Abstract
Shade avoidance response (S.A.R) is regulated by light and circadian clock. Circadian clock controls S.A.R by the transcriptional regulation of positive regulators of S.A.R, PIF4 and PIF5, to prevent plants from responding to 'light' of dark period. Thus, in many cases, deficits in circadian clock appear in abnormalities of hypocotyl and/or petiole elongation. Previously, interesting phenomena were reported that the triple mutants of PSEUDO RESPONSE REGULATORS9, 7 and 5, which are clock components, show longer petioles and smaller leaves under light/dark cycle than those under continuous lighting. These S.A.R-like phenotypes cannot be explained by their hyposensitivity to red light. We demonstrated detailed analyses of this mutant to reveal the leaf-specific S.A.R regulated by circadian clock. Expression analyses of S.A.R-related genes suggested that PRR5 functions as a repressor of S.A.R. Morphological analyses of leaves under different light condition revealed that PRR5 is involved in the inhibition of leaf expansion in S.A.R.
Introduction
In plants, shade avoidance response (S.A.R), such as promotion of stem and/or petiole elongation and repression of leaf expansion, is one of the important adaptive responses to changes of light environment. Under shade cast by canopies (canopy-shade), the ratio of red light (R) to infrared light (FR) (R/FR) and intensity of blue light are decreased by the absorption of red and blue light by photosynthetic pigments. The former and latter are perceived by red/infrared photoreceptors: phytochromes and blue/UV receptors: cryptochromes, respectively.Citation1-Citation4 In Arabidopsis thaliana (L.) Heynh. (Arabidopsis), five phytochrome-encoding genes, PHYA to PHYE, and two cryptochrome genes, CRY1 and CRY2, have been isolated.Citation1-Citation6 PHYB and CRY1 play major roles in S.A.R.Citation3,Citation7,Citation8 The ratio of R to FR is perceived as the photoequilibrium between FR-absorbing form (Pfr) and R-absorbing form (Pr) of PHYB. Pfr and Pr reversibly photointerconvert each other.Citation9 On the other hand, CRY1 is activated in a manner depending on intensity of blue light.Citation10 Pfr form of PHYB (PhyBfr) and activated CRY1 repress S.A.R.Citation8,Citation11
PhyBfr accumulates in nuclei and interacts with a group of basic helix-loop-helix (bHLH) transcriptional factors, which are called phytochrome interacting factors (PIFs).Citation12-Citation14 Their functions partially and intricately overlap each other.Citation15 Especially, PIF3, PIF4 and PIF5, which interact with and degraded by PhyBfr, act as positive regulators of S.A.R.Citation16-Citation19 They directly bind to G-box and/or E-BOX motifs located in the promoter regions of the downstream genes in phytochrome-mediated signal transduction pathway, such as other bHLH transcriptional factors (e.g., PIL1 and HFR1), YUCCAs that participate in auxin biosynthesis, IAA/AUX which regulate auxin signal transduction and a HD-Zip gene, AtHB-2.Citation20-Citation22 Particularly, PIL1, AtHB-2 and HFR1 are rapidly induced by canopy-shade stimulus, thus their expression are often used as indexes of S.A.R.Citation23,Citation24
S.A.R is regulated not only by light but also by circadian clock.Citation25,Citation26 A lot of mutants of circadian clock genes display abnormalities in photomorphogenesis, such as hypocotyl elongation.Citation27-Citation29 Currently, it is thought that circadian clock regulates S.A.R mainly to repress the expression of PIF4 and PIF5 from dusk until midnight.Citation26,Citation28,Citation30 This diurnal change in the regulation of S.A.R helps plants to respond to light environment only during light period.
A mutant of PSEUDO RESPONSE REGULATOR9, 7 and 5 (PRR9, PRR7 and PRR5), which are components for circadian clock, is also one of the evidences of the connection between circadian clock and light signal transduction pathway of S.A.R. prr9;prr7;prr5 mutants show extreme hyposensitivity to red light in hypocotyl elongation and the arrhythmic expression of other major clock genes, CIRCADIAN CLOCK-ASSOCIATED 1 (CCA1), LATE ELONGATED HYPOCOTYL (LHY), TIMIMG OF CAB EXPRESSION 1 (TOC1) and GIGANTEA (GI).Citation27 Interestingly, Niinuma et al.Citation31 reported that the leaves of prr9;prr7;prr5 mutants grown under 16h light/8 h dark cycle (LD) show extremely longer petioles and smaller leaf blades than those grown under continuous lighting (LL), while their hypocotyls are much longer than those of wild type (WT) under LD and LL. Similar phenomena to these have not been reported. Ordinarily, both hypocotyls and petioles are the stalk organs to support photosynthetic organs: leaves physically, and they have same behaviors of longitudinal growth promotion in S.A.R.Citation19,Citation32,Citation33 Those phenomena of prr9;prr7;prr5 mutants cannot be explained only by the abnormal S.A.R caused by defective circadian clock.
In this study, we assured that the above-mentioned phenomena of prr9;prr7;prr5 mutants were the clues to reveal the leaf-specific regulation of S.A.R, and performed detailed analyses of light responses in the leaves of prr mutants. Morphological analyses of leaves under different light qualities suggested that sensitivity to red light kept in prr9;prr7;prr5 mutants is involved in leaf-specific S.A.R. Analyses of S.A.R-related gene expressions revealed that PRR5 has a unique function to repress the amplitude of S.A.R in leaves. Furthermore, genetic analyses indicated that PRR5 regulates S.A.R in the downstream of PIF5. This suggested the existence of direct regulation of S.A.R in the downstream of PIFs by circadian clock components. Interestingly, low R/FR treatment of prr mutants suggested that PRR5 is involved in the inhibition of leaf expansion in S.A.R. Collectively, this study indicates a new point of contact between circadian clock and S.A.R and a tissue-specific function of a circadian clock component.
