Abstract
Taking as a basis of discussion Kalanchoe’s spontaneous and evoked extracellular activities recorded at the whole plant level, we put the challenging questions: do these low-voltage variations, together with endocellular events, reflect integrative properties and complex behavior in plants? Does it reflect common perceptive systems in animal and plant species? Is the ability of plants to treat short-term variations and information transfer without nervous system relevant? Is a protoneural construction of the world by lower organisms possible? More generally, the aim of this paper is to reevaluate the probably underestimated role of plant surface potentials in the plant relation life, carefully comparing the biogenesis of both animal and plant organisms in the era of plant neurobiology. Knowing that surface potentials participate at least to morphogenesis, cell to cell coupling, long distance transmission and transduction of stimuli, some hypothesis are given indicating that plants have to be studied as environmental biosensors and non linear dynamic systems able to detect transitional states between perception and response to stimuli. This study is conducted in the frame of the “plasticity paradigm,” which gives a theoretical model of evolutionary processes and suggests some hypothesis about the nature of complexity, information and behavior.
Plant neurobiology, as a new field of plant sciences, has clearly shown that following the pioneering discoveries about bioelectricity made by Darwin, Galvani, Burdon Sanderson or Pfeffer at the end of the XVIII century and then by Bose at the beginning of the XX century,Citation1 a floating period, aggravated by the Backster’s syndrome of the 1970s,Citation2 had heavy consequences on plant electrophysiological research, particularly at the extracellular level.Citation3,Citation4 Actually, if the mechanistic analogies between the neuronal action potentials and the electromotive pulvinar activities of Mimosa Pudica detected by Bose were clarified by Ricca and other numerous authors succeeding him, the ex-CIA agent Backster’s pseudoscientific use of a lie detector to record some “hyper-reactivity to stress” in plants, induced a sustainable confusion. Indeed, following this publication, plant electrophysiologists neglected during a long time coherent data concerning surface potentials or extracellular recordings made at the level of polarized groups of plant cells or tissues, being focused on the understanding of the genesis of endocellular events until patch-clamp technologies applied to plants demonstrated that the repertory of ionic channels of plant membranes was quite similar to that of animal cells.Citation5-Citation8 Another reason explaining this indifference about low voltage spontaneous activities or evoked responses to stimuli recorded at the level of different plant organs was that the functional role of these bioelectrical signals in the plant relation life was unclear. Our work, initiated at the end of the 1970s and published in 1992, showed in this context that extracellular spontaneous surface potential variations (ESV) or electrophytographic activities (EPG), as well as evoked bursts of spikes recorded at the level of leave or roots occur widely in plant tissues.Citation9 These basic microvolted activities—at that time called “surface potentials”—were correlated with classical intracellular action potentials (APs) and proposed to represent resulting macroscopic currents locally sustained by plant receptor-channels acting through the different membrane compartments and globally expressed as EPG derivations of synchronized “protoneural networks” diffusing information at the whole plant level.
As other few contemporaneous studies devoted to the same approach in the seventies,Citation10-Citation12 particularly those of Pickard (1972, 1973) who classified these recordings as spontaneous activity and putative APs reflecting endocellular activities, the physiological role of these potentials was attributed to a possible control of release of chemical agents or linked to pinocytosis or extrusion of vesicles.Citation13,Citation14 Other hypotheses to support these microvolted extracellular activities were the facilitation of long distance transmission signals or local osmotic regulation.Citation15 Moreover, if the similar behavior of plant and animal kingdoms was sensed in all these studies, the clear stake was during this period to prove that APs and excitability were of wide occurrence and linked to co-ordination in higher as ordinary plants. Indeed, the neuroïd conduction was then extensively explored at the cellular level, first showing neighboring properties compared with Hodgkin and Huxley equations for nerve fibers (1952) i.e., conduction speeds reaching those of type A nervous fibers (10−2m.s−1), but absolute refractory period from 20 to 60 times longer than in neurons.Citation16 The rapid motricity of Mimosa pudica was then demonstrated by Sibaoka and Stoeckel as due to parenchymatous excitable cells where propagated APs precede cell deturgescence and the motor phenomenon itself.Citation17-Citation19 Furthermore, Hedrich’s et al. later patch-clamp studies of ionic channels of plasmic or vacuolar membranes from higher or ordinary plant cells showed calcium voltage-dependent channels or gap junctions similar to epithelial cells or central mammalian neurons studies and more specific mechanisms involving electrogenic pumps using ATP or mechanosenstive channels that could act for Mimosa pudica motor cells.Citation20-Citation22
Many more recent data about bioelectrical or information-processing data, long-distance communication, phenoplasticity, morphogenesis, learning, self-recognition or complex adaptive behaviors are reviewed in the paper. They show that, in addition to classical intracellular activities, low voltage potential variations recorded at the level of group of polarized cells or tissues could participate to the fine regulation of the major physiological functions of plants and ask the question of a centralized (or multi-centralized) integration of these activities at the whole plant level? In spite of this knowledge, plant scientists stood back until the plant neurobiology revolution emerged at the first symposium dedicated to this paradigm at Firenze in 2005,Citation23 following Trewavas revisited definition of plant prototypical “intelligence.”Citation24 Our aim is here: (1) to exemplify, through our earlier study of low “surface electrical currents" (or field potentials) recorded in Kalanchoe’s tissues, the proximity and/or the convergence of both plant and animal integrated bio-systems conducting to common protoneural dynamic behaviors; (2) to revalue these researches and the advances which they could engender in the light of the new plant neurobiology field, a transdisciplinary one.
Experimental Results on Kalanchoe’s Spontaneous and Evoked Extracellular Potential Variations
The data we are taking as a basis of discussion are electrophysiological measurements showing that different patterns of low voltage spontaneous and evoked activity occur widely in ordinary plants like Kalanchoë daigremontiana and tubiflora.Citation25 These patterns have essentially been divided into three typical classes: fluctuant mono- or polymorph activity, relative electrical silence periods and spike potentials ( and ). All these basic spontaneous activities were recorded in the low microvolt range (10 to 300 µV) and were expressed as slow waves of low frequency (< 5 Hz) broken by solitary or small bursts of spike potentials (200 µV to 1 mV) and relative electrical silence or RES periods lasting from 5 sec to some hours, probably linked to metabolism or nyctemeral cycles ().
Figure 1. Spontaneous and evoked extracellular responses in Kalanchoë daigremontiana. This figure shows spontaneous microvolted potential variations constituted by FPA activity and some isolated spikes recorded in the stem of Kalanchoë daigremontiana plantlets (A, platinum wires), followed by evoked trains of putative APs. Indeed, burst of spikes were induced by a paired mechanical stimulus consisting of local application of distilled water and 3 small pricks made by glass micropipettes (10 µm diameter at the head) at the same location and during the same time (wounding stimuli). As shown by the traces (B–E), once the stimulation applied (36 sec after), we observed the supervening of some isolated putative APs initiating a burst of spikes during 1.50 min broken by RES periods (G, 8–36 sec). After 6.50 min of RES period, a big spike initiated a burst of very short time-rise APs (< 10 msec) followed at the end of the scope trace by a slower solitary spike (0.5 sec). Except this one, spikes were +/−, 300 µV-3 mV, 350 msec and the total duration of the discharge was 21 min. Kalanchoë tissue remains excitable in that case, showing no fatigue and a good recovery of basic FPA activity (H).
