Abstract
The endophytic fungus Piriformospora indica colonizes the roots of many plant species including Arabidopsis and promotes their performance, biomass, and seed production as well as resistance against biotic and abiotic stress. Imbalances in the symbiotic interaction such as uncontrolled fungal growth result in the loss of benefits for the plants and activation of defense responses against the microbe. We exposed Arabidopsis seedlings to a dense hyphal lawn of P. indica. The seedlings continue to grow, accumulate normal amounts of chlorophyll, and the photosynthetic parameters demonstrate that they perform well. In spite of high fungal doses around the roots, the fungal material inside the roots was not significantly higher when compared with roots that live in a beneficial symbiosis with P. indica. Fifteen defense- and stress-related genes including PR2, PR3, PAL2, and ERF1 are only moderately upregulated in the roots on the fungal lawn, and the seedlings did not accumulate H2O2/radical oxygen species. However, accumulation of anthocyanin in P. indica-exposed seedlings indicates stress symptoms. Furthermore, the jasmonic acid (JA) and jasmonic acid-isoleucine (JA-Ile) levels were increased in the roots, and consequently PDF1.2 and a newly characterized gene for a 2-oxoglurate and Fe2+-dependent oxygenase were upregulated more than 7-fold on the dense fungal lawn, in a JAR1- and EIN3-dependent manner. We conclude that growth of A. thaliana seedlings on high fungal doses of P. indica has little effect on the overall performance of the plants although elevated JA and JA-Ile levels in the roots induce a mild stress or defense response.
Introduction
Mutualism is a balanced stage of plant/microbe interaction where both partners benefit from each other.Citation1-Citation3 In the symbiosis between the clavicipitaceous fungal endophyte Epichloë festucae and its host ryegrass, the degree of root colonization determines whether the interaction is mutualistic or parasitic.Citation4 In mycorrhiza, the beneficial interaction is based on the delivery of soil nutrients from the fungus to the plant and reduced carbon from the plant to the fungus.Citation5 However, environmental changes or mutations can shift these mutualistic interactions to commensalism (when one organism benefits without affecting the other) or parasitism (when one organism benefits while the other is harmed). Crucial for the maintenance of a mutualistic interaction is a balanced growth of the 2 symbionts, which requires a permanent signaling to establish an equilibrium between plant defense gene activation and propagation of the fungus.Citation1,Citation2,Citation6-Citation13 This balance is also a prerequisite for appropriate reprogramming of the host development in response to endosymbionts.Citation14 The important role of defense gene activation in symbiotic interactions is shown for rice, where 43% of the genes respond to colonization by both arbuscular mycorrhiza fungi and pathogenic fungi, and many of them are involved in plant defense and stress.Citation15 Campos-Soriano and SegundoCitation8 proposed that increased demands for sugars by the fungus might activate host defense responses that will then contribute to the stabilization of root colonization. Plants may restrict carbohydrate flux toward their mycorrhizal partners to avoid fungal parasitism.Citation7 In tomato mycorrhiza, oxylipin metabolism and signaling may activate host defense responses that will contribute to both the control of fungal spread and the increased resistance to fungal pathogens in mycorrhizal plants.Citation9 Finally, Barto et al.Citation16 proposed fungal superhighways that enhance belowground communication. In their model, infochemical transport via common mycorrhizal networks allows chemical defense signaling across plant populations. These examples demonstrate that regulation of plant defense and stress genes is crucial in symbiotic interactions and for the restriction of root colonization. A balanced activation of defense genes from the host is important to control fungal growth.
We study the interaction of Arabidopsis roots with the endophytic fungus Piriformospora indica, which colonizes the roots of many plant species. Similar to arbuscular mycorrhizal fungi, P. indica promotes plant growth, biomass, and seed productionCitation17 and confers resistance to biotic and abiotic stress.Citation18,Citation19 P. indica is a member of Sebacinales, grows inter- and intracellularly and forms pear-shaped spores, which accumulate within the roots and on the root surface.Citation20 After the establishment of a beneficial interaction, barely any defense or stress genes are activated and no reactive oxygen species (ROS) are produced by the host against P. indica.Citation3,Citation21,Citation22 Like in mycorrhizal symbiosis, the reason for the low level of plant defense against beneficial microbes in unknown. Jacobs et al.Citation23 proposed that P. indica is confronted with a functional plant immune system. It does not evade plant detection but rather suppresses immunity by various microbe-associated molecular patterns. Furthermore, they could show that the ability to suppress host immunity is compromised in the jasmonate mutants jasmonate-insensitive 1 (jin1) and jasmonate-resistant 1 (jar1). We and others have shown that the mutualistic interaction is disturbed in Arabidopsis mutants with lesions in specific defense genes or signaling processes leading to defense gene activation.Citation19,Citation21,Citation23-Citation26 Mutants with lesions in a specific defense response are often unable to restrict growth of P. indica hyphae in the roots, and consequently, the roots become overcolonized. The host plant responds to it by activating other defense processes, which are not mutated in the host, to restrict fungal growth and to re-establish a balanced symbiosis of the 2 partners. In contrast to mycorrhizal fungi, P. indica can grow on synthetic media without a host.Citation27 Therefore we addressed the question of how Arabidopsis seedlings develop when they are growing on a dense fungal lawn. Do high fungal doses in the environment of the roots also lead to a higher colonization of the roots and, if so, does this affect plants’ performance? Do the plants activate defense responses against the high fungal doses that surround the roots?
Results
High doses of P. indica inhibit growth but do not have any effect on the efficiency of the photosynthetic electron transport in Arabidopsis seedlings. When 9-d-old Arabidopsis seedlings were transferred from MS to Kaefer medium (KM) with or without a dense fungal lawn for 7 d (), growth of the seedlings was slower on the medium with the fungus compared with the control. We observed a > 2-fold increase in the shoot and root fresh weights, respectively, for seedlings grown in the presence of the fungus for 7 d, while the increase on media without the fungus was ~3-fold (). The 2-fold increase in root and shoot fresh weight clearly indicates that the seedlings can grow on the fungal lawn. The slower growth rate in the presence of the fungus might be caused by an inhibitory effect of the fungus or simply by the fact that the access of the roots to nutrients in the agar medium is reduced ( and ).
Figure 1. Plants exposed to a dense fungal lawn. (A) Nine-day-old Arabidiopsis wild-type seedlings were transferred from MS medium to a plate with KM without P. indica (left) or with a dense fungal lawn (right) for 7 d. Growth occurred in continuous light at 80 µmol min-2 sec-1. (B) False color images of typical seedlings representing Fs/Fm values as described in Methods and Material and ref. Citation29.