Result
prr9;prr7;prr5 mutants have deficit in red light signaling pathway
In order to analyze nature of reported S.A.R-like phenomena exhibited by the prr9;prr7;prr5 mutants, we measured the length of leaf blade and petioles of prr mutants under continuous light LL or LD (). In this study, it was noted that the T-DNA insertion lines of prr9-10 (SALK_007551) and prr7-11 (SALK_030430) were considered as null alleles of PRR9 and PRR7, respectively, because their transcripts were not detected in the respective lines.Citation27 Although a truncated transcript of PRR5 was expressed in prr5-11 (KG24599) mutants, they showed almost the same phenotypes of a null allele of PRR5: prr5-3 (SALK_064538) in light responsibility and period of rhythms in LL, as previously reported.Citation34 Thus, prr5-11 line was assumed to be a null allele of PRR5. Plants were grown for 22 d after germination (d.a.g) under LL or LD, and then their 3rd leaves were sampled and measured. Under LL, the petiole lengths of all prr mutants except for prr9;prr7;prr5 mutants were not different from those of WT. The leaf blades of prr7;prr5 mutants were slightly shorter than those of WT, and the leaf blades and petioles of prr9;prr7;prr5 mutants were much shorter than those of WT. Under LD, the leaf blades and petioles of all prr mutants except for prr9;prr7;prr5 mutants were shorter than those of WT. prr9;prr7;prr5 mutants only displayed extremely short leaf blades under LD. Interestingly, the petioles of prr9;prr7;prr5 mutants under LD were much longer than those of them under LL, unlike the other prr mutants and WT (). Although the hypocotyls of prr9;prr7, prr9;prr5 and prr7;prr5 mutants were longer than those of WT under both LL and LD, their leaf shapes were hardly different from those of WT (). These results indicated that the behavior of hypocotyl elongation is not always the same with that of petiole elongation.
Figure 1. Length of leaf blade, petiole and hypocotyl of prr mutants grown under continuous light (LL, left panel) or 16 h light/8 h dark (LD, right panel). d9, d7 and d5 in these figures mean prr9, prr7 and prr5 mutants, respectively. Double and triple mutants were represented by the combinations of these abbreviations. (A) Plants were grown under for 22 d.a.g under 40 μmol m-2 s-1 PPFD, and then 3rd leaves were measured (n ≥ 6). (B) Seedlings were grown for 7 d.a.g under 20 μmol m-2 s-1 PPFD and measured (n ≥ 12). Error bars represent SE, and single or double asterisks indicate significant difference from WT using Tukey's LSD (*p ≤ 0.05, **p ≤ 0.01).
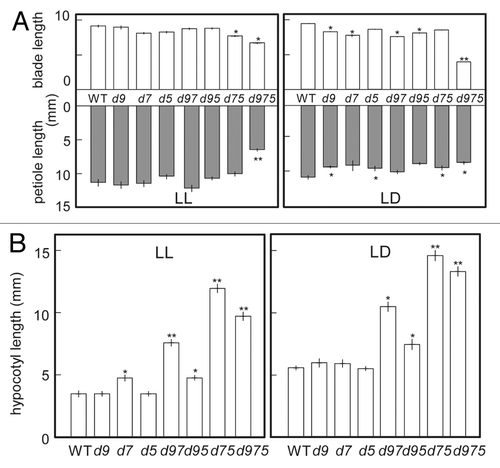
To determine which light signal transduction pathways are involved in the S.A.R-like phenomena of prr9;prr7;prr5 mutants under LD, we measured the leaf shapes of prr9;prr7;prr5 mutants treated with continuous red or blue light (). Because the leaves of prr9;prr7;prr5 mutants were much smaller than those of WT under any kind of light condition, we could not compare the effects of light quality on leaf shape using their absolute dimensions. Thus, in each line, the dimensions of leaves under red or blue light were normalized to the average dimensions of those under white light. Under red light, prr9;prr7;prr5 mutants displayed longer petioles and smaller leaf blades, similarly to S.A.R, than those grown under continuous white light (). Under blue light, the leaf shapes of prr9;prr7;prr5 mutants were not different from those of WT, except in size (). These demonstrate that the leaves of prr9;prr7;prr5 mutants have deficits in red light signal transduction pathway in photomorphogenesis as well as their hypocotyls.Citation27
Figure 2. Dimensions of leaf blades and petioles of prr9;prr7;prr5 mutants under red or blue light. Plants were grown under continuous white light (60 μmol m-2 s-1 PPFD) for 16 d.a.g as control groups, or for 10 d.a.g under continuous white light and 6 d under continuous red or blue light (60 μmol m-2 s-1 PFD). 1st and 2nd leaves were measured at 17 d.a.g. (A) The Images of the leaves of WT and prr9;prr7;prr5 (d975) mutants under every light conditions. A scale bar indicates 5 mm. (B) and (C) In each line, each organ size was normalized to the average size of the control group. Error bars represent SE, and asterisks indicate significant difference from WT using Student's t-test (n ≥ 6, p ≤ 0.05).
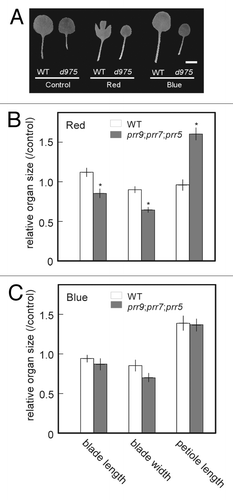
PIF4 and PIF5 mis-expression during dark period in prr9;prr7;prr5
To gain insight into S.A.R-like phenomena in prr9;prr7;prr5 mutants under LD, we analyzed the expression of the molecular markers for S.A.R: HFR1 and AtHB-2, PIF4 and PIF5. We first measured the expressions of these marker genes in prr mutants during dark period. Plants were grown under LD for 20 d.a.g, and then their 3rd and 4th leaves were sampled and measured. AtHB-2 expression was rapidly and intensely induced during dark period in the leaves of prr9;prr7;prr5 mutants, and the same behavior was observed in the leaves of prr7;prr5 mutants without some lag (). These results suggested that S.A.R might be induced in the leaves of prr7;prr5 and prr9;prr7;prr5 mutants during dark period. However, leaves of prr7;prr5 mutants did not show S.A.R-like phenomena unlike their hypocotyls under LD (16 h light/8 h dark) (). Besides the fact that leaves of prr7;prr5 mutants displayed longer petioles than those of WT under 12 h light/12 h dark cycle (Fig. S1), it was supposed that 8 h dark was not long enough for their leaves to cause S.A.R-like phenomena. Additionally, AtHB-2 highly expressed in prr9, prr5 and prr9;prr5 mutants in comparison with WT at the end of dark period (). However, HFR1 expression in them was similar to that in WT (Fig. S2).