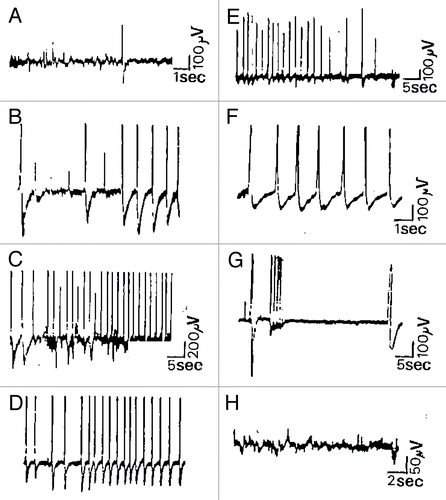
Figure 2. Chemical activation of Kalanchoë Daigremontiana stem tissues. (A) recording of spontaneous spikelets 5 min before FC activation. FC activation responses occurred 7 min after the local FC deposition (3.7 µM, n = 4) between both recording electrodes as trains of −/+ spikes in which the rising phase is 1/3 of the descending one (B1 and B2). This corresponds to classical hyperpolarizations observed in mammalian cells linked to calcium dependent K+ conductance. AP duration ranged from 300–500 msec for the fastest, and up to 2 sec for the slowest, with maximal amplitudes of 3 mV, and separation intervals from 2–16 sec Return to the baseline ranged from 0.25–2.5 sec depending on the duration of the repolarizing phase. The total duration of this burst was 27 min and it was immediately interrupted by application of FCCP 50 µM at the same site (C1, n = 2). However, no restoration was seen, even after 2 h recording, which was not the case for other metabolic inhibitors like 2–4-DNP (100 µM) or low temperature (0°C) that show partial or total recovery, possibly due to the capacity of the tissue to more easily recover from a block of ATP (not shown). Concerning the Material and Methods (electrophysiological set-up, soil culture conditions, temperature and humidity %, stimulating procedure, elimination of interference signals by Faraday cage, correlated methods, use of several kind of electrodes including Ag-AgCl probes, controls made with salt bridges during several hours etc...), please refer to Debono and Bouteau (1992) referenced in Citation9.
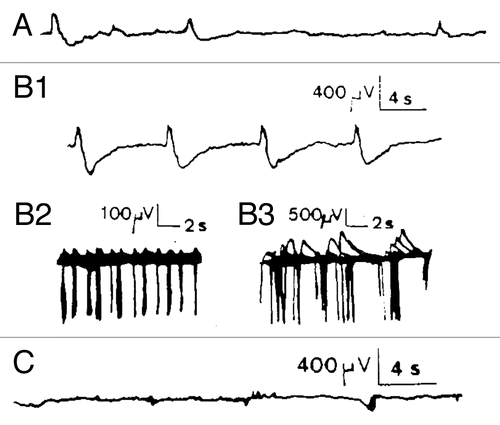
Moreover, typical patterns of evoked spike potentials (isolated or in burst) have been obtained in the microvolt range following exogenous mechanical or chemical stimuli, some of them being well correlated with transmembrane potentials using simultaneous two scale recordings (not shown). The fast spikes recorded after a mechanical stimulation (local deposition of a drop of distilled water, 50 µl) may have rise times of less than 50 msec and duration from 100 to 500 msec. They often constitute trains of APs including fatigue phenomena resembling those at vertebrate peripheral nerves or not, depending of the stimulus (, b-g). Similar trains of fast rise putative APs during 9–12 min, including irregular bursting and RES periods were also recorded in Kalanchoë tubiflora (not shown). Chemical stimulation (use of the activator of H+ excretion pump FC) induced also bursts of APs. with a total duration of about 30 min that were interrupted by application of the protonophore FCCP at the same site (). Like FCCP, lowering temperature or the use of metabolism inhibitors such as DNP abolished excitation, but they show partial or total recovery, which was not the case for FCCP, possibly due to the capacity of the tissue to more easily recover from a block of ATP (not shown). The complex nature of these microvolted potentials and their coherent responses to metabolism agents suggest that H+ electrogenic pumps, and at least K+ and Ca2+ receptor channels are involved in such responses. However, their precise role has to be clearly investigated in the light of recent works in plants’ bioelectricity, particularly at local electric fields and network activities recorded at the level of tissues.
What Could Be The Precise Role Of Extracellular Activities Recorded in Polarized Groups of Plant Cells or Tissues?
To answer this question, it is necessary to do first a brief step backward concerning macroscopic or endocellular currents involved in the activation of a variety of receptor channels of the plant cell membrane. Indeed, well known anionic (voltage-dependent chloride channels) or cationic (potassium or calcium-activated potassium channels) channels participate in all basic physiological functions of plants such as volume or pressure regulation (stretch activated ion-channels), signal transduction and growth or hormonal driven stomatal closing.Citation26-Citation28 Transduction of particular stimuli is always associated with the functional expression of these channels. For instance, we know that the pea epicotyl friction through the soil induces an AP linked to ethylene production whereas polar regeneration transduces an electrical stimulus in Acetabularia.Citation29 For Dionea muscipula, a well-known carnivorous plant, the rapid closure of the trap was clearly associated with the previous emission of an AP. In that case, the conduction speed reach 200 mm/sec−1 and the rise time of the AP is 0.1 sec In Berberis vulgaris, a mechanical stimulus induces a movement of the bunting, preceded for 1 to 2 sec by an AP. Desbiez has clearly shown the link between the transmission of a traumatic signal (small pricks) via cell depolarization and the induction of correlation between cotyledonary buds of Bidens pilosus (morphogenetic efficacy).Citation30
Furthermore, recent progress permits now, as for animal cells, to clone plant ionic channels or recombinant subunits of these channels and to express them in vitro (injection of cDNA, mRNAs and recombinant receptor subunits in Xenopus oocytes.Citation31 A lot of other mechanisms involving electrically excitable plant cells are described throughout the text. Contrarily to intracellular currents, only some basic electrical phenomena are described concerning low-voltage or “microscopic” currents in the literature. It is nevertheless the case for surface local electrostatic fields surrounding calcium activated potassium channels which can influence ion permeation characteristics of the channel proteinCitation32 or endogenous fields and cellular dipoles during tip growth of root hairs or pollen tubes.Citation33,Citation34 These positive tip potentials are partially attributed to localized calcium influx mediated by electrophoretic or cytoskeletal mechanisms.Citation35 Calcium waves are also implicated in cytoplasmic movements, induction of stomatal closing by hormonal mediationCitation36 and identified morphogens which include biophysical, electrical, mechanical or gravitational forces.Citation37 Such ionic mechanisms coupled with voltage gradients participate to morphogenesis, cell to cell coupling and transcellular fields.
As a consequence, surface charge density factors, calcium waves and cell-to-cell communication could be both directly or indirectly related to the extracellular microvolted potential variations or surface electrical currents that we have recorded at the level of polarized groups of cells or tissues. These cellular events, possibly using mainly calcium as intracellular as cytoplasmic messenger and local field potentials, are suitable to produce small surface currents carried by ion fluxes (hydrogen, calcium, potassium…), flowing across the different cell membranes, using enzymes and being amplified at the level of plant organs, are able to play key roles for the regulation of plant development and reactivity to stimuli. However, their precise functional role in all these processes remains unclear and has not really been studied until today. Whatever their location (to be studied and probably situated at specialized tissues: see farther), they may contribute, as macroscopic current responses, to control and/or facilitate transduction of many stimuli. For instance, they could be implicated in regulating the direction of growth of rhizoid structures, as shown by the pioneering work of Lund (1947) on bioelectric fields and growth, or be involved in the generation of variation potentials during perception of low electric fields such as those linked to hydraulic or atmospheric pressure. Indeed, Goldworthy realized long-term measurements around individual tobacco cells growing in culture with vibrating probes showing the presence of micro-currents (as low as 0.1 µA/cm2) which are able to change their own current patterns in order to be in line with external applied currents (an order of magnitude bigger than the inner currents).Citation38
These results suggest that plant cells may act as electrosensors when thunderstorms are arriving (the magnitude of the currents are compatible) with the relay of calcium acting as well as cellular messenger, voltage-gating ion and growth factor. When a large amount of cells are polarized, intercellular communication is enhanced and cooperation is effective at the tissue level to increase growth rates of shoots or to respond to exogenous stimuli. More precisely, plasma membrane polarity activates many intracellular sorting events (transport of proteins and lipids) probably involving specialized compartments at the intersection of the apical and basolateral endocytic pathways.Citation39 Up to date reviews concerning plasma and dipole membrane surface potentials or densities and ion-plant or toxin interactions or plant cell wall electrical potentials were recently done by Wang or Kinraide.Citation40,Citation41 They show the complexity of electrostatic interactions and structural constraints linked to protein transport or ion uptake and their impact on the generation of surface, transmembrane and dipole potentials.