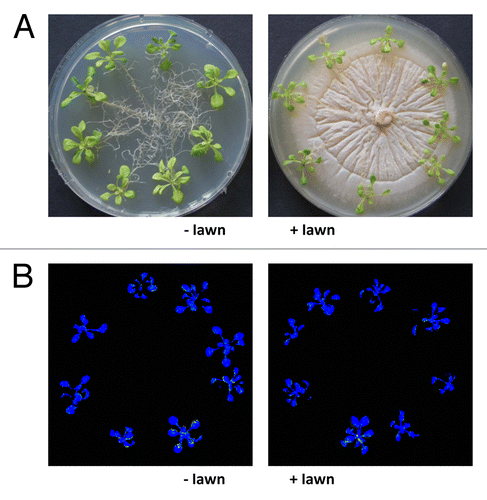
Figure 2. Fold increase in the fresh weight of the shoots and roots during the 7-d growth of the Arabidopsis wild-type (WT), ein3, and jar1 seedlings on KM in the presence (+ lawn) or absence (− lawn) of P. indica. The values represent [fresh weight 9d MS + 7d KM ±P. indica/fresh weight9d MS]. Based on 6 independent experiments with 20 seedlings each, bars represent SEs. Errors are the sum of the individual errors.
![Figure 2. Fold increase in the fresh weight of the shoots and roots during the 7-d growth of the Arabidopsis wild-type (WT), ein3, and jar1 seedlings on KM in the presence (+ lawn) or absence (− lawn) of P. indica. The values represent [fresh weight 9d MS + 7d KM ±P. indica/fresh weight9d MS]. Based on 6 independent experiments with 20 seedlings each, bars represent SEs. Errors are the sum of the individual errors.](/cms/asset/da1a1d12-dd56-482e-ac71-56130bb2c1f7/kpsb_a_10926301_f0002.gif)
The efficiency of the photosynthetic electron flow, measured by chlorophyll fluorescence based parameters, is a sensitive parameter for the fitness of a plant.Citation28-Citation30 After 7 d on KM either with or without the fungal lawn, the seedlings were dark-adapted for 15 min and the chlorophyll fluorescence was measured using a FluorCam 700F. False color images of the seedlings in plates () are shown in (compare ref. Citation29) and quantified data are presented in . The quantum yield of photosystem II (ФPSII), photochemical (qP) and non-photochemical quenching (NPQ), and maximum quantum yield of PSII (Fv/Fm) were almost identical for seedlings exposed to the dense fungal lawn and the control (; ). Thus, the efficiency of the photosynthetic electron transport (ФPSII, qP), the ability of heat dissipation of photochemical energy (NPQ), and the ratio of function photosystem II to total photosystem II (Fv/Fm) were not impaired by exposure of the seedlings to the dense fungal lawn. Furthermore, the amount of chlorophyll per gram fresh weight was not significantly different between the 2 treatments (). We have previously demonstrated that the efficiency of the photosynthetic electron transport is not affected or even slightly improved when the seedlings are exposed to a low concentration of the fungus and beneficial interaction conditions.Citation30 We conclude that the overall performance of the seedlings on the dense fungal lawn is quite well and comparable to seedlings that were not exposed to the dense fungal lawn, although their growth rate is reduced.
Table 1. Chlorophyll concentration and photosynthetic electron transfer efficiency in the leaves of Arabidopsis seedlings grown on a dense fungal lawn of P. indica.
Intracellular growth of the mycelium on the dense fungal lawn is not significantly higher than under beneficial co-cultivation conditions
Growth of the seedlings on the dense fungal lawn results in a high concentration of mycelium and spores around the roots (). After staining of the fungal material (compare Material and Methods) and microscopic analyses of the distribution of the mycelium and spores on the root surface and in the root material, we observed that the concentration of mycelium and spores inside the roots of seedlings grown on the fungal lawn was not higher than in roots that were grown with the fungus under beneficial co-cultivation conditions (). This demonstrates that growth of the fungus inside the root material is independent of the concentration of the fungal material around the roots. This is further supported by quantitative RT-PCR analyses (): the fungal ITS cDNA/plant GAPC2 cDNA ratio is not significantly different in roots grown under beneficial co-cultivation conditions or on the fungal lawn.
Figure 3. (A) Distribution of fungal mycelium and spores on the root surface and in the root material. The seedlings were either co-cultivated with P. indica on the fungal lawn for 7 d (upper part, fungal lawn), or co-cultivated with the fungus under beneficial conditions for 7 d (lower part, beneficial interaction). After staining of the fungal material, light microscopic or fluorescent pictures were taken from the root surface or from the central part of the root. Note the high concentration of spores and mycelium on the surface of the roots grown on the fungal lawn, which are not detectable inside of the roots. Under beneficial co-cultivation conditions for 7 d, spores are not yet formed, but the mycelium is detectable on the root surface. (B) The amount of the fungal ITS cDNA relative to the root GAPC2 mRNA. Arabidopsis seedlings were co-cultivated with P. indica under beneficial conditions or on the fungal lawn for 7 d. After extensive washing of the roots, the mRNA was extracted and the amounts of the fungal and plant genes were determined by quantitative RT-PCR.