Figure 3. The expression of AtHB-2 during dark period in prr mutants. Plants were grown for 22 d.a.g under LD (40 μmol m-2 s-1 PPFD), and then RNA samples were prepared at specific time points from the end of light period to the end of dark period. The abbreviations of prr mutants are described in . Error bars represent SE, and asterisks indicate significant difference from WT using Tukey's LSD (p ≤ 0.05).
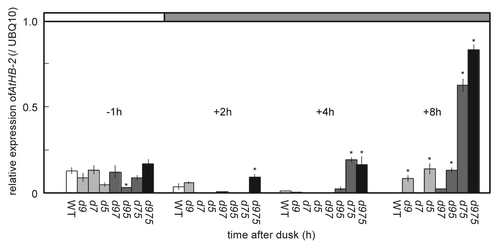
Second, to obtain further insight into what occurred in prr9;prr7;prr5 mutants during dark period, we measured the gene expression profiles of HFR1, PIF4 and PIF5. HFR1 expression in the leaves of prr9;prr7;prr5 mutants was rapidly induced during dark period and remained high as compared with that in the leave of WT throughout the days despite the rapid reduction at dawn (). PIF4 expression in the leaves of prr9;prr7;prr5 mutants under LD was kept considerably higher than that in the leaves of WT throughout the days except at dusk, and PIF5 expression oscillated arrhythmically and dramatically in amplitude (). Besides the arrhythmic phenotypes of prr9;prr7;prr5 mutants as previously reported, these results suggested that mis-expression of PIF4 and PIF5 during dark period induces S.A.R-like phenomena under LD.Citation27 However, this does not explain the rapid induction of AtHB-2 and HFR1 in the beginning of dark period in the leaves of prr9;prr7;prr5 mutants ( and ). Photoactivated phytochrome B (PhyBfr), which degrades PIF4 and PIF5, is stable under dark condition.Citation19,Citation35 Moreover, The dark-induced expressions of AtHB-2 and HFR1 were rapidly reduced by red light irradiation in the leaves of prr9;prr7;prr5 mutants (Fig. S3). Thus, PhyBfr in the leaves of prr9;prr7;prr5 mutants was unlikely to change rapidly in abundance or in conformation in the beginning of dark period.
Extreme induction of S.A.R by FR irradiation in prr9;prr7;prr5 and prr7;prr5 mutants
To gain insight into the rapid induction of the marker genes for S.A.R in the beginning of dark period in the leaves of prr9;prr7;prr5 mutants, we measured expression levels of these genes with end-of-day FR (E.O.D-FR) treatment in loss-of-function mutants and overexpressor (ox) lines of the PRR genes. Plants were grown under LD for 20 d.a.g, and then given FR irradiation at the end of light period. Previous studies reported that phyB mutants and PIF5ox, which display constitutive S.A.R, were hardly different from WT in the expressions of these marker genes induced by low R/FR or E.O.D-FR treatment.Citation19,Citation25,Citation36 Interestingly, the expressions of AtHB-2 and HFR1 in prr7;prr5 and prr9;prr7;prr5 mutants were induced rapidly and approximately 2.5 to 4-fold more highly than those in WT with E.O.D-FR treatment, whereas such intense inductions were not observed in phyB mutants or PIF5ox plants (; Fig. S4). It was also noted that prr5 mutants with E.O.D-FR treatment displayed intense induction of these marker genes as compared with WT and the other prr single mutants with E.O.D-FR treatment (). Furthermore, remarkable suppression of the expression of these marker genes was observed only in PRR5 overexpressors (PRR5ox) with E.O.D-FR treatment, whereas the other PRR overexpressors were hardly different from WT in the marker genes expression (). Besides the fact that prr5 mutants did not display conspicuous phenotypes under normal conditions, these results suggested that PRR5 has a unique function to reduce the amplitude of induced S.A.R, unlike PRR9 and PRR7.
Figure 5. Effect of E.O.D-FR on the expression of AtHB-2 and HFR1 in the leaves of loss-of-function mutants and overexpressor lines of PRR genes. Plants were grown for 20 d.a.g under LD (40 μmol m-2 s-1 PPFD), and then some of them were irradiated with PFD of FR for 15 min (closed symbols) at the end of light period, and the others were not (open symbols). RNA samples were prepared at specific time points. (A) Extreme induction of these marker genes by E.O.D-FR in the leaves of prr7;prr5 and prr9;prr7;prr5 mutants. (B) Intense induction of the marker genes by E.O.D-FR in the leaves of prr5 mutants. (C) Remarkable suppression of the induction of the marker genes by E.O.D-FR in the leaves of PRR5ox.
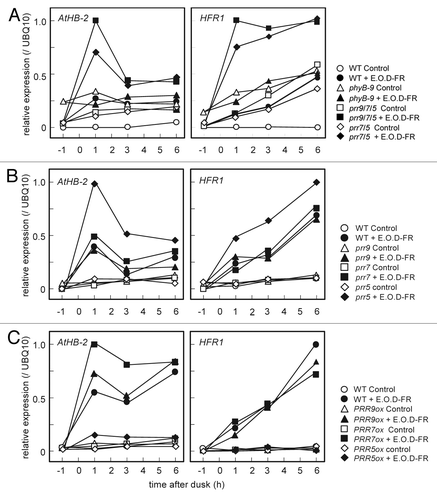
Repression of S.A.R by PRR5 in the downstream of PIF5
To further analyze this unique function of PRR5 in the regulation of S.A.R, we analyzed the phenotypes of the double overexpressors of PIF5ox and PRR5ox (PIF5oxPRR5ox). PIF5ox plants displayed elongated hypocotyls and constitutive expression of AtHB-2 and HFR1 under LL like phyB mutants (). In contrast, PRR5ox plants showed much shorter hypocotyls than those of WT. It was noteworthy that PIF5oxPRR5ox plants displayed as short hypocotyls and low-level expression of AtHB-2 and HFR1 as PRR5ox plants (). These results suggested that PRR5 represses S.A.R in the downstream of PIF5 in phytochrome-mediated light signaling pathway.