If we come back now to extracellular spontaneous or evoked activities recorded at the level of plant tissues, these three kinds of membrane cell-surface potentials integrating complex molecular-dynamics designs affect probably the bioelectrical derivatives recorded at the level of multicellular organs. A first step to better understand the role of the ESVs that we have recorded at Kalanchoë leaves and stems will so be not to consider the integrative mechanisms themselves, but their functional effectiveness. In the case of propagated APs, it is easy to correlate them to precise motor or sensitive functions. For microvolted ESVs, they could underlie growth, gravity or transcellular communication and act as electrosensors, however, to my knowledge, no clear link are established in term of function relatively to the whole plant relation life, due to scattered and uncoordinated researches. Our hypothesis is that they could reflect, as for animal cells, a macroscopic derivation of electrogenic tissue activities (like EKG, EMG or EEGs represent the algebraic summation of a great population of cardiac, muscular or neuronal cells) and the ability of plants to react acutely and sometimes rapidly to micro-environmental changes. Indeed, our findings, suggest that ESVs are concomitant of several intracellular activities of plants that could be synchronized and propagated at one or several organic loci. Furthermore, they may have precise roles as for detecting small energies and contribute, by their own electrochemical properties, or synchronous electrical networks, to control growth, perception of stimuli and integrated bio-signals permitting better developmental adaptations. We will see further in the discussion that recent technologies permitting to monitor spontaneous and evoked activities at several levels of a same plant tissue were able to confirm our hypothesis and could boost the study of ESVs to there stayed in the shadow.
Common Signaling and Transducing Pathways Used by Biological Systems: Evolutionary Processes and Non Linear Dynamics
All these data taken together clearly show that electrical activity is a general property of excitable cells, and is not restricted to nerve structures. Moreover, if it is an evidence to assert that neurons used electricity long after plants, the fact of considering any kind of tropisms or sensory transduction including recent discoveries about proprioceptive sensing,Citation42 phenotype plasticity, fast motor reactivity, cell memory, morphogenesis, self/non-self recognition, high sensitivity to environmental changes, stress-induced responses, plant-plant and insect-plant interactions, cognitive abilities and behaviors,Citation43 lead us irreparably to ask the question of a convergence of expression before or juxtaposed to the divergence of evolution both animal and plant kingdoms? This hypothesis is supported by two main discoveries: (1) the existence of four trunks of plant complex organisms showing nuclear structures and a weak branch separation from animal species;Citation44 (2) the existence in plants of a lot of gene receptor superfamilies with a strong structural analogy to animal receptor proteins (endogenous synthesized neuroactive molecules like glutamate, glycine or GABA typically found in the CNS of animal and human brains), but also hormones, calcium binding proteins and synaptotagmins, both arguments going for Chiu et al. (1999) in the direction of a primitive signaling mechanism existing before the divergence of plants and animals.Citation45
Our results are comforted by these discoveries suggesting that the non-nervous system of plants was a prototypic phase (a protoneural one) nonlinearly conducting to the fast synaptic transmission of neurons. However, the question remains to understand how living systems apparently lacking highly centralized structures have developed such bioelectrical profiles correlated with complex behaviors in plants? We think that global exchange or integrative centers (ICs) constituted by clusters synchronizing both basic ESVs and field potentials (as well as intercellular and then plant-to-plant or insect-plant activities) could be present at the whole plant level. Indeed, although no integrative center is now unmistakably identified, several structures such as cytoskeletal membrane proteins and calcium voltage dependent channels at the cellular level, and richly interconnected zones like roots’ phloem, xylem or apical meristem constituting rich symplasmic fields or sensori-motor structures (like pulvini, traps, stomata or growth cone) are likely to contribute to the integrative properties of plants. Baluška et al.Citation46 identified more specifically root apices as a possible “brain like” command center having ramifications (transition zones), plant synapses transporting auxin and a rich vascular tissue network permitting high rate transmission of information. Moreover, plant synapses were shown to use actin-based domains for cell-to-cell communication presenting analogies with the immunological concept of self demonstrated in animal neurons.Citation47 These end-poles of root cells transporting hormonal transmitter, supporting long-distance communication and acting like brain synapses are therefore serious candidates to centralization. An open question would however be to know if it excludes several interactive loci of information? All these discoveries should so be studied within the framework of living system plasticity and a transdisciplinary approach of nonlinear dynamic systems like ICs in evolutionary processes. The main observation is that such sensitivity and complexity in “simpler organisms” can only open new research fields about the nature of perception, access to experience, and downstream, that of cognitive and conscious processes.Citation48
Light- or Mechanical-Induced Signals and Morphogenesis
Now, it is not surprising that excitable plant cells can exhibit the same complex bioelectrical profiles as animals, knowing the universal use of electric fields and the role of complexity during evolution. Indeed, if the complexity process is undeniable, symbiotic and co-evolutionary motors in the lower part of the phylogenetic scale could accompany it. An interesting example of symbiosis of ligand and voltage-gated anion channels in plant and animal cells was reported by Hedrich and Jeromin (1992) where the anion channels are directly affected by growth hormones like auxin.Citation49 Concerning morphogenetic expression, calmodulin gene expression is involved after wounding stimuli of Bidens pilosa (with slow-waves electrical responses as long-distance transmitters for wound signals) whereas mRNA is only locally accumulated.Citation50 Morphogenetic signals of the shoots of apical meristems cells are interconnected by plasmodesmata form symplasmic paths.Citation51 The symplasmic paths of the tunica are subdivided and play a role in intercommunication of cells, with boundary interactions between them (as for glia-neurones interactions) and protection of the distal meristem cells from organogenesis. They are assimilated here to metabolic working units co-coordinating morphogenetic fields, or able to regulate essential developmental functions following Davies that has also shown that action and variation potentials induce proteinase inhibitor gene expression in tomato plants.Citation52
In other cases, proton pumps regulate light-induced oscillations of membrane potentials.Citation53 Specialized structures such as thylacoïd inside plant chloroplasts constitute light-stimulated single electric units containing more than 2.108 chlorophyll molecules in an area of more than 400 µ2.Citation54 Endogenous large electric fields have also been described to accompany many polarized tip-growing cells such as algal rhizoids or root hairsCitation55 or traversing growing pollen tubes,Citation56 whereas many studies show that exogenous 60 Hz electric fields are able to act upon the cells through an alteration of transmembrane potentials.Citation57 Electrical, but also hormonal and hydraulic signals are known to modulate gene expression through transcription and translation via calcium-dependent cytoskeleton-protein-channels.Citation58 Beyond voltage-dependent ionic currents from excitable cells (cytosolic calcium waves are similar to those of animal cells), many examples of electrocoupling of transporters involving nonlinear oscillations of dynamic systems and providing long-term osmotic regulation are available like in the guard cells of the plasmalemma of certain plants.Citation59 Gradmann presented in that way different models simulating the oscillations linked to enzymes and ionic channels affecting the membrane voltage of plants.Citation60
At the whole plant level, many sensitive systems are also classically described, from which that of Dioanea muscipula (“moon trap”) and Brionnea that are excellent examples of fast motor activity and strong and fast thigmoreactivity to touch, respectively. Bauer et al. equally show that common basic mechanisms like cell calcium oscillations were probably developed before the divergence of plant and animal cells.Citation61 More generally, the evolutionary conservation of common developmental features or gene structure for members of superfamilies of proteins present in animals and plants are well documented.Citation62 For instance, common mechanism of developmental light-dependent phenomena exists in mammalian and plant systems. Pleiotropic protein like the key repressor COP1 regulating the photomorphogenic development interrelations are for instance used by plant species like Arabidopsis as well as by humans.Citation63 This family of transcription factors regulating the nuclear and subcellular localization of proteins is a highly conserved regulatory system.