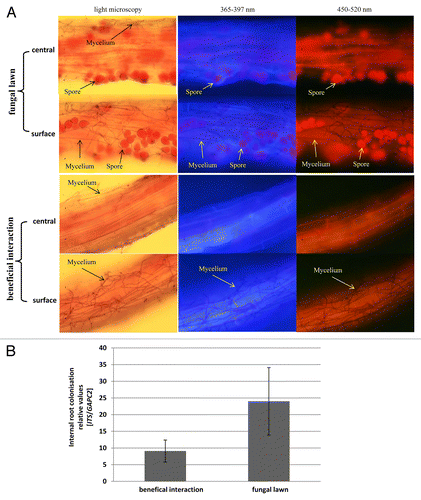
High doses of P. indica do not stimulate H2O2/ROS production
Under beneficial co-cultivation conditions, P. indica does not induce H2O2/ROS production,Citation22 while exposure of roots to stress or pathogens is often associated with a massive ROS production. NBT staining of roots and shoots did not show any obvious difference between plant material exposed to the P. indica lawn and the mock-treatment (data not shown). Therefore, we used a more sensitive assay for quantitative measurement of ROS levels based on the Amplex Red peroxidise technology (compare Methods and Material). Within 7 d, the overall ROS production in the roots of seedlings exposed to high fungal doses increased approximately 1.5–2-fold compared with seedlings grown in the absence of the fungus, but the difference was not significant (). The differences in the ROS levels in the leaves were even smaller than those in the roots (). We conclude that elevated ROS production cannot be observed even by high fungal doses. Furthermore, a ROS-inducible gene, OXI1,Citation31 is not significantly upregulated by P. indica in the shoots and roots (), and 3 independent Arabidopsis lines in which an OXI1::uidA construct was introducedCitation31 did not respond to P. indica (). For comparison, we performed the same cultivation experiment with Arabidopsis seedlings on a dense lawn of Alternaria brassicae. The measurements were performed after 24 h, since seedlings exposed to A. brassicae for 7 d were already dead (data not shown). A strong stimulation of ROS production can be measured within 24 h in the shoots and roots of the pathogen-exposed seedlings (). The OXI1 mRNA level and expression of OXI1::uidA construct was strongly upregulated after 24 h ( and ). Under these conditions, OXI1 is induced by H2O2/ROS, which accumulate after pathogen attack.Citation31 The results indicate that exposure of Arabidopsis to high doses of P. indica over a longer period of time does not lead to the induction of substantial amounts of ROS in roots and shoots.
Figure 4. Relative ROS levels in the shoots and roots of Arabidopsis seedlings that were kept on KM medium with P. indica (+ lawn) or without P. indica (− lawn) for 7 d (top) or with A. brassicae (+ lawn) or without A. brassicae (− lawn) for 24 h (bottom). The ROS levels of the “– lawn” controls were set as 1.0 and the other values expressed relative to them. Based on 6 independent experiments with 20 seedlings each, bars represent SEs.
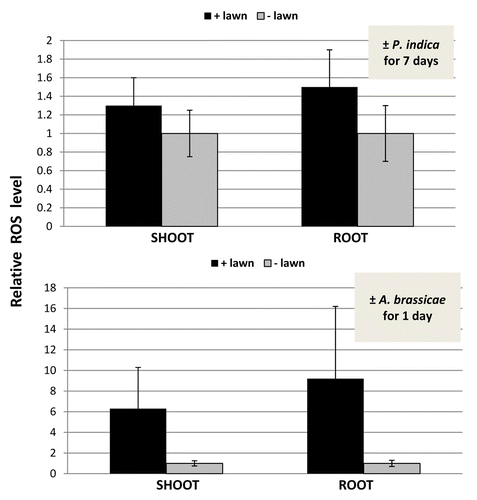
Figure 5. Relative OXI1 mRNA level in the shoots and roots of Arabidopsis seedlings that were kept on KM medium with P. indica (+ lawn) or without P. indica (− lawn) for 7 d (top) or with A. brassicae (+ lawn) or without A. brassicae (− lawn) for 24 h (bottom). The OXI1 mRNA levels in the “– lawn” samples were set as 1.0 and the other values expressed relative to them. Based on 6 independent experiments with 20 seedlings each, bars represent SEs.
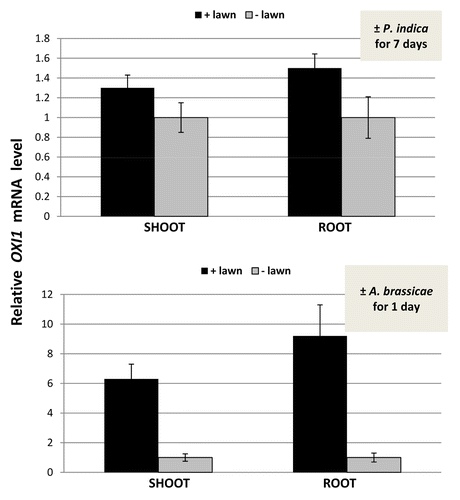
Figure 6. GUS activity in the roots of Arabidopsis seedlings that were kept on KM medium with P. indica (+ lawn) or without P. indica (− lawn) for 7 d (top) or with A. brassicae (+ lawn) or without A. brassicae (− lawn) for 24 h (bottom). The uidA gene was expressed under the control of the oxi1 promoter (compare ref. Citation31). Three independent transformants (#5, #6, and #11) were analyzed. Based on 6 independent experiments with 20 seedlings each, bars represent SEs.
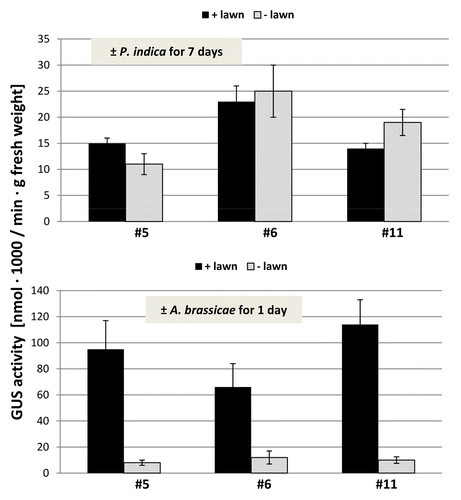
High doses of P. indica induce jasmonic acid and jasmonate-isoleucine levels in roots
Under beneficial co-cultivation conditions for 7 d, P. indica exposed and control seedlings of A. thaliana did not show differences in the jasmonic acid (JA) and jasmonate-isoleucine (JA-Ile) levels (unpublished). The JA and JA-Ile levels were > 2-fold upregulated in the roots of Arabidopsis seedlings grown on the dense fungal lawn for 7 d (). Interestingly, the stimulatory effect of the fungus was restricted to the roots and not observed for shoots of the same plant material, and the JA-Ile level in the shoots was even downregulated by the fungus (). This suggests a root-specific and not systemic effect of the high fungal doses on JA/JA-Ile levels. Furthermore, we included the jar1 and ein3 mutants into the study (compare below). Since JAR1 conjugates JA to Ile,Citation32-Citation34 the JA-Ile levels are low in the roots and shoots of both P. indica-exposed and control jar1 seedlings (). Finally, the JA level in roots of the jar1 mutant was not upregulated on the dense fungal lawn. Therefore, upregulation of JA by P. indica in the roots requires JAR1 ().
Figure 7. Phytohormone levels (jasmonic acid, JA; jasmonic acid isoleucine, JA-Ile) in the shoots and roots of wild type, ein3, and jar1 seedlings. Nine-day-old seedlings were transferred from MS medium to a plate with KM without P. indica or with a dense fungal lawn for 7 d. The phytohormone levels for shoots and roots were analyzed separately. Based on 6 independent experiments with 20 seedlings each, bars represent SEs. **; p ≤ 0.01.