Figure 6. Repression of shade avoidance response by PRR5. (A) Hypocotyl length of phyB, prr9;prr7;prr5 mutants, PIF5ox, PRR5ox and PIF5oxPRR5ox. Seedlings were grown under LL (20 μmol m-2 s-1 PPFD) for 10 d.a.g, and then measured (n ≥ 12). Error bars represent SE (B) The expression profiles of AtHB-2 and HFR1 in these seedlings under LL. The seedlings were grown under the same condition of (A) for 12 d.a.g, and then sampled at specific time points after 12 h of dark treatment. RNA samples were extracted from whole above ground parts of the seedlings.
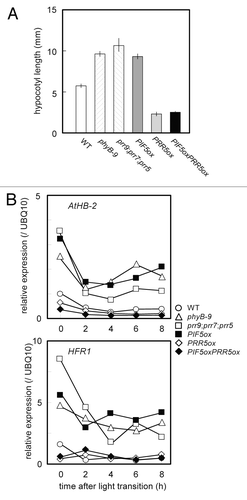
The regulation of leaf-specific S.A.R involved in PRR5
We empirically noticed that prr5 mutants had larger leaves than WT and the other prr mutants when densely planted. It was speculated that prr5 mutants had some defects in S.A.R in leaves. To confirm this speculation, we measured the petiole lengths and the areas of leaf blades of prr single mutants under high or low R/FR. In WT, prr9 and prr7 mutants, smaller leaf blades and longer petioles were shown under low R/FR than those under high R/FR. It was notable that prr5 mutants under low R/FR displayed extremely elongated petioles, and that their leaf blades were as large as those under high R/FR (). Extreme petiole elongation of prr5 mutants under low R/FR was consistent with the intense induction of the marker genes of S.A.R in them with E.O.D-FR treatment (). However, the normal leaf expansion in prr5 mutants under low R/FR could not be explained by it. Besides the slight hyposensitivity to red light in hypocotyl elongation of prr5 mutants, these results suggested that PRR5 is involved in the leaf-specific S.A.R.Citation27 It was also noticed that petiole elongation is not always correlated with the reduction of the leaf blade areas in S.A.R.
Figure 7. Extreme petiole elongation and normal leaf expansion under low R/FR in the leaves of prr5 mutants. Plants were grown under high R/FR (LD, 40 μmol m-2 s-1 PPFD) for 13 d.a.g, and then transferred to continuous high or low R/FR for 7 d. Their 3rd leaves were measured (n = 6). (A) Representative phenotypes of 3rd leaves in WT, phyB-9 and prr mutants grown under high (upper side) or low (lower side) R/FR. A scale bar indicates 5 mm. (B) Petiole length and leaf area of 3rd leaves. Error bars represent SE. Asterisks indicate significant difference from WT using Tukey's LSD (p ≤ 0.05).
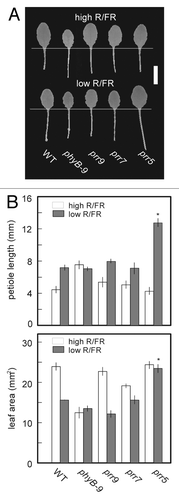
Discussion
Hyposensitivity to red light in the leaves of prr9;prr7;prr5 mutants
Previous analyses of early photomorphogenesis showed that PRR9, PRR7 and PRR5 act as positive regulators of red light signaling pathway and that prr9;prr7;prr5 mutants are extremely hyposensitive to red light in spite of their normal sensitivity to blue light.Citation30,Citation37-Citation39 Consistent with these findings, the leaves of prr9;prr7;prr5 mutants are as hyposensitive as their hypocotyls to red light in spite of their normal sensitivity to blue light (). phyB mutants and PIF5ox plants also show extreme hyposensitivity to red light and normal sensitivities to blue light in hypocotyl elongation.Citation17 However, in this study, their leaves showed S.A.R under both LL and LD, and dramatic change in leaf shape dependent on light/dark cycle was not observed in them, unlike in prr9;prr7;prr5 mutants (data not shown). This suggested that the sensitivity to red light is not lost completely in the leaves of prr9;prr7;prr5 mutants. This is reinforced by the result that the dark-induced expressions of the marker genes for S.A.R were rapidly reduced by red light irradiation (Fig. S3). Considering the result that prr9;prr7;prr5 mutants showed elongated hypocotyls as well as phyB mutants and PIF5ox plants under LL (), the red light sensitivity kept in prr9;prr7;prr5 mutants seems to be involved in leaf-specific S.A.R.
A unique function of PRR5 to reduce the amplitude of S.A.R
According to previous studies, degradation of PIF4 and PIF5 by PhyBfr partially represses S.A.R during light period.Citation19,Citation28 In contrast, the expression of PIF4 and PIF5 are upregulated during light period and downregulated during dark period by light and circadian clock.Citation30 In this study, the mis-expression of PIF4 and PIF5 during dark period was observed in the leaves of prr9;prr7;prr5 mutants (), and this seems to be the cause of S.A.S-like phenotypes of the leaves of prr9;prr7;prr5 mutants under LD. This is consistent with the arrhythmicity of prr9;prr7;prr5 mutants reported previously.Citation21,Citation27 However, this supposition cannot explain the rapid induction of the marker genes for S.A.R in the beginning of dark period in the leaves prr9;prr7;prr5 mutants (; Fig. S2). This is because PIF5ox plants, in which abundance of PIF5 increases rapidly in the beginning of dark period, did not show such rapid induction of the marker genes (Fig. S4).Citation19,Citation28 Furthermore, we found that, with E.O.D-FR treatment, the expressions of the marker genes were intensely induced in the leaves of prr5 mutants and remarkably suppressed in those of PRR5ox plants (). This suggested that PRR5 has a unique function to reduce the amplitude of S.A.R, unlike PRR9 or PRR7. This newly discovered function of PRR5 can explain that, in the leaves of prr9;prr7;prr5 mutants, the absence of PRR5 enhanced the influence of the mis-expressed PIF4 and PIF5 in the beginning of dark period. The same seems to be true for the conspicuous induction of the marker genes by E.O.D-FR treatment in the leaves of prr7;prr5 and prr9;prr7;prr5 mutants ().