Ubiquitous Proteins, Globin- and Actin- Encoding Gene Families
It is the same case for other family of transcription factors common in vertebrates and invertebrates or the control of cell-surface proteins, particularly the downregulation of plasma membrane proteins like channels or transporters by ubiquitins.Citation64 As a matter of fact, markers like ubiquitous proteins or hormones have a common pathway before being expressed in many species including animals and plants. For instance, GF14-3-3 brain protein homologs are present in Arabidopsis thaliana, where a high degree of protein sequence is apparent in the various Arabidopsis isoforms. The overall structures of plant forms are quite conserved compared with the mammalian forms whereas gene phylogenies indicate a different evolutionary course from that of mammalians and a probable common ancestral sequence,Citation65,Citation66 Roth et al. having previously shown that myoglobin or hemoglobins present in higher plants as in protozoa or in insects.Citation67 Indeed, the globin gene family exists in virtually all kingdoms from bacteria to man and a concerted mode of evolution of both animal and plant globin receptor genes is possible.Citation68,Citation69 A pre-existing ancient structural gene has probably conducted to the evolution of not only coding regions, but different regulated functions of hemoglobins (leghemoglobin genes present alignment of sequences very similar to animal receptors).
Photosynthetic proteins of plants have also their homologs in bacteria whereas the equivalent heat shock proteins of eukaryotes and prokaryotes are intensively studied today. At the ontogenetic level, signaling is used like in animals for plant embryogenesis where positional information has a major role for sporofitic cycle and embryonic axis.Citation70 Many homeoboxes are present in plant genomes and have crucial roles in development.Citation71 The different families have diverged prior to the separation of the branches leading to animals, plants and fungi.Citation72 In the same manner, defense signaling pathways are developed at plant level where apoptotic cells and conserved disease resistance genes are present and may be related to functions in animals.Citation73,Citation74 For instance, PR5K receptor kinases are antifungal pathogenesis-related proteins,Citation75 whereas several common signaling and transducing mechanisms using specific proteins or enzymes (like for instance regulating cell division in the plant meristem) common to plant and animal cell communication are described with some specificities.Citation76,Citation77 In the same manner, nuclear receptor gene superfamilies encoding putative receptors and unknown plant or animal ligands have a complex evolutionary history.Citation78 They present, as for the chloroplast-expressed glutamine synthetase (ncpGS), configurations in which gene duplication, swapping between domains important for transcription, DNA or hormone binding are usually found.Citation79
The actin-encoding gene family is also present in plants, being differentially expressed. At least two ancient classes of genes that diverged early in land plant evolution and may have separated vegetative from reproductive actins and six subclasses are identified, showing a distinct pattern of tissue specific expression. They seem to have evolved independently.Citation80 More recent data about cytoskeleton-membrane interactions or actin and endocytosis show that homologs to mammalian proteins of the clathrincoat, those performing basic functions in CCV budding (AP180, epsin), scission (dynamin), uncoating events (auxilin, Hsc70, synaptojanin) and that may act as linkers to the actin cytoskeleton or as key regulators in actin assembly (Arp2), are present in Arabidopsis.Citation81 They are clearly operational, rather as ortholog than homolog proteins compared with mammalian showing high degree of similarity not only in the vesicle fusion but also in the clathrin and network machineries between plants and animals. MAP kinases such as ERK or P38 are also involved in rot cells or targeted to clathrin-coated vesicles.Citation82 Recent works also show that actin, myosin VIII and ABP1 could act as central organizers of auxin-secreting plant synapses.Citation83
Plant Neuroactive Ligands and G-Protein Coupled Receptors
Although these citations are not exhaustive, they reveal the high level of intra- and intercellular communication in plants, previously demonstrated by Wildon et al. (1992) concerning electrical signaling and systemic proteinase inhibitor induction in the wounded plant.Citation84 Two other major binding proteins families are represented in plants: the G-protein family and the calcium binding proteins. For the first one, a cDNA from Arabidopsis thaliana was recently cloned and characterized showing new putative G-protein-coupled receptors with translated sequences showing significant homology to several animal receptors like the calcitonin or serotonin family and the rhodopsin G-protein receptors.Citation85 Heteromeric GTP binding proteins composed of α, β and γ subunits are also involved in signal transduction pathways in both animal and plant systems. In plants, they are implicated in ion channel regulation, light signaling, hormone and pathogen responses are identified. Only one class of plant G α genes has been identified today plus a novel AtXLG1 binding protein.Citation86 A higher plant 7TM receptor (GCR1) is involved in cytokinin signal transduction, suggesting that 7Tm are ancient and predate the divergence of plants and animals.Citation1 The second superfamily now clearly identified in both kingdoms concerns the calcium binding proteins and the calcium-dependent metabolic regulation of biological systems. Indeed Ca2+ ions have multiple functions as a second messenger or as signaling proteins.Citation87 They are well described in eukaryotes and in prokaryotes, indicating a conserved calmodulin gene. Plant calmodulin binds differs from vertebrate ones. So, the whole family of calcium binding proteins may have arisen from a common genetic ancestry.Citation88 Moreover, calmodulin binding to GAD, which is required for the regulation of glutamate and GABA metabolism, is present and necessary to the normal development of plants.Citation89
Recent discoveries thus confirm that except specific mechanosensitive or plant anionic channels, ionotropic glutamate receptors known in rapid synaptic transmission in animal brains exist in plants and are probably involved in light-signal transduction in some species. It may be that signaling between cells by EAA in animal brains evolved from a primitive signaling mechanism that preceded the divergence between animals and plants.Citation90 The structure of these putative glutamate receptor-genes or GLRs is very near. In Arabidopsis, newly isolated GLRs show extensive sequence identity with the same two ligand-bind domains GlnH1-H2 and the 4TM domains M1- M4, especially M3 (the most highly conserved domain). So they have probably co-evolved before the divergence between animals and plants. Their degree of similarity is linked to the kainate-AMPA subtype rather than the NMDA subtype. It indicates that the divergence of animal iGluRs and plant GLR genes predated the divergence of glutamate receptor-genes. It is now possible that they produce GluR agonists in order to regulate their endogenous ionotropic GluRs, and that selective pressure then led to high level production for defense against herbivores. They may also regulate a variety of as yet unknown cell-to cell signaling systems. The fact that iGluRs appear to exist in plants and animals, but not in unicellular organisms, suggests that cell-to cell signaling in multicellular organisms may also predate the divergence of both species subtypes.Citation91,Citation92 Since these discoveries, an accumulation of data has shown the neurotransmitter-like actions or receptor homolog in plants recently summarized by Roshina (2001).Citation93 Among them, specific glutamate agonists, but also GABA mediated transmission implicated in the directional growth of pollen tubes,Citation94 and AcetylcholineCitation95 found at maize plants as acetylcholinesterase or F-actin enriched cross-walls in root apices of Arabidopsis, so-called “plant synapses.”Citation96
Finally, we cannot conclude this presentation of common signaling and transducing pathways in plants and animals without quoting the great phenotypic and epigenetic plasticity of plants recently confirmed for plant communities (variation in the abiotic environment, in the presence or identity of neighbors and in herbivory) by Callaway et al.Citation97 showing competitive to facilitative interactions promoting coexistence and community diversity, or by Schmitz et al. (2011) concerning epimutations of Arabidopsis thaliana studied upon 30 generations and showing probable better advantages than selection during evolution.Citation98
Extracellular Potential Variations as Protoneural Models To Translate Informational Processes Into Function
All these works showing common mechanisms of action of animal and plant organs, together with the evidence of very wide-spread long distance electrical signaling in plants strongly comfort our earlier experimental data on Kalanchoe’s ESVs,Citation2 also shown by some authors in cucurbits.Citation99,Citation100 These data suggested common bioelectrical profiles of animals and plants, including long-distance signal transmission, but also evoked the question of a putative primary integration of information, an IC one, whose role could be to coordinate basic activities and reactivity at the whole plant level. As shown in the text, several loci are now evoked, and particularly the root apex. Related spontaneous or evoked ESV recordings constituted by extracellular potential or “surface potential” variations could indeed represent the summation of transient changes in plasma membrane potential differences carried by ion fluxes flowing across channel proteins, but also be carried by transfer of information linked to the perception of spontaneous magnetic or electric fields (like those used in the fusion and orientation process of membrane cells and vesicles and then by whole plants),Citation101 hydraulic conductivity, cell-to-cell interactions, water and salt exchanges or hormonal transfers. Our results have also shown that low voltage spontaneous activity or evoked responses to mechanical and chemical stimuli could be affected by metabolic agents (). They were therefore assumed to represent the mathematical algebraic derivative of synchronized intracellular activities recorded at tissue level and that could propagate. Their relevance should be more investigated in the future to obtain a complete bioelectrical profile of plants.