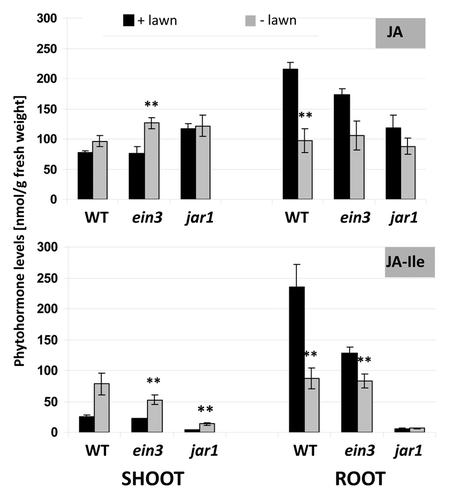
jar1 and ein3 seedlings do not suffer more than the wild-type from high fungal doses
To test whether JAR1 and EIN3 influence the performance of Arabidopsis seedlings on the dense fungal lawn, we compared wild-type, jar1, and ein3 seedlings. However, we did not observe any visible differences in the growth behavior or fitness between the 3 genotypes (). The seedlings of all 3 genotypes grow slower in the presence of the fungus. Also, the chlorophyll content and the photosynthetic parameters were not different from the wild-type seedlings (). ROS production is not stimulated on the dense fungal lawn in the jar1 and ein3 mutants, again similar to the wild-type seedlings. We observed a < 2-fold stimulation of ROS production in the roots and shoots of 2 mutants, but these data were not significantly different from the untreated controls. Also the phenotype of their roots (root lengths and root architecture) did not differ when wild-type, jar1, and ein3 seedlings were grown in the presence or absence of the fungus (data not shown). This suggests that JAR1 and EIN3 do not play an important role for the performance of Arabidopsis seedlings on a dense fungal lawn, although the JA and JA-Ile levels were increased in the roots.
High doses of P. indica strongly induce PDF1.2 and the gene for a 2-oxoglutarate and Fe2+-dependent oxygenase in the roots
PDF1.2 encodes a JA-inducible and ethylene (ET)-responsive plant defensin.Citation35 Consistent with the observation that the JA and JA-Ile levels were upregulated in the roots, we observed a > 10-fold upregulation of the PDF1.2 mRNA level in P. indica-exposed wild-type roots compared with the mRNA level in roots that were not exposed to the fungus (). A ~7-fold stimulation of the PDF1.2 mRNA level can be detected in the shoots (), although the JA and JA-Ile levels did not increase in the shoots on the dense fungal lawn. Therefore, the response in the shoots is systemic. The stimulatory effect in jar1 and ein3 seedlings was less than half compared with the wild-type, which indicates that JA/JA-Ile and also ET signaling is involved in PDF1.2 expression. Interestingly, we identified another gene (At4g10500) encoding a 2-oxoglutarate and Fe2+-dependent oxygenase that shows a similar regulation in the 3 genotypes (, cf. Discussion).
Figure 8. Fold-induction of PDF1.2 and At4g10500 transcripts levels in the roots (black) and shoots (gray) of wild-type (WT), ein3, or jar1 seedlings that were either kept on the fungal lawn of P. indica or mock-treated. Nine-day-old seedlings were transferred from MS medium to a plate with KM without or with a fungal lawn for 7 d before the RNA was extracted from their roots and shoots. The values indicate fold induction (mRNA+P. indica/mRNA-P. indica). Based on 6 independent experiments with 20 seedlings each, bars represent SEs. Errors are the sum of the individual errors.
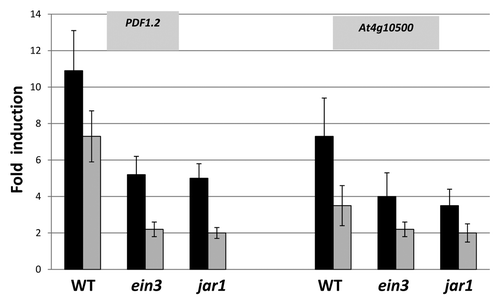
High doses of P. indica induce only a mild upregulation of defense and stress genes
Next, we tested whether defense- and stress-related genes other than PDF1.2 and At4g10500 are upregulated on the fungal lawn, since accumulation of anthocyanin in the aerial parts and roots indicates that they are stressed to some extent (). We measured an approximately 6-fold upregulation of the anthocyanin level in the roots and 4-fold upregulation in the shoots of wild-type seedlings on the fungal lawn (). Comparable results were obtained for ein3 and jar1 seedlings (), which again confirms that the mutants do not suffer more than the wild-type when exposed to the high fungal doses. The elevated anthocyanin levels are also reflected by a ~2-fold stimulation of the mRNA for the phenylalanine ammonium lyase (PAL) 2 both in roots and shoots () after 7 d on the fungal lawn. PAL2 is the main PAL isoform expressed in roots (compare Discussion). Neither the PAL2 mRNA nor the anthocyanin levels are upregulated in roots or shoots under beneficial interaction conditions. For comparison, after 24 h on an A. brassicae lawn, a 22 ± 3-fold increase in the PAL2 mRNA level was measured in the roots and a 6 ± 1-fold increase in the shoots (data not shown). Similar regulations in response to both P. indica for 7 d or to A. brassicae for 24 h were observed for the defense-related genes PR2, PR3, and ERF1 in roots and shoots: the stimulatory effects by P. indica after 7 d were below a factor of 2.5 (), whereas those by A. brassicae after 24 h were > 9-fold in the roots and > 5-fold increase in the shoots (data not shown). Again, the expression levels of PAL2, PR2, PR3, and ERF1 in P. indica-exposed or mock-treated ein3 and jar1 roots were comparable to those in the wild-type (based on 6 independent experiments with 20 plants each). Taken together, quite different defense and stress genes are only mildly upregulated in the roots and shoots of Arabidopsis seedlings even exposed to high doses of P. indica for 7 d.
Figure 9. Fold-induction of anthocyanin levels in the roots (black) and shoots (gray) of wild-type (WT), ein3 and jar1 seedlings that were either kept on the fungal lawn of P. indica or mock-treated. Nine-day-old seedlings were transferred from MS medium to a plate with KM without or with a fungal lawn for 7 d before the anthocyanin level was determined for the roots and shoots. The values indicate fold induction (A530+P. indica/A530-P. indica). For experimental details cf. Material and Methods. Based on 9 independent experiments with 20 seedlings each, bars represent SEs. Errors are the sum of the individual errors.