Molecular function of PRR5 in phytochrome signaling pathway
It has been proposed that PRR5 together with PRR9 and PRR7 acts as a circadian clock element, and participates in phytochrome-mediated light signal transduction pathways to regulate periodic expression of PIFs directly or indirectly.Citation17,Citation21,Citation28 According to recent studies, PRR5 directly represses the expression of LHY and CCA1, which repress TOC1 transcription, and PRR5 and TOC1 oligomerize with their PRR domain to enhance the stability and nuclear localization of TOC1.Citation40,Citation41 Moreover, overexpressors of TOC1 (TOC1ox) show extreme hypersensitivity to red light in hypocotyl elongation.Citation37,Citation38 On the basis of these findings, the intense repression of S.A.R in PRR5ox seems to be attributed to enhanced accumulation and nuclear localization of TOC1. Contrary to this supposition, in hypocotyl elongation, overexpressors of PRR5 without PRR domain, which cannot interact with TOC1, show extreme hypersensitivity to red light, and prr5 TOC1ox plants are not different from TOC1ox in sensitivity to red light.Citation37,Citation42 From the above, PRR5 is suggested to reduce the amplitude of S.A.R independently of TOC1. Furthermore, this study showed that the hypocotyl elongation and expression of AtHB-2 and HFR1 in PIF5oxPRR5ox plants were intensely repressed as well as those in PRR5ox plants (). This result suggests that PRR5 suppresses S.A.R in the downstream of PIF5 directly or indirectly. Recently, Nakamichi et al. reported that PRR5, as well as PRR9 and PRR7, directly binds to the upstream regions of HFR1 and a C2C2-CO-like transcriptional factor: B-BOX DOMAIN PROTEIN 24 (BBX24), which was reported as an enhancer for S.A.R, and generally acts as a transcriptional repressor.Citation43,Citation44 This can explain the repression of HFR1 and the inhibition of hypocotyl elongation in PRR5ox and PIF5oxPRR5ox plants. However, the mechanism for the repression of AtHB-2 in them and the functional uniqueness of PRR5 in the suppression of S.A.R still remain unclear. Taken together, these findings suggest that PRR5 regulates S.A.R not only by control of periodic expression of PIFs as a clock component but also by direct regulation of the downstream of PIFs in phytochrome-mediated light signaling pathway.
PRR5-regulated leaf-specific S.A.R
Although both leaf blades and petioles are components of eudicot leaves, their morphogenic behaviors in S.A.R differ largely.Citation19,Citation45 According to previous anatomical analyses of leaves in several mutants, leaf blades and petioles can be regarded as different organs.Citation46 In facts, approximately half of the genes induced by E.O.D-FR treatment in leaves are petiole-specific.Citation24 In this study, we found that prr5 mutants showed normal leaf expansion under low R/FR in spite of their extreme petiole elongation, unlike prr9 and prr7 mutants (). The extreme petiole elongation in prr5 mutants under low R/FR can be explained by the above-mentioned function of PRR5 as a negative regulator of S.A.R. On the other hand, the normal leaf expansion in prr5 mutants under low R/FR suggests that PRR5 is involved in the leaf-specific S.A.R. It was previously reported that phytochrome in leaf blades but not that in petioles regulates S.A.R in petioles.Citation24 On the basis of these findings, canopy-shade signal seems to be normally transduced from leaf blades to petioles in prr5 mutants. Further analyses of prr5 mutants will reveal the mechanism of inter-organic growth regulation in S.A.R.
Roles of PRR5 in diurnal regulation of phytochrome-meditated S.A.R
According to previous reports, the expression of PRR5 peaks between middle and end of light period, and the abundance of PRR5 peaks with some lag and is reduced rapidly in darkness by ZEITLUPE (ZTL).Citation40,Citation47 ZTL consists of a LOV domain, a F-box and six Kelch repeats, and interacts with other proteins under influence of light.Citation48-Citation50 The degradation of PRR5 by ZTL is also repressed by blue light.Citation44 On the basis of these findings, it is speculated that PRR5 represses S.A.R from late light period to early dark period. This is consistent with the repression of S.A.R at dusk reported previously.Citation25 Interestingly, it was reported that, in early photomorphogenesis, loss of function mutants of ZTL (ztl) is hyposensitive to red light in spite of their normal sensitivity to blue light.Citation49 These phenomena can be explained that accumulated PRR5 due to absence of ZTL represses phytochrome-meditated S.A.R. Taken together, PRR5 seems to gate phytochrome-meditated S.A.R around dusk and to be one of the cross-talk points between red and blue light signal transduction pathway. The details of PRR5 as a regulator of S.A.R will be revealed by further analyses using artificial induction of PRR5 in various mutants of clock and photomorphogenetic genes.
Materials and Methods
Plant materials and growth conditions
Arabidopsis accession Columbia-0 (Col-0) was used as WT in this study. prr9-10 (SALK_007551), prr7-11 (SALK_030430), prr5-11 (KG24599; from the KAZUSA DNA Research Institute) and their hybrid lines were described previously.Citation27 Overexpressors of PRR9, PRR7 and PRR5 were kind gifts from Dr.Yamashino.Citation38,Citation47 PIF5ox tagged with GFP was a kind gift from Dr.Lorrain.Citation19 phyB-9 was described previously.Citation51 PIF5oxPRR5ox plants were obtained by crossing. PIF5ox was genotyped using GFP fluorescence in root, and PRR5ox was genotyped based on hypocotyl length and the expression of PRR5 detected by RT-PCR. For morphometry and gene expression profiling, seeds were sown on rock wool, and the seedlings were watered with 0.5 g L-1 Hyponex solution (Hyponex, Co. Ltd.). For selection of crossed lines, seeds were sown on Murashige and Skoog (MS) mediumCitation52 with 2% sucrose, solidified with 0.5% Gelann Gum. After 2 d of darkness at 4°C, seedlings were transferred under several light conditions at 23°C in closed growth chambers (LH-80CCFL-DT, NKsystem Co. Ltd.).
