Lins L, El Kirat K, Charloteaux B, Flore C, Stroobant V, Thomas A, et al. Lipid-destabilizing properties of the hydrophobic helices H8 and H9 from colicin E1. Mol Membr Biol 2007; 24:419 - 30; http://dx.doi.org/10.1080/09687860701228254; PMID: 17710646
- Veeramalai M, Ye Y, Godzik A. TOPS++FATCAT: fast flexible structural alignment using constraints derived from TOPS+ Strings Model. BMC Bioinformatics 2008; 9:358; http://dx.doi.org/10.1186/1471-2105-9-358; PMID: 18759993