We propose to investigate this hypothesis using three research targets: (1) the study of master regulator of genes expression promoting developing plants like PKL in Arabidopsis playing an analogous role in animal C. elegans,Citation102 and more generally genetic factors common to both plants and animals; (2) that of signal transduction concerning oscillations of electrical potentials along leafs or parenchyma/xylem interfaces, electromotive properties of excitable cells or electrical memory like that found in Venus flytrap;Citation103 and (3) the extensive use of Multi-Electrode Array (MEA), Xenopus oocyte functional expression or new imagery technologies to study surface potentials. Indeed, recent technologies like MEA applied to non-nerve electrogenic tissues like plants clearly confirm our earlier discovery showing the existence of spontaneous activity, excitable traveling waves and synchronous bioelectrical network activities at maize root apex. More precisely, using 60-channels MEA, Masi et al. demonstrate for the first time the propagation of synchronous extracellular population spike activities, determining the whole spatiotemporal dynamic profile of these low-voltage propagated signals (10–20 µV peak to peak) and their possible generation at the level of root apex cells.Citation104
Plant Neuroplasticity
Extending these studies to several plant species compared with invertebrate lower animals and mammals seems to be a promising way to explore the spatiotemporal dynamics of ESVs. It could also permit to understand the physiological role of these extracellular spontaneous activities constituting dynamic synchronized networks in plants. More generally, all these bioelectrical activities have to be studied as nonlinear dynamic systems able to detect transitional states and acquisition of crucial environmental information for plant’s survival. This constitutes an undeniable level of integration without nervous system and reflects the dynamic protoneural abilities of plants and lower animals to react with adequacy to stress or environmental changes using sensing effectors to translate information into function. It is possible to extrapolate this kind of reasoning following algorithmic information theories where positive relationships between complexity and the amount of information are established.Citation105 These relationships involve the emergence of new properties, of time-related irreversible changes, of stock and recall of information and probably of precognitive or cognitive behaviors, what is seriously discussed in several recent studies.Citation106-Citation108 The recent confirmations of Wallace’s earlier experiments (1930) about the inhibitory effects of volatile anesthetics on Mimosa pudica or Dionea muscipula’s motoric activitiesCitation109,Citation110 ask questions in this area and could have, as many new fields opened by researches in plant neurobiology, good ‘side effects’, permitting to better understand the mechanism of action of these drugs at the level of the CNS of vertebrates as well as of the cognitive scale of biological systems itself.
More generally, considering biosensors like plants as bottom-up “plastic interfaces” accumulating informational states—the role of extracellular spontaneous potential variations having to be precisely elucidated—could seriously help. Indeed, the epistemic concept of plasticity develops a theoretical model showing plasticity as an inherent property of the matter that is observed at all the levels of organization, describing at the same time a system’s property and its own dynamics.Citation111 This property is considered as not purely systemic or emergent but as a founding one. Plasticity is formally distinguished from passive elasticity or flexibility, having the unique ability to induce active and irreversible binding processes, particularly at the level of living systems. This specificity implies reciprocity between ascending and downward networks like brains and a high cohesion of interacting co-evolutionary systems, the expression of which is finally a co-signified world. Synaptic plasticity illustrates thus typically at the brain-mind interface directly linking biological matter to mental processes. This describes a “complex of plasticity” (from complexiō) that could be described at different scales, like that of the matter-form (morphogenesis), the space-time interface (ST curvature) or the subject-object (human plasticity, intersubjectivity). Metaplasticity explores the genesis of all these natural binding processes obeying to a transverse logic where plasticity plays the part of a catalytic element conducting irreducible expressions or dimensions to a trans-formation.
To summarize, we can consider three main levels of plasticity, the first being the plasticity of the matter, the second the plastic code of life and the third the plasticity of mind (not concerned in our context). This indicates an irreversible binding, contrarily to elastic processes, well- illustrated by synaptic plasticity at the brain level, and a dynamic behavior, particularly relevant at complex co-evolving or co-implicated living systems. That fits well with the consideration of plant integrated bio-systems having high capacities to percept and interact “intelligibly” with their congeners or specific animal species. It also constitutes a new transdisciplinary challenge in living organism’s plasticity where plants may act as pre-cognitive or cognitive entities.Citation112 Since the symposium on plant neurobiology held in Florence in 2005, such a transdisciplinary field is clearly open, progressively changing our point of view concerning biological complexity, extended levels of cognition and evolutionary processes. We can therefore hope that plants will be recognized in a close future as self-organized biosensors having high capacities to perceive, to communicate and to interact with their environment and the ecosystem.
Abbreviations: | ||
AP | = | action potential |
ESV | = | extracellular spontaneous bioelectrical variations |
EPG | = | electrophytograhic activity |
IC | = | integrative center, MEA, multielectrode array |
FC | = | fusicoccin |
FCCP | = | carbonyl cyanide p-(trifluoromethoxy) phenylhydrazone |
GABA | = | gamma-aminobutyric acid |
GLRs | = | glutamate receptor genes |
NMDA | = | N-methyl-D-aspartate |
PKL | = | pickle |
ATP | = | adenosine triphosphate |
GTP | = | guanosine triphosphate |
EAA | = | excitatory amino acid |
GAD | = | glutamate decarboxylase |
GCR | = | glucocorticoïd receptor |
Acknowledgments
We thank Professor F. Baluška for his encouragements to write this paper and Ilke Angela Marechal for the proofreading of the manuscript in English.
Disclosure of Potential Conflicts of Interest
No potential conflicts of interest were disclosed.
References
- Bose JC. Comparative electrophysiology. London, New-York, Toronto: Longmans, Green & Co., 1901.
- Tompkins P, Bird C. The secret life of plants. Harper & Row, 1973.
- Brenner ED, Stahlberg R, Mancuso S, Vivanco J, Baluška F, Van Volkenburgh E. Plant neurobiology: an integrated view of plant signaling. Trends Plant Sci 2006; 11:413 - 9; http://dx.doi.org/10.1016/j.tplants.2006.06.009; PMID: 16843034
- Baluška F, Mancuso S, Volkmann D. Communication in plants: neuronal aspects of plant life: Springer Verlag, 2006.