Light source and E.O.D-FR treatment
White light was provided by white cold cathode fluorescent lamp (CCFL-1; NKsystem Co. Ltd.). Red, blue and infrared lights were provided by light-emitting diodes (LEDs) at peak wavelengths of 660 nm (LED-mR; Eyela Co. Ltd.), 470 nm (LED-mB; Eyela Co. Ltd.) and 735 nm (LED-mFR; Eyela Co. Ltd.), respectively. High R/FR light means white light (R/FR = 4.2), and Low R/FR light (R/FR = 0.2) was provided by the white light with supplementary FR LEDs. For E.O.D-FR treatment, plants were irradiated with 20 μmol m-2 s-1 PFD of FR for 15 min in the end of light period. For sampling under dark condition, irradiation with green LEDs at peak wavelength of 525 nm (OSPG5161P; Optosupply Co. Ltd.) was used as a working light.
Measurements of leaves and hypocotyls
For leaf measurement, leaves were excised at the basal portion of petiole and placed on white papers with white tape; then images of the leaves were scanned with a scanner. For hypocotyl measurement, seedlings were directly placed on a scanner and imaged. The lengths of hypocotyls, leaf blades and petioles were determined using the software Image J (http://rsb.info.nih.gov/ij/, NIH).
Gene expression profiling
3rd and 4th leaves of 20 d.a.g old plants were excised at the basal portion of petiole and immediately frozen in liquid nitrogen at specific time points. RNA in the frozen samples was extracted using Plant RNA Isolation Reagent (Invitrogen Co. Ltd.), and reverse-transcribed using the PrimeScript Reverse Transcriptase (TAKARA, Co. Ltd.). Quantitative PCR was performed with the 7500 Real-Time PCR System (Applied Biosystems). Each reaction was performed in triplicate using a primer concentration of 0.3 μM. The sequences of the primer sets used in this study are given in Table S1.
Abbreviations: | ||
S.A.R | = | shade avoidance response |
PIF | = | phytochrome Interacting factor |
CCA1 | = | CIRCADIAN CLOCK-ASSOCIATED 1 |
LHY | = | LATE ELONGATED HYPOCOTYL |
TOC1 | = | TIMIMG OF CAB EXPRESSION 1 |
GI | = | GIGANTEA |
PRR | = | PSEUDO RESPONSE REGULATOR |
LD | = | 16 h light/8 h dark cycle |
LL | = | continuous lighting |
ZTL | = | ZEITLUPE |
Additional material
Download Zip (134.8 KB)Acknowledgments
We thank Dr. Lorrain for the gifts of PIF5ox seeds, Dr. Yamashino and Dr. Mizuno for the gifts of the seed of prr mutants and OX lines, Dr. Kozuka for the gifts of the seeds of photoreceptor mutants and advice, Dr. Kiba and Dr. Nakamichi for important information and suggestion, and Dr. Ferjani and Dr. Horiguchi for their kind assistance and advice.
Disclosure of Potential Conflicts of Interest
No potential conflicts of interest were disclosed.
References
- Ahmad M, Cashmore AR. HY4 gene of A. thaliana encodes a protein with characteristics of a blue-light photoreceptor. Nature 1993; 366:162 - 6; http://dx.doi.org/10.1038/366162a0; PMID: 8232555
- Lin C, Ahmad M, Chan J, Cashmore A. CRY2, a second member of the Arabidopsis cryptochrome gene family. Plant Physiol 1996; 110:1047 - 52; PMID: 8819875
- Franklin KA, Praekelt U, Stoddart WM, Billingham OE, Halliday KJ, Whitelam GC. Phytochromes B, D, and E act redundantly to control multiple physiological responses in Arabidopsis. Plant Physiol 2003; 131:1340 - 6; http://dx.doi.org/10.1104/pp.102.015487; PMID: 12644683
- Chen M, Chory J, Fankhauser C. Light signal transduction in higher plants. Annu Rev Genet 2004; 38:87 - 117; http://dx.doi.org/10.1146/annurev.genet.38.072902.092259; PMID: 15568973
- Sharrock RA, Quail PH. Novel phytochrome sequences in Arabidopsis thaliana: structure, evolution, and differential expression of a plant regulatory photoreceptor family. Genes Dev 1989; 3:1745 - 57; http://dx.doi.org/10.1101/gad.3.11.1745; PMID: 2606345
- Clack T, Mathews S, Sharrock RA. The phytochrome apoprotein family in Arabidopsis is encoded by five genes: the sequences and expression of PHYD and PHYE. Plant Mol Biol 1994; 25:413 - 27; http://dx.doi.org/10.1007/BF00043870; PMID: 8049367
- Devlin PF, Patel SR, Whitelam GC. Phytochrome E influences internode elongation and flowering time in Arabidopsis. Plant Cell 1998; 10:1479 - 87; PMID: 9724694
- Lin C, Yang H, Guo H, Mockler T, Chen J, Cashmore AR. Enhancement of blue-light sensitivity of Arabidopsis seedlings by a blue light receptor cryptochrome 2. Proc Natl Acad Sci U S A 1998; 95:2686 - 90; http://dx.doi.org/10.1073/pnas.95.5.2686; PMID: 9482948
- Neff MM, Fankhauser C, Chory J. Light: an indicator of time and place. Genes Dev 2000; 14:257 - 71; PMID: 10673498
- Zhao X, Yu X, Foo E, Symons GM, Lopez J, Bendehakkalu KT, et al. A study of gibberellin homeostasis and cryptochrome-mediated blue light inhibition of hypocotyl elongation. Plant Physiol 2007; 145:106 - 18; http://dx.doi.org/10.1104/pp.107.099838; PMID: 17644628
- Franklin KA, Whitelam GC. Light signals, phytochromes and cross-talk with other environmental cues. J Exp Bot 2004; 55:271 - 6; http://dx.doi.org/10.1093/jxb/erh026; PMID: 14673030
- Kircher S, Gil P, Kozma-Bognár L, Fejes E, Speth V, Husselstein-Muller T, et al. Nucleocytoplasmic partitioning of the plant photoreceptors phytochrome A, B, C, D, and E is regulated differentially by light and exhibits a diurnal rhythm. Plant Cell 2002; 14:1541 - 55; http://dx.doi.org/10.1105/tpc.001156; PMID: 12119373