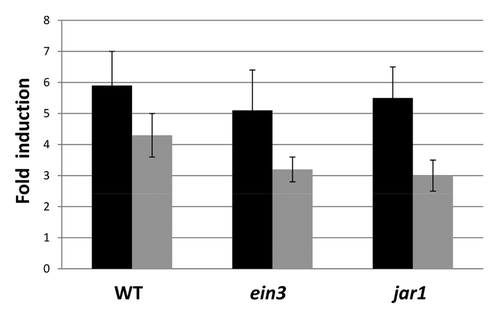
Table 2. Stress- and defense-related genes and their regulation in the roots (and shoots) after growth of wild-type (WT), ein3, or jar1 seedlings on a dense fungal lawn of P. indica for 7 d ()
To test whether other genes with stress- and defense-related functions are upregulated in Arabidopsis roots exposed to the fungal lawn of P. indica, we tested genes that were not upregulated under beneficial co-cultivation conditions of the 2 symbionts, but that responded > 10-fold to unbalances in the symbiontic interaction (Vahabi et al., manuscript in preparation). Interestingly, only At4g10500 () showed a strong response to the fungal lawn, while all other genes were upregulated less than 2.5-fold () and thus comparable to PAL2, PR2, PR3, and ERF1. This includes genes for P450 enzymes, the calmodulin-binding protein CBP60 g, a chitinase, the stress-related RmlC-like cupins protein At5g38910, the trypsin inhibitor ATT1, a glutathione-S-transferase (At1g02930), and the GDSL-lipase GLIP1. Several of the genes are involved in JA (CBP60 g, CYP82C2), ABA (CBP60 g), cytokinin (2-oxoglutarate and Fe2+-dependent oxygenase), and ET (GLIP1) signaling (compare Discussion). We conclude that growth on the fungal lawn does not induce a massive stress response in the seedlings (compare Discussion).
Discussion
The overall observation is that growth of Arabidopsis seedlings on a dense fungal lawn does not result in a higher fungal concentration in the root material. The performance of the plants, measured on the basis of the efficiency of the photosynthetic electron flow, is not impaired on the dense fungal lawn, compared with plants grown without the fungus or under beneficial co-cultivation conditions. We also did not observe a massive defense or stress response in roots or shoots, although the growth rate of the seedlings is reduced compared with control seedlings grown on the KM without the fungus ( and ). The reduced growth rate may be caused by inhibitory factors from the fungus or by the fact that the seedlings do not have direct access to essential nutrients in the agar. Since accumulation of chlorophyll per gram leaf tissue and the efficiency of the photosynthetic electron transport is not or barely impaired on the fungal lawn (), the plants are fit and do not suffer. We cannot detect significant increases in H2O2/ROS production (), the stimulation of the H2O2 inducible OXI1 gene (), and the activation of the H2O2-inducible oxi1 promoter () in the P. indica-exposed tissue, which is consistent with previous observations.Citation22,Citation36 Furthermore, classical defense genes such as PR2 (encodes a β-1,3-glucanase), PR3 (encodes a basic chitinase), and ERF1 (encodes an ET responsive element binding factor) are only mildly upregulated (). ERF1 has been included in this study because it has previously been demonstrated that this transcription factor gene is involved in the Arabidopsis/P. indica interaction.Citation24,Citation25 The elevated anthocyanin and PAL2 mRNA levels in the P. indica-exposed seedlings (; ) suggest that the seedlings suffer to some extent, although this does not have an effect on the efficiency of the photosynthetic electron transport in the leaves (). Four PAL genes are present in the Arabidopsis genome,Citation37 and they respond to a multitude of environmental stress stimuli including pathogen infection, wounding, nutrient depletion, UV irradiation, or extreme temperatures.Citation38-Citation40 PAL2 is mainly expressed in roots, and we could show that this gene is only marginally upregulated on the P. indica lawn (), when compared with the induction by A. brassicae. Furthermore, growth of wild-type, jar1, and ein3 seedlings on the dense fungal lawn was reduced compared with seedlings that were grown without the fungus, but the reduction was similar for the 3 genotypes, and the performance of the seedlings were identical (). Since also stimulation of anthocyanin, and of the PAL2, PR2, PR3, and ERF1 mRNA levels were comparable for the 3 genotypes (), neither EIN3 nor JAR1 have a significant influence on the performance of the seedlings on the fungal lawn. The lack of massive defense gene activation in the roots exposed to a dense fungal lawn suggests that P. indica is either unable to release microbe-associated molecular patterns that activate defense processes, or the fungus activates mechanisms to repress their activation. Jacobs et al.Citation23 suggested that P. indica has established efficient mechanism(s) to bypass or supress host immunity, since the fungus is confronted with a functional root immune system. Either this is also the case for our co-cultivation conditions, or the 2 co-cultivation conditions cannot be compared.
The results shown here open the question why the cultivation of A. thaliana on a dense fungal layer does not result in a massive infection (). The roots may either have established mechanisms to control fungal invasion for their protection against overcolonization, or intracellular root colonization follows a highly regulated program that is independent of the fungal concentration around the roots and in the rhizosphere. The absence of a massive defense response of the roots against P. indica is consistent with the colonization data (). For mycorrhizal interaction, empirical studies have shown that mycorrhizal colonization intensity exhibits substantial heritable genetic variation within plant and fungal species and are influenced by plant genotype/fungal genotype interactions, suggesting the potential for ongoing coevolutionary selection.Citation41 It might be possible that plant genetic traits limit root colonization in the P. indica/Arabidopsis symbiosis. It has also been discussed that root colonization maybe controlled by a phosphate/carbon exchange between the 2 partners, and that limitations in any of the nutrients may limit the colonization process.Citation42 In mycorrhizal interactions, exchange of small molecules from both partners may determine the degree of root colonization.Citation43 Candidates for small molecules from the fungus that may be important for the control of root colonization are discussed, after the entire P. indica genome has been sequenced.Citation44,Citation45 Finally, JA and salicylic acid signaling during specific stages of root colonization may control a balance between compatibility and defense in mutualistic interactions,Citation46 and this might be also the reason why the level of the active form of JA increases on the dense fungal lawn. Since the jar1 mutant does not suffer on the lawn during the 7 d of co-cultivation, it appears that this phytohormone is not required during early phases of the interaction. However, the phytohormone might be required during later phases of the interaction. Finally, Lahmann and ZuccaroCitation47 and others describe a biphasic colonization strategy of barley and Arabidopsis roots by P. indica upon penetration of the root: Perturbance of plant hormone homeostasis and secretion of fungal lectins and other small proteins (effectors) may be involved in the evasion and suppression of host defenses at these early colonization steps. At later stages, P. indica is found more often in moribund host cells where it secretes hydrolytic enzymes. This strategy of colonizing plants is reminiscent of that of hemibiotrophic fungi, although a defined shift to necrotrophy with massive host cell death is missing. It is reasonable to assume that the plant has established mechanisms that specifically counteract cell-death inducing processes by restricting fungal invasion or growth inside the plant tissue, in particular if the symbiosis is beneficial for the plant.