- Hedrich R, Schroeder JI, Fernandez JM. Patch-clamp studies on higher plant cells: a perspective. Trends Biol Sci 1987; 12:49 - 52; http://dx.doi.org/10.1016/0968-0004(87)90025-9
- Ivashikina N, Becker D, Ache P, Meyerhoff O, Felle HH, Hedrich RK. K(+) channel profile and electrical properties of Arabidopsis root hairs. FEBS Lett 2001; 508:463 - 9; http://dx.doi.org/10.1016/S0014-5793(01)03114-3; PMID: 11728473
- Zhang W, Nilson SE, Assmann SM. Isolation and whole-cell patch clamping of Arabidopsis guard cell protoplasts. CSH Protoc 2008; 2008:t5014; PMID: 21356848
- Ward JM, Mäser P, Schroeder JI. Plant ion channels: gene families, physiology, and functional genomics analyses. Annu Rev Physiol 2009; 71:59 - 82; http://dx.doi.org/10.1146/annurev.physiol.010908.163204; PMID: 18842100
- Debono MW, Bouteau F. Spontaneous and evoked surface potentials in Kalanchoe tissues. Life Sci Adv Plant Physiol 1992; 11:107 - 17
- Paszeuski A, Krolikowska Z.. Investigation of electric potentials in plants. Ann Univ Marie Curie, Lublin 1961; L:XVI-9 141 - 52
- Sinyukin AM, Stolarek J. Investigation of electric potentials in plants. Ann Univ Mariae Curie Sklodowska Sect 3 [Biol] 1961; 16:215 - 28
- Karlsson L. Nonrandom bioelectrical signals in plant tissue. Plant Physiol 1972; 49:982 - 6; http://dx.doi.org/10.1104/pp.49.6.982; PMID: 16658096
- Pickard BG. Spontaneous electrical activity in shoots of Ipomea, Pisum and Xanthium. Planta 1971; 102:91 - 113; http://dx.doi.org/10.1007/BF00384863
- Pickard BG. Action potentials in higher plants. Bot Rev 1973; 39:172 - 201; http://dx.doi.org/10.1007/BF02859299
- La Spada G, Salleo A, Salleo S, Urna G. Attività elttrica spontanea in Sechium edule. Riv Biol 1977; LXX:1 - 2
- Pfirsh R, Stoeckel H. Analysis of the absolute refractory stage of the action potential in Mimosa pudica. CRAS, Paris 1969; 268:1725
- Sibaoka T. Excitable cells in Mimosa. Science 1962; 137:226; http://dx.doi.org/10.1126/science.137.3525.226; PMID: 13912476
- Sibaoka T. Physiology of rapid movements in higher plants. Annu Rev Plant Physiol 1969; 20:165 - 84; http://dx.doi.org/10.1146/annurev.pp.20.060169.001121
- Stoëckel H. Electrophysiological study of excitability and transmission phenomena at the level of primary pulvinus of Mimosa pudica. PhD thesis, Strasbourg, France 1976.
- Hedrich R, Moran O, Conti F, Busch H, Becker D, Gambale F, et al. patch-clamp studies on higher plant cells: a perspective. Trends Biol Sci 1987; 12:49 - 52; http://dx.doi.org/10.1016/0968-0004(87)90025-9
- Debono MW, Bouteau F. Spontaneous and evoked surface potentials in Kalanchoe tissues. Life Sci Adv Plant Physiol 1992; 11:107 - 17
- Haswell ES, Phillips R, Rees DC. Mechanosensitive channels: what can they do and how do they do it?. Structure 2011; 19:1356 - 69; http://dx.doi.org/10.1016/j.str.2011.09.005; PMID: 22000509
- 1st Symposium on plant neurobiology, Accademia dei Georgofili, Florence, May 17-20, 2005.
- Trewavas A. Green plants as intelligent organisms. Trends Plant Sci 2005; 10:413 - 9; http://dx.doi.org/10.1016/j.tplants.2005.07.005; PMID: 16054860
- Debono MW, Bouteau F. Spontaneous and evoked surface potentials in Kalanchoe tissues. Life Sci Adv Plant Physiol 1992; 11:107 - 17
- Lew RR. Pressure regulation of the electrical properties of growing Arabidopsis thaliana L. root hairs. Plant Physiol 1996; 112:1089 - 100; http://dx.doi.org/10.1104/pp.112.3.1089; PMID: 8938411
- Davies E. Intercellular and intracellular signals and their transduction via the plasma membrane-cytoskeleton interface. Semin Cell Biol 1993; 4:139 - 47; http://dx.doi.org/10.1006/scel.1993.1017; PMID: 8391345
- Schroeder JI, Hagiwara S. Repetitive increases in cytosolic Ca2+ of guard cells by abscisic acid activation of nonselective Ca2+ permeable channels. Proc Natl Acad Sci USA 1990; 87:9305 - 9; http://dx.doi.org/10.1073/pnas.87.23.9305; PMID: 2174559
- Benthrup FW. Reception and transduction of electrical and mechanical stimuli. Encycl of Plant Physiol 1979; New Series:7 42 - 70
- Desbiez MO. Experimental basis of a new interpretation of correlations between the cotyledon and its axillary shoot. PhD thesis, Clermont-Ferrand, France 1985.
- Miller AJ, Zhou JJ. Xenopus oocytes as an expression system for plant transporters. Biochim Biophys Acta 2000; 1465:343 - 58; http://dx.doi.org/10.1016/S0005-2736(00)00148-6; PMID: 10748264
- MacKinnon R, Latorre R, Miller C. Role of surface electrostatics in the operation of a high-conductance Ca2+-activated K+ channel. Biochemistry 1989; 28:8092 - 9; http://dx.doi.org/10.1021/bi00446a020; PMID: 2605175
- Weisenseel MH, Nuccitelli R, Jaffe LF. Large electrical currents traverse growing pollen tubes. J Cell Biol 1975; 66:556 - 67; http://dx.doi.org/10.1083/jcb.66.3.556; PMID: 1158971
- Cooke TJ, Racusen RH. The role of electrical phenomena in tip growth, with special reference to the developmental plasticity of filamentous fern gametophytes. Symp Soc Exp Biol 1986; 40:307 - 28; PMID: 3544307
- Véry AA, Davies JM. Hyperpolarization-activated calcium channels at the tip of Arabidopsis root hairs. Proc Natl Acad Sci USA 2000; 97:9801 - 6; http://dx.doi.org/10.1073/pnas.160250397; PMID: 10920194
- Davies E. Intercellular and intracellular signals and their transduction via the plasma membrane-cytoskeleton interface. Semin Cell Biol 1993; 4:139 - 47; http://dx.doi.org/10.1006/scel.1993.1017; PMID: 8391345
- Nuccitelli R. Ionic currents in morphogenesis. Experientia 1988; 44:657 - 66; http://dx.doi.org/10.1007/BF01941026; PMID: 2457508
- Goldsworthy A. The electric compass of plants. New Sci 1986; 2:22
- van IJzendoorn SC, Hoekstra D. The subapical compartment: a novel sorting centre?. Trends Cell Biol 1999; 9:144 - 9; http://dx.doi.org/10.1016/S0962-8924(99)01512-3; PMID: 10203791
- Wang L. Measurements and implications of the membrane dipole potential. Annu Rev Biochem 2012; 81:615 - 35; http://dx.doi.org/10.1146/annurev-biochem-070110-123033; PMID: 22443933
- Kinraide TB. Possible influence of cell walls upon ion concentrations at plasma membrane surfaces. Toward a comprehensive view of cell-surface electrical effects upon ion uptake, intoxication, and amelioration. Plant Physiol 2004; 136:3804 - 13; http://dx.doi.org/10.1104/pp.104.043174; PMID: 15489281
- Bastien R, Bohr T, Moulia B, Douady S. Unifying model of shoot gravitropism reveals proprioception as a central feature of posture control in plants. Proc Natl Acad Sci USA 2013; 110:755 - 60; http://dx.doi.org/10.1073/pnas.1214301109; PMID: 23236182
- Baluška F, Mancuso S, Volkmann D, Barlow PW. The ‘root-brain’ hypothesis of Charles and Francis Darwin: Revival after more than 125 years. Plant Signal Behav 2009; 4:1121 - 7; http://dx.doi.org/10.4161/psb.4.12.10574; PMID: 20514226
- XVIth International Botanical congress of St Louis MO, USA 1999.