- Khanna R, Huq E, Kikis EA, Al-Sady B, Lanzatella C, Quail PH. A novel molecular recognition motif necessary for targeting photoactivated phytochrome signaling to specific basic helix-loop-helix transcription factors. Plant Cell 2004; 16:3033 - 44; http://dx.doi.org/10.1105/tpc.104.025643; PMID: 15486100
- Leivar P, Tepperman JM, Cohn MM, Monte E, Al-Sady B, Erickson E, et al. Dynamic antagonism between phytochromes and PIF family basic helix-loop-helix factors induces selective reciprocal responses to light and shade in a rapidly responsive transcriptional network in Arabidopsis. Plant Cell 2012; 24:1398 - 419; http://dx.doi.org/10.1105/tpc.112.095711; PMID: 22517317
- Shin J, Kim K, Kang H, Zulfugarov IS, Bae G, Lee CH, et al. Phytochromes promote seedling light responses by inhibiting four negatively-acting phytochrome-interacting factors. Proc Natl Acad Sci U S A 2009; 106:7660 - 5; http://dx.doi.org/10.1073/pnas.0812219106; PMID: 19380720
- Huq E, Quail PH. PIF4, a phytochrome-interacting bHLH factor, functions as a negative regulator of phytochrome B signaling in Arabidopsis. EMBO J 2002; 21:2441 - 50; http://dx.doi.org/10.1093/emboj/21.10.2441; PMID: 12006496
- Fujimori T, Yamashino T, Kato T, Mizuno T. Circadian-controlled basic/helix-loop-helix factor, PIL6, implicated in light-signal transduction in Arabidopsis thaliana. Plant Cell Physiol 2004; 45:1078 - 86; http://dx.doi.org/10.1093/pcp/pch124; PMID: 15356333
- Park E, Kim J, Lee Y, Shin J, Oh E, Chung WI, et al. Degradation of phytochrome interacting factor 3 in phytochrome-mediated light signaling. Plant Cell Physiol 2004; 45:968 - 75; http://dx.doi.org/10.1093/pcp/pch125; PMID: 15356322
- Lorrain S, Allen T, Duek PD, Whitelam GC, Fankhauser C. Phytochrome-mediated inhibition of shade avoidance involves degradation of growth-promoting bHLH transcription factors. Plant J 2008; 53:312 - 23; http://dx.doi.org/10.1111/j.1365-313X.2007.03341.x; PMID: 18047474
- Hornitschek P, Lorrain S, Zoete V, Michielin O, Fankhauser C. Inhibition of the shade avoidance response by formation of non-DNA binding bHLH heterodimers. EMBO J 2009; 28:3893 - 902; http://dx.doi.org/10.1038/emboj.2009.306; PMID: 19851283
- Kunihiro A, Yamashino T, Nakamichi N, Niwa Y, Nakanishi H, Mizuno T. Phytochrome-interacting factor 4 and 5 (PIF4 and PIF5) activate the homeobox ATHB2 and auxin-inducible IAA29 genes in the coincidence mechanism underlying photoperiodic control of plant growth of Arabidopsis thaliana. Plant Cell Physiol 2011; 52:1315 - 29; http://dx.doi.org/10.1093/pcp/pcr076; PMID: 21666227
- Hornitschek P, Kohnen MV, Lorrain S, Rougemont J, Ljung K, López-Vidriero I, et al. Phytochrome interacting factors 4 and 5 control seedling growth in changing light conditions by directly controlling auxin signaling. Plant J 2012; 71:699 - 711; http://dx.doi.org/10.1111/j.1365-313X.2012.05033.x; PMID: 22536829
- Roig-Villanova I, Bou J, Sorin C, Devlin PF, Martínez-García JF. Identification of primary target genes of phytochrome signaling. Early transcriptional control during shade avoidance responses in Arabidopsis. Plant Physiol 2006; 141:85 - 96; http://dx.doi.org/10.1104/pp.105.076331; PMID: 16565297
- Kozuka T, Kobayashi J, Horiguchi G, Demura T, Sakakibara H, Tsukaya H, et al. Involvement of auxin and brassinosteroid in the regulation of petiole elongation under the shade. Plant Physiol 2010; 153:1608 - 18; http://dx.doi.org/10.1104/pp.110.156802; PMID: 20538889
- Salter MG, Franklin KA, Whitelam GC. Gating of the rapid shade-avoidance response by the circadian clock in plants. Nature 2003; 426:680 - 3; http://dx.doi.org/10.1038/nature02174; PMID: 14668869
- Sellaro R, Pacín M, Casal JJ. Diurnal dependence of growth responses to shade in Arabidopsis: role of hormone, clock, and light signaling. Mol Plant 2012; 5:619 - 28; http://dx.doi.org/10.1093/mp/ssr122; PMID: 22311777
- Nakamichi N, Kita M, Ito S, Yamashino T, Mizuno T. PSEUDO-RESPONSE REGULATORS, PRR9, PRR7 and PRR5, together play essential roles close to the circadian clock of Arabidopsis thaliana. Plant Cell Physiol 2005; 46:686 - 98; http://dx.doi.org/10.1093/pcp/pci086; PMID: 15767265
- Nozue K, Covington MF, Duek PD, Lorrain S, Fankhauser C, Harmer SL, et al. Rhythmic growth explained by coincidence between internal and external cues. Nature 2007; 448:358 - 61; http://dx.doi.org/10.1038/nature05946; PMID: 17589502
- Farré EM. The regulation of plant growth by the circadian clock. Plant Biol (Stuttg) 2012; 14:401 - 10; http://dx.doi.org/10.1111/j.1438-8677.2011.00548.x; PMID: 22284304
- Yamashino T, Matsushika A, Fujimori T, Sato S, Kato T, Tabata S, et al. A Link between circadian-controlled bHLH factors and the APRR1/TOC1 quintet in Arabidopsis thaliana. Plant Cell Physiol 2003; 44:619 - 29; http://dx.doi.org/10.1093/pcp/pcg078; PMID: 12826627