We identified only 2 genes that were strongly upregulated in response to the fungal lawn. One of these genes is PDF1.2 (). The response is consistent with elevated JA and JA-Ile levels (). PDF1.2 is a marker gene for JA and ET signaling.Citation35 Since the PDF1.2 mRNA level is less upregulated in jar1 and ein3 roots compared with wild-type roots (), it is likely that both phytohormones are involved in the induction. PDF1.2 is induced both locally at the site of infection by incompatible fungal pathogens and systemically in remote noninoculated regions of the plant.Citation48 This activation occurs via the JA/ET rather than the SA pathway.Citation35,Citation48 Necrotrophic fungi can inhibit JA-induced defense gene activation, as recently shown for SSITL, an effector from Sclerotinia sclerotiorum that plays a significant role in the suppression of JA/ET signal pathway-mediated resistance at the early stage of infection.Citation49 Interestingly, PDF1.2 was also upregulated in the leaves of P. indica-exposed seedlings (), suggesting a systemic effect, although the JA/JA-Ile levels were not higher in the leaves (). Suza et al.Citation34 have shown that jar1 has little or no impact on several wound-induced genes. To test for a possible JAR1 role in systemic induction, younger unwounded leaves from the same wounded plants were examined. PDF1.2 transcripts accumulated in both wounded and unwounded leaves, and jar1 did not affect the timing or magnitude of accumulation. This provides an example for a JAR1-independent regulation of PDF1.2, and might be comparable to the upregulation of PDF1.2 in leaves where the JA/JA-Ile levels were not elevated, as well as to the upregulation of PDF1.2 in jar1 plants on the fungal lawn ( and ). Finally, ein3 usually shows a lower PDF1.2 expression compared with the wild type,Citation50 consistent with our observations ().
Whether PDF1.2 regulation is a defense response or a general stress response under our co-cultivation conditions is unclear. In barley P. indica elicits a non-specific defense reaction by upregulation of a multiplicity of stress responsive genes.Citation51 If this is also true for the interaction studies described here, the fungus induce only a mild stress response in Arabidopsis roots, which has little effect on plant performance and no effect on the efficiency of the photosynthetic electron transport (). Interestingly, the 2-oxoglutarate and Fe2+-dependent oxygenase-encoding gene At4g10500 shows the same regulation as PDF1.2 on the fungal lawn (). The gene is stimulated in response to calcium stress,Citation52 senescence,Citation53 and is involved in cytokinin signaling.Citation54 The message is also upregulated in powdery mildew resistant 4 (pmr4), a mutant lacking pathogen-induced callose.Citation55 Further studies are required to understand the role of this protein in the symbiotic interaction.
We also analyzed the expression of a set of less studied defense- and stress-related genes, but all of them were barely or not induced in the roots in response to the dense fungal lawn (). ERF1, a target transcription factor of both JA and ET signaling, is important for PDF1.2 activationCitation56,Citation57 and involved in the Arabidopsis/P. indica interaction.Citation24,Citation25 CBP60 g is a calmodulin-binding protein that has previously been described to respond to P. indica under beneficial co-cultivation conditions in wild-type roots.Citation58 The protein is a positive regulator of both disease resistance and drought tolerance in Arabidopsis.Citation59 Overexpression of CBP60 g caused elevated SA accumulation, increased expression of the defense genes, enhanced resistance to Pseudomonas syringae, hypersensitivity to abscisic acid (ABA), and enhanced tolerance to drought stress.Citation59 CBP60 g has a partially redundant role with SAD1, which affect defense responses in addition to SA production.Citation60,Citation61 The P450 protein CYP82C2 (At4g31970) modulates JA-induced root growth inhibition, defense gene expression, and indole glucosinolate biosynthesis.Citation62 CYP82C2 affects JA-induced accumulation of tryptophan, but not the JA-induced auxin- or pathogen-induced camalexin, and thus acts in the metabolism of tryptophan-derived secondary metabolites under conditions in which JA levels are elevated.Citation62 The enzyme is also involved in the systemic resistance response induced by the root-colonizing Pseudomonas fluorescens strain SS101 against several bacterial pathogens, including Pseudomonas syringae pv tomato, and the insect pest Spodoptera exigua.Citation63 The RmlC-like cupins protein At5g38910 is an apoplastic manganese ion binding protein with potential nutrient reservoir activity. The mRNA was induced by cesium stressCitation64 as well as treatments with 9 other abiotic stresses.Citation65 The mRNA for the plasma membrane-associated protein At5g25260 is induced in response to geminivirusCitation66 and Pseudomonas syringaeCitation67 infections, and involved in ABA-mediated defense responses.Citation67 At4g11170 is a defense protein, which responds to ozone and shows a high expression in stems, roots, and stamen.Citation68 The failure of this gene to respond to the dense fungal lawn is consistent with the observation that the H2O2/ROS levels do not increase. The P450 enzyme CYP81F2 (At5g57220) is involved in glucosinolate biosynthesis and Arabidopsis innate immune responses.Citation69 The GDSL lipase-like 1 (At5g40990) regulates systemic resistance associated with ET signalingCitation70 and elicits both local and systemic resistance. GLIP1-overexpressors exhibited enhanced resistance against necrotrophic pathogens, including A. brassicicola and Erwinia carotovora, and the hemibiotrophic pathogen Pseudomonas syringae.Citation70 The FAD-binding berberine protein At1g26390 is an oxidoreductase in the endomembrane system that is upregulated in response to various biotic and abiotic stresses.Citation71-Citation73 The identified peroxidase At5g39580 is involved in various defense responses.Citation74 Finally, the P450 enzyme CYP1A12 is involved in camalexin synthesis and thus crucial for defense responses against pathogens and herbivores.Citation75 All these genes have been identified in previous studies to be upregulated > 10-fold when the symbiotic interaction between P. indica and Arabidopsis is disturbed (Vahabi et al., unpublished). The lack of regulation of these genes on the dense fungal lawn suggests that the interaction of the 2 symbionts is not harmful. The exact function of these proteins in the P. indica/Arabidopsis symbiosis under unfavorable co-cultivation conditions is currently under study.