- Chiu J, DeSalle R, Lam HM, Meisel L, Coruzzi G. Molecular evolution of glutamate receptors: a primitive signaling mechanism that existed before plants and animals diverged. Mol Biol Evol 1999; 16:826 - 38; http://dx.doi.org/10.1093/oxfordjournals.molbev.a026167; PMID: 10368960
- Baluška F, Mancuso S, Volkmann D, Barlow P. Root apices as plant command centres: the unique ‘brain like’ status of the root apex transition zone. Biologia (Bratisl) 2004; 59:7 - 19
- Baluška F, Volkmann D, Menzel D. Plant synapses: actin-based domains for cell-to-cell communication. Trends Plant Sci 2005; 10:106 - 11; http://dx.doi.org/10.1016/j.tplants.2005.01.002; PMID: 15749467
- Debono MW. From perception to consciousness: an epistemic vision of evolutionary processes. Leonardo. Theoretical Perspective 2004; 37:243 - 8
- Hedrich R, Jeromin A. A new scheme of symbiosis: ligand- and voltage-gated anion channels in plants and animals. Philos Trans R Soc Lond B Biol Sci 1992; 338:31 - 8; http://dx.doi.org/10.1098/rstb.1992.0126; PMID: 1280838
- Vian A, Henry-Vian C, Schantz R, Ledoigt G, Frachisse JM, Desbiez MO, et al. Is membrane potential involved in calmodulin gene expression after external stimulation in plants?. FEBS Lett 1996; 380:93 - 6; http://dx.doi.org/10.1016/0014-5793(96)00015-4; PMID: 8603755
- Rinne PL, van der Schoot C. Symplasmic fields in the tunica of the shoot apical meristem coordinate morphogenetic events. Development 1998; 125:1477 - 85; PMID: 9502728
- Davies E. Intercellular and intracellular signals and their transduction via the plasma membrane-cytoskeleton interface. Semin Cell Biol 1993; 4:139 - 47; http://dx.doi.org/10.1006/scel.1993.1017; PMID: 8391345
- Miedema H, Prins HB. Simulation of the light-induced oscillations of the membrane potential in Potamogeton leaf cells. J Membr Biol 1993; 133:107 - 17; http://dx.doi.org/10.1007/BF00233792; PMID: 8515430
- Schönknecht G, Althoff G, Junge W. The electric unit size of thylakoid membranes. FEBS Lett 1990; 277:65 - 8; http://dx.doi.org/10.1016/0014-5793(90)80810-6; PMID: 1702737
- Matzke AJ, Behensky C, Weiger T, Matzke MA. A large conductance ion channel in the nuclear envelope of a higher plant cell. FEBS Lett 1992; 302:81 - 5; http://dx.doi.org/10.1016/0014-5793(92)80290-W; PMID: 1375170
- Cooke TJ, Racusen RH. The role of electrical phenomena in tip growth, with special reference to the developmental plasticity of filamentous fern gametophytes. Symp Soc Exp Biol 1986; 40:307 - 28; PMID: 3544307
- Miller MW, Dooley DA, Cox C, Carstensen EL. On the mechanism of 60-Hz electric field induced effects in Pisum sativum L. roots: vertical field exposures. Radiat Environ Biophys 1983; 22:293 - 302; http://dx.doi.org/10.1007/BF01323679; PMID: 6665120
- Davies E. Intercellular and intracellular signals and their transduction via the plasma membrane-cytoskeleton interface. Semin Cell Biol 1993; 4:139 - 47; http://dx.doi.org/10.1006/scel.1993.1017; PMID: 8391345
- Gradmann D, Blatt MR, Thiel G. Electrocoupling of ion transporters in plants. J Membr Biol 1993; 136:327 - 32; http://dx.doi.org/10.1007/BF00233671; PMID: 8114082
- Gradmann D. Models for oscillations in plants. Aust J Plant Physiol 2001; 28:577 - 90
- Bauer CS, Plieth C, Hansen UP, Sattelmacher B, Simonis W, Schönknecht G. Repetitive Ca2+ spikes in a unicellular green alga. FEBS Lett 1997; 405:390 - 3; http://dx.doi.org/10.1016/S0014-5793(97)00231-7; PMID: 9108324
- Fromm J, Lautner S. Electrical signals and their physiological significance in plants. Plant Cell Environ 2007; 30:249 - 57; http://dx.doi.org/10.1111/j.1365-3040.2006.01614.x; PMID: 17263772
- Osterlund MT, Ang LH, Deng XW. The role of COP1 in repression of Arabidopsis photomorphogenic development. Trends Cell Biol 1999; 9:113 - 8; http://dx.doi.org/10.1016/S0962-8924(99)01499-3; PMID: 10201077
- Hicke L. Gettin’ down with ubiquitin: turning off cell-surface receptors, transporters and channels. Trends Cell Biol 1999; 9:107 - 12; http://dx.doi.org/10.1016/S0962-8924(98)01491-3; PMID: 10201076
- Ferl RJ, Lu G, Bowen BW. Evolutionary implications of the family of 14-3-3 brain protein homologs in Arabidopsis thaliana. Genetica 1994; 92:129 - 38; http://dx.doi.org/10.1007/BF00163762; PMID: 7958937
- Wu K, Rooney MF, Ferl RJ. The Arabidopsis 14-3-3 multigene family. Plant Physiol 1997; 114:1421 - 31; http://dx.doi.org/10.1104/pp.114.4.1421; PMID: 9276953
- Roth J, Leroith D, Collier ES, Watkinson A, Lesniak MA. The evolutionary origins of intercellular communication and the Maginot Lines of the mind. Ann N Y Acad Sci 1986; 463:1 - 11; http://dx.doi.org/10.1111/j.1749-6632.1986.tb21498.x; PMID: 3013064
- Hardison R. Hemoglobins from bacteria to man: evolution of different patterns of gene expression. J Exp Biol 1998; 201:1099 - 117; PMID: 9510523
- Brown GG, Lee JS, Brisson N, Verma DP. The evolution of a plant globin gene family. J Mol Evol 1984; 21:19 - 32; http://dx.doi.org/10.1007/BF02100624; PMID: 6442356
- Harada JJ. Signaling in plant embryogenesis. Curr Opin Plant Biol 1999; 2:23 - 7; http://dx.doi.org/10.1016/S1369-5266(99)80005-3; PMID: 10047570
- Chan RL, Gago GM, Palena CM, Gonzalez DH. Homeoboxes in plant development. Biochim Biophys Acta 1998; 1442:1 - 19; http://dx.doi.org/10.1016/S0167-4781(98)00119-5; PMID: 9767075
- Wang DY, Kumar S, Hedges SB. Divergence time estimates for the early history of animal phyla and the origin of plants, animals and fungi. [Biol] Proc Biol Sci 1999; 266:163 - 71; http://dx.doi.org/10.1098/rspb.1999.0617; PMID: 10097391
- Pifanelli P, Devoto A, Schulze-Lefert P.. Defense signaling pathways in plants. Current opinion in Biology 1999; 2:295 - 300
- Baker B, Zambryski P, Staskawicz B, Dinesh-Kumar SP. Signaling in plant-microbe interactions. Science 1997; 276:726 - 33; http://dx.doi.org/10.1126/science.276.5313.726; PMID: 9115193
- Wang X, Zafian P, Choudhary M, Lawton M. The PR5K receptor protein kinase from Arabidopsis thaliana is structurally related to a family of plant defense proteins. Proc Natl Acad Sci USA 1996; 93:2598 - 602; http://dx.doi.org/10.1073/pnas.93.6.2598; PMID: 8637920
- Kohorn BD. Shuffling the deck: plant signalling plays a club. Trends Cell Biol 1999; 9:381 - 3; http://dx.doi.org/10.1016/S0962-8924(99)01643-8; PMID: 10481175
- Walker JC. Structure and function of the receptor-like protein kinases of higher plants. Plant Mol Biol 1994; 26:1599 - 609; http://dx.doi.org/10.1007/BF00016492; PMID: 7858206
- Laudet V, Hänni C, Coll J, Catzeflis F, Stéhelin D. Evolution of the nuclear receptor gene superfamily. EMBO J 1992; 11:1003 - 13; PMID: 1312460
- Emshwiller E, Doyle JJ. Chloroplast-expressed glutamine synthetase (ncpGS): potential utility for phylogenetic studies with an example from Oxalis (Oxalidaceae). Mol Phylogenet Evol 1999; 12:310 - 9; http://dx.doi.org/10.1006/mpev.1999.0613; PMID: 10413625
- McDowell JM, Huang S, McKinney EC, An YQ, Meagher RB. Structure and evolution of the actin gene family in Arabidopsis thaliana. Genetics 1996; 142:587 - 602; PMID: 8852856
- Sanderfoot AA, Raikhel NV. The secretory system of Arabidopsis. In Somerville CR, Meyerovitz EM Ed. The arbidopsis book. Am Soc Plant Biol 2002.