- Niinuma K, Nakamichi N, Miyata K, Mizuno T, Kamada H, Mizoguchi T. Roles of Arabidopsis PSEUDO-RESPONSE REGULATOR (PRR) genes in the opposite controls of flowering and organ elongation under long-day and continuous light conditions. Plant Biotechnol 2008; 25:165 - 72; http://dx.doi.org/10.5511/plantbiotechnology.25.165
- Djakovic-Petrovic T, de Wit M, Voesenek LA, Pierik R. DELLA protein function in growth responses to canopy signals. Plant J 2007; 51:117 - 26; http://dx.doi.org/10.1111/j.1365-313X.2007.03122.x; PMID: 17488236
- Cole B, Kay SA, Chory J. Automated analysis of hypocotyl growth dynamics during shade avoidance in Arabidopsis. Plant J 2011; 65:991 - 1000; http://dx.doi.org/10.1111/j.1365-313X.2010.04476.x; PMID: 21288269
- Michael TP, Salomé PA, Yu HJ, Spencer TR, Sharp EL, McPeek MA, et al. Enhanced fitness conferred by naturally occurring variation in the circadian clock. Science 2003; 302:1049 - 53; http://dx.doi.org/10.1126/science.1082971; PMID: 14605371
- Furuya M. Molecular properties and biogenesis of phytochrome I and II. Adv Biophys 1989; 25:133 - 67; http://dx.doi.org/10.1016/0065-227X(89)90006-3; PMID: 2696339
- Carabelli M, Morelli G, Whitelam G, Ruberti I. Twilight-zone and canopy shade induction of the Athb-2 homeobox gene in green plants. Proc Natl Acad Sci U S A 1996; 93:3530 - 5; http://dx.doi.org/10.1073/pnas.93.8.3530; PMID: 11607652
- Ito S, Matsushika A, Yamada H, Sato S, Kato T, Tabata S, et al. Characterization of the APRR9 pseudo-response regulator belonging to the APRR1/TOC1 quintet in Arabidopsis thaliana. Plant Cell Physiol 2003; 44:1237 - 45; http://dx.doi.org/10.1093/pcp/pcg136; PMID: 14634162
- Matsushika A, Kawamura M, Nakamura Y, Kato T, Murakami M, Yamashino T, et al. Characterization of circadian-associated pseudo-response regulators: II. The function of PRR5 and its molecular dissection in Arabidopsis thaliana. Biosci Biotechnol Biochem 2007; 71:535 - 44; http://dx.doi.org/10.1271/bbb.60584; PMID: 17284847
- Nakamichi N, Kita M, Ito S, Sato E, Yamashino T, Mizuno T. The Arabidopsis pseudo-response regulators, PRR5 and PRR7, coordinately play essential roles for circadian clock function. Plant Cell Physiol 2005; 46:609 - 19; http://dx.doi.org/10.1093/pcp/pci061; PMID: 15695441
- Nakamichi N, Kiba T, Henriques R, Mizuno T, Chua NH, Sakakibara H. PSEUDO-RESPONSE REGULATORS 9, 7, and 5 are transcriptional repressors in the Arabidopsis circadian clock. Plant Cell 2010; 22:594 - 605; http://dx.doi.org/10.1105/tpc.109.072892; PMID: 20233950
- Wang L, Fujiwara S, Somers DE. PRR5 regulates phosphorylation, nuclear import and subnuclear localization of TOC1 in the Arabidopsis circadian clock. EMBO J 2010; 29:1903 - 15; http://dx.doi.org/10.1038/emboj.2010.76; PMID: 20407420
- Ito S, Niwa Y, Nakamichi N, Kawamura H, Yamashino T, Mizuno T. Insight into missing genetic links between two evening-expressed pseudo-response regulator genes TOC1 and PRR5 in the circadian clock-controlled circuitry in Arabidopsis thaliana. Plant Cell Physiol 2008; 49:201 - 13; http://dx.doi.org/10.1093/pcp/pcm178; PMID: 18178585
- Nakamichi N, Kiba T, Kamioka M, Suzuki T, Yamashino T, Higashiyama T, et al. Transcriptional repressor PRR5 directly regulates clock-output pathways. Proc Natl Acad Sci U S A 2012; 109:17123 - 8; http://dx.doi.org/10.1073/pnas.1205156109; PMID: 23027938
- Crocco CD, Holm M, Yanovsky MJ, Botto JF. Function of B-BOX under shade. Plant Signal Behav 2011; 6:101 - 4; http://dx.doi.org/10.4161/psb.6.1.14185; PMID: 21301219
- Goto N, Kumagai T, Koornneef M. Flowering responses to light-breaks in photomorphogenic mutants of Arabidopsis thaliana, a long-day plant. Physiol Plant 1991; 83:209 - 15; http://dx.doi.org/10.1111/j.1399-3054.1991.tb02144.x
- Tsukaya H, Kozuka T, Kim GT. Genetic control of petiole length in Arabidopsis thaliana. Plant Cell Physiol 2002; 43:1221 - 8; http://dx.doi.org/10.1093/pcp/pcf147; PMID: 12407202
- Kiba T, Henriques R, Sakakibara H, Chua NH. Targeted degradation of PSEUDO-RESPONSE REGULATOR5 by an SCFZTL complex regulates clock function and photomorphogenesis in Arabidopsis thaliana. Plant Cell 2007; 19:2516 - 30; http://dx.doi.org/10.1105/tpc.107.053033; PMID: 17693530
- Somers DE, Schultz TF, Milnamow M, Kay SA. ZEITLUPE encodes a novel clock-associated PAS protein from Arabidopsis. Cell 2000; 101:319 - 29; http://dx.doi.org/10.1016/S0092-8674(00)80841-7; PMID: 10847686
- Somers DE, Kim WY, Geng R. The F-box protein ZEITLUPE confers dosage-dependent control on the circadian clock, photomorphogenesis, and flowering time. Plant Cell 2004; 16:769 - 82; http://dx.doi.org/10.1105/tpc.016808; PMID: 14973171
- Kim WY, Fujiwara S, Suh SS, Kim J, Kim Y, Han L, et al. ZEITLUPE is a circadian photoreceptor stabilized by GIGANTEA in blue light. Nature 2007; 449:356 - 60; http://dx.doi.org/10.1038/nature06132; PMID: 17704763
- Reed JW, Nagpal P, Poole DS, Furuya M, Chory J. Mutations in the gene for the red/far-red light receptor phytochrome B alter cell elongation and physiological responses throughout Arabidopsis development. Plant Cell 1993; 5:147 - 57; PMID: 8453299
- Murashige T, Skoog F. A revised medium for rapid growth and bioassays with tobacco tissue cultures. Physiol Plant 1962; 15:473 - 97; http://dx.doi.org/10.1111/j.1399-3054.1962.tb08052.x