Under beneficial co-cultivation conditions, defense and stress genes including PDF1.2 are not upregulated in Arabidopsis roots.Citation3,Citation18,Citation19,Citation21,Citation22,Citation24-Citation26 However, Arabidopsis mutants, which are impaired in establishing a beneficial interaction or are unable to maintain a long-term harmony between the 2 symbionts, activate a mild defense response against P. indica.Citation18,Citation19,Citation21,Citation22,Citation24-Citation26 In particular, PDF1.2 has been reported repeatedly as being upregulated under these conditions.Citation19 Unbalances in the interaction are often accompanied by an increase in root colonization.Citation19 This has also been observed for other mutualistic interactions, e.g., for mycorrhiza formationCitation4,Citation46,Citation76,Citation77 and the interaction of plant-growth promoting microbes with roots.Citation78,Citation79 The results shown here are somewhat surprising since they show that Arabidopsis seedlings can be exposed to a high dosis of a beneficial fungus without a significant defense gene activation from the host against the microbe or shift from mutualism to parasitism. The plants perform quite well. The available tools and genes described here allow us to investigate the signaling between the symbionts in greater details. In particular, the results demonstrate that these genes are not simply upregulated if the roots are surrounded by high fungal doses of P. indica.
Material and Methods
Growth conditions of plants and fungus
Wild-type or mutant (jar1, obtained from Dr. J. Vadassery, Max-Planck-Institute for Chemical Ecology and ein3Citation24) Arabidopsis thaliana seeds were surface-sterilized and placed on petri dishes containing MS nutrient medium.Citation80 After cold treatment at 4 °C for 48 h, plates were incubated for 7 d at 22 °C under continuous illumination (75 µmol m−2 sec−1). P. indica was cultured as described previouslyCitation20,Citation81,Citation82 on KM modified by Pham et al.Citation82 For solid medium 1% (w/v) agar was included. Alternaria brassicae cultivation has been described in details in Johnson et al.Citation83
Nine-day-old wild-type, ein3, or jar1 seedlings were directly transferred from MS medium to a plate with a fungal lawn of P. indica.Citation83 The fungal lawn was obtained by placing a fungal plug on KM and the fungus was allowed to grow for 14 d at 24 °C in the dark, before the seedlings were transferred to the plate. Control seedlings were transferred to KM without the fungus. The plates were incubated for 7 d at 22 °C under continuous illumination (µmol m−2 sec−1) from above.Citation83 The co-cultivation experiments with A. brassicae were performed under the same conditions, except that the fungus colonized the plate only for 5 dCitation83 and co-cultivation was terminated after 24 h. Fresh weights were determined directly after harvest. Beneficial co-cultivation conditions of the 2 symbionts for 7 d was performed on PNM medium, because growth of the fungus on Kaefer medium is too fast for a balanced interaction of P. indica and A. thaliana.Citation20 A detailed protocol is given in Johnson et al.Citation83
Staining of fungal mycelium and spores
Fuchsin acid and trypan blue staining of fungal hyphae in Arabidopsis roots was described in details in Vahabi et al.Citation84 In brief, Arabidopsis roots co-cultivated with P. indica were collected and intensively washed with distilled water. After incubation in fuchsin acid solution for 10 min, the material was washed with destilled water for 1 min. They were then stained with trypan blue (0.0001mg/ml) solution for 3 min and washed again for 1 min.
After addition of 50 μl GL solution (glycerol:lactic acid:water, 1:1:1) on a glass slide, the sample was protected with a glass cover, before analysis by light and fluorescent microscopy using different wavelengths. The localization of hyphae and spores on the surface of the roots or in the root material was distinguished by scanning through the plant material.
RNA extraction and cDNA synthesis
RNA was isolated from the roots or shoots with an RNA isolation kit (RNeasy, Qiagen, Hilden, Germany). For quantitative RT-PCR, RNA from Arabidopsis mock-treated roots/shoots and roots/shoots treated with a high amount of fungal hyphae were used. Reverse transcription of 1 µg of total RNA was performed with an oligodT Primer. First strand synthesis was performed with a kit from Qiagen (Omniscript RT Kit, Qiagen, Hilden, Germany).
Real-time PCR
Real-time quantitative RT-PCR was performed using the iCycler iQ real time PCR detection system and iCycler software version 2.2 (Bio-Rad, Munich, Germany). For the amplification of the PCR products, iQ SYBR Supermix from Bio-Rad was used according to the manufacturer’s instructions in a final volume of 23 µl. The iCycler was programmed to 95 °C 3 min, 40 × (94 °C 30 s, 57 °C 30 s, 72 °C 40 s), 72 °C 10 min followed by a melting curve program (50–85 °C in increasing steps of 0.5 °C). All reactions were repeated at least 4 times. The mRNA levels for each cDNA probe were normalized with respect to the GAPC2 message level. Fold induction values were calculated with the ΔΔCP equation of Pfaffl.Citation85 P. indica cDNA was detected with primers for the ITS region (CAACACATGT GCACGTCGAT; CCAATGTGCA TTCAGAACGA). Root colonization was determined relative to the plant GAPC2 cDNA levels.
The following Arabidopsis primer pairs were used:
Phytohormone measurement
Phytohormones were extracted by homogenizing approximately 100 mg of Arabidopsis material and adding 1 ml ethylacetate spiked with internal standards [D6-ABA, D2-JA, D4- SA, and 13C6-JA-Ile (200 ng/each)]. Samples were homogenized twice by reciprocal shaking (FastPrep speed 6.5) for 45 s and centrifuged at 13 000 rpm for 20 min at 4 °C. The supernatant was transferred to a fresh 2 ml microcentrifuge tube and the extraction was repeated by adding 1 ml ethylacetate without internal standards. The organic phases were pooled and evaporated under reduced pressure. The dried sample was dissolved in 500 μl 70% methanol, vortexed, and centrifuged at 13 000 rpm for 10 min. 100 μl of the supernatant were transferred into an HPLC vial with insert and the samples were analyzed by liquid chromatography tandem mass spectormetry system (Varian 1200L Triple-Quadrupol-MS). Ten μl of each sample were injected onto a ProntoSIL column (C18; 5μm, 50 × 2mm). The mobile phase comprised solvent A (0.05% formic acid in water) and solvent B (0.05% formic acid in acetonitrile). Compounds were ionized by electrospray ionization and analyzed in the negative mode by multiple reaction monitoring (MRM).