- Samaj J, Read ND, Volkmann D, Menzel D, Baluskă F. The endocytic network in plants. Trends Cell Biol 2005; 15:425 - 33; http://dx.doi.org/10.1016/j.tcb.2005.06.006; PMID: 16006126
- Baluška F. in Volkov AG. Ed. Plant electrophysiology: method and cell electrophysiology. Springer, 2012.
- Wildon DC, Thain JH, Minchin PEH, Gubb IR, Reilly AJ, Skipper YD, et al. Electrical signaling and systemic proteinase inhibitor induction in the wounded plant. Nature 1992; 360:62 - 65
- Josefsson LG, Rask L. Cloning of a putative G-protein-coupled receptor from Arabidopsis thaliana. Eur J Biochem 1997; 249:415 - 20; http://dx.doi.org/10.1111/j.1432-1033.1997.t01-1-00415.x; PMID: 9370348
- Lee YR, Assmann SM. Arabidopsis thaliana ‘extra-large GTP-binding protein’ (AtXLG1): a new class of G-protein. Plant Mol Biol 1999; 40:55 - 64; http://dx.doi.org/10.1023/A:1026483823176; PMID: 10394945
- Plakidou-Dymock S, Dymock D, Hooley R. A higher plant seven-transmembrane receptor that influences sensitivity to cytokinins. Curr Biol 1998; 8:315 - 24; http://dx.doi.org/10.1016/S0960-9822(98)70131-9; PMID: 9512416
- Malho R. Coding information in plant cells: the multiple roles of Ca2+ as a second messenger. Plant Biol 1999; 1:487 - 494; http://dx.doi.org/10.1111/j.1438-8677.1999.tb00774.x
- Baum G, Lev-Yadun S, Fridmann Y, Arazi T, Katsnelson H, Zik M, et al. Calmodulin binding to glutamate decarboxylase is required for regulation of glutamate and GABA metabolism and normal development in plants. EMBO J 1996; 15:2988 - 96; PMID: 8670800
- Sengupta LK, Singh BB, Mishra R, Pandey PK, Singh S, Sengupta S, et al. Calcium-dependent metabolic regulations in prokaryotes indicate conserved nature of calmodulin gene. Indian J Exp Biol 1998; 36:136 - 47; PMID: 9754041
- Chiu J, DeSalle R, Lam HM, Meisel L, Coruzzi G. Molecular evolution of glutamate receptors: a primitive signaling mechanism that existed before plants and animals diverged. Mol Biol Evol 1999; 16:826 - 38; http://dx.doi.org/10.1093/oxfordjournals.molbev.a026167; PMID: 10368960
- Lam HM, Chiu J, Hsieh MH, Meisel L, Oliveira IC, Shin M, et al. Glutamate-receptor genes in plants. Nature 1998; 396:125 - 6; http://dx.doi.org/10.1038/24066; PMID: 9823891
- Roshina VV. Neurotransmitters in plant life. Science Publishers, 2001.
- Bouché N, Fromm H. GABA in plants: just a metabolite?. Trends Plant Sci 2004; 9:110 - 5; http://dx.doi.org/10.1016/j.tplants.2004.01.006; PMID: 15003233
- Sagane Y, Nakagawa T, Yamamoto K, Michikawa S, Oguri S, Momonoki YS. Molecular characterization of maize acetylcholinesterase: a novel enzyme family in the plant kingdom. Plant Physiol 2005; 138:1359 - 71; http://dx.doi.org/10.1104/pp.105.062927; PMID: 15980188
- Henze S. Schlicht M, Menzel D, Baluska F. Acetycholine signaling targets ‘plant synapses’. Abst. of the 1st Symposium on plant neurobiology, Accademia dei Georgofili, Florence, 2005.
- Callaway RM, Ragan M, Pennings SC, Richards CL. Phenotypic plasticity and interactions among plants. Ecology 2003; 84:1115 - 28; http://dx.doi.org/10.1890/0012-9658(2003)084[1115:PPAIAP]2.0.CO;2
- Schmitz RJ, Schultz MD, Lewsey MG, O’Malley RC, Urich MA, Libiger O, et al. Transgenerational epigenetic instability is a source of novel methylation variants. Science 2011; 334:369 - 73; http://dx.doi.org/10.1126/science.1212959; PMID: 21921155
- Debono MW, Bouteau F. Spontaneous and evoked surface potentials in Kalanchoe tissues. Life Sci Adv Plant Physiol 1992; 11:107 - 17
- Gunar II, Sinyukin AM. Functional significiance of action currents affecting the gas exchange of higher plants. Sov Plant Physiol 1963; 10:219 - 26
- Zimmermann U. Electric field-mediated fusion and related electrical phenomena. Biochim Biophys Acta 1982; 694:227 - 77; http://dx.doi.org/10.1016/0304-4157(82)90007-7; PMID: 6758848
- Dean Rider S Jr., Henderson JT, Jerome RE, Edenberg HJ, Romero-Severson J, Ogas J. Coordinate repression of regulators of embryonic identity by PICKLE during germination in Arabidopsis.. Plant J 2003; 35:33 - 43; http://dx.doi.org/10.1046/j.1365-313X.2003.01783.x; PMID: 12834400
- Volkov AG, Carell H, Baldwin A, Markin VS. Electrical memory in Venus flytrap. Plant Cell Environ 2010; 33:163 - 73; http://dx.doi.org/10.1111/j.1365-3040.2009.02066.x; PMID: 19895396
- Masi E, Ciszak M, Stefano G, Renna L, Azzarello E, Pandolfi C, et al. Spatiotemporal dynamics of the electrical network activity in the root apex. Proc Natl Acad Sci USA 2009; 106:4048 - 53; http://dx.doi.org/10.1073/pnas.0804640106; PMID: 19234119
- Maze J. Studies into abstract properties of individuals. III: A study of factors affecting emergence. Int J Plant Sci 1999; 160:809 - 17; http://dx.doi.org/10.1086/314181; PMID: 10506461
- Garzón FC, Keijzer F. Cognitions in Plants. In F. Baluskă ed. Plant environment interactions: behavioral perspective. Elsevier, 2009; 1-16.
- Garzón FC. The quest for cognition in plant neurobiology. Plant Signal Behav 2007; 2:208 - 11; http://dx.doi.org/10.4161/psb.2.4.4470; PMID: 19516990
- Barlow P. Plant roots: autopoietic and cognitive constructions. Plant Root 2010; 4:40 - 52; http://dx.doi.org/10.3117/plantroot.4.40
- Okasaki N, Takai K, Sato T. Immobilization of a sensitive plant, Mimosa pudica L. by volatile anesthetics. The Jap J of Anesthesiol 1993; 42:1190 - 3
- De Luccia TP. Mimosa pudica, Dionaea muscipula and anesthetics. Plant Signal Behav 2012; 7:1163 - 7; http://dx.doi.org/10.4161/psb.21000; PMID: 22899087
- Debono MW. The concept of plasticity: a new epistemological paradigm. Dogma 2005.
- Debono MW. Perceptive levels in plants: a transdisciplinary challenge in living organism's plasticity. Trans J Eng & Sci 2013; In press