NBT stain
Arabidopsis seedlings were grown as described before. After 7 d on the fungal lawn, the roots and shoots were stained for 5 min in a solution containing 2 mM nitrobluetetrazolium (NBT; Sigma Aldrich) in water. The reaction was stopped by washing the roots with water. Roots were evaluated under the Axiovert 135 (Carl Zeiss MicroImaging GmbH, Jena, Germany).
ROS measurements
Quantitative ROS measurements from leaves and roots were performed using the Amplex Red hydrogenperoxide/peroxidase assay kit (Molecular Probes) according to the manufacturer’s instructions (http://tools.invitrogen.com/content/sfs/manuals/mp22188.pdf) using the substrate carboxy-H2DFFDA (Molecular Probes) according to the manufacturer’s instructions (https://tools.invitrogen.com/content/sfs/manuals/mp36103.pdf). Leaf sections of 0.5–1 mm width and root sections of 2–3 cm length were incubated in 20 μM carboxy-H2DFFDA prepared in KRPG buffer for 30 min in the dark. The fluorescence intensity was quantified with a fluorescence microplate reader (TECAN Infinite 200) with an excitation at 485 nm and emission at 530 nm. The reaction mixture without the substrate and plant material served as control.
Anthocyanin measurements
Roots and shoots were ground in liquid nitrogen and extracted with 80% methanol/5% HCl in the dark and incubated overnight at 4 °C. After centrifugation (15.000 g, 20 min) the supernatant was removed and the anthocyanin concentration was determined spectrophotometrically with a Lambda 12 spectrophotometer (Perkin-Elmer Company). The amount of anthocyanin is expressed as A530/mg fresh weight.
GUS assay
Oxi promoter::uidA linesCitation31 were grown on MS media with the appropriate amount of kanamycin for 9 d and were then exposed for 5 d to the treatments. Whole plants were harvested and ground with 500 µl of lysis buffer (100 mM Na3PO4, pH 7.0; 500 mM EDTA; 0,1% Triton X-100; 0,1% lauroyl sarcosin; 10 mM β-mercaptoethanol). The homogenate was clarified by centrifugation and 10 µl of the supernatant was used for assaying GUS activity in a total volume of 100 µl with 1 mM methylumbelliferyl-β-D-glucoside in lysis buffer. The enzyme reaction was performed at 37 °C and stopped after 30 min with 900 µl 100 mM Na2CO3. Samples were measured with the fluorometer VersoFluor from Bio-Rad (Bio-Rad, Munich, Germany) after setting the range according to the manufacturer’s instructions. The system of reference was an equal amount of fresh weight.
Measurement of chlorophyll and photosynthesis parameters
Measurement of chlorophyll content was performed as described in Porra et al.Citation86 The Arabidopsis seedlings were dark-adapted for 15 min and then the chlorophyll fluorescence was measured using a FluorCam 700F (Photon System Instruments, Czech Republic). Program parameters of FluorCam were set according to Wagner et al.Citation29 Photosynthesis parameters, quantum yield of PSII (ΦPSII), maximum quantum yield of PSII (Fv/Fm), photochemical quenching (qP), and non-photochemical quenching (NPQ) were calculated according to Maxwell and Johnson.Citation28 False color images of the seedlings in plates were obtained as described by Wagner et al.Citation29 Chlorophyll fluorescence images representing Fs/Fm values are shown, whereas blue represents low Fs/Fm values above a threshold of 0.06 and red represents high Fs/Fm values with an upper threshold limit of 0.17.
Acknowledgments
We thank Dr. H. Hirt for providing the OXI1 promoter::GUS lines, Dr. Rothe and Dr. I. T. Baldwin (Max Planck Institute for Chemical Ecology, Jena, Germany) for supporting the phytohormone measurements, Sarah Mußbach and Claudia Röppischer for excellent technical assistance, and the Jena School for Microbial Communication (JSMC) for funding.
Disclosure of Potential Conflicts of Interest
No potential conflicts of interest were disclosed.
References
- Kogel KH, Franken P, Hückelhoven R. Endophyte or parasite--what decides?. Curr Opin Plant Biol 2006; 9:358 - 63; http://dx.doi.org/10.1016/j.pbi.2006.05.001; PMID: 16713330
- Paszkowski U. Mutualism and parasitism: the yin and yang of plant symbioses. Curr Opin Plant Biol 2006; 9:364 - 70; http://dx.doi.org/10.1016/j.pbi.2006.05.008; PMID: 16713732
- Johnson JM, Oelmüller R. Mutualism or parasitism: life in an unstable continuum. Endocytobiol Cell Res 2009; 19:81 - 111
- Tanaka A, Christensen MJ, Takemoto D, Park P, Scott B. Reactive oxygen species play a role in regulating a fungus-perennial ryegrass mutualistic interaction. Plant Cell 2006; 18:1052 - 66; http://dx.doi.org/10.1105/tpc.105.039263; PMID: 16517760
- Harrison MJ. Cellular programs for arbuscular mycorrhizal symbiosis. Curr Opin Plant Biol 2012; 15:691 - 8; http://dx.doi.org/10.1016/j.pbi.2012.08.010; PMID: 23036821
- Oldroyd GE. Speak, friend, and enter: signalling systems that promote beneficial symbiotic associations in plants. Nat Rev Microbiol 2013; 11:252 - 63; http://dx.doi.org/10.1038/nrmicro2990; PMID: 23493145
- Nehls U, Göhringer F, Wittulsky S, Dietz S. Fungal carbohydrate support in the ectomycorrhizal symbiosis: a review. Plant Biol (Stuttg) 2010; 12:292 - 301; http://dx.doi.org/10.1111/j.1438-8677.2009.00312.x; PMID: 20398236
- Campos-Soriano L, Segundo BS. New insights into the signaling pathways controlling defense gene expression in rice roots during the arbuscular mycorrhizal symbiosis. Plant Signal Behav 2011; 6:553 - 7; http://dx.doi.org/10.4161/psb.6.4.14914; PMID: 21422823
- León-Morcillo R, Ocampo J, García-Garrido JM. Plant 9-lox oxylipin metabolism in response to arbuscular mycorrhiza. Plant Signal Behav 2012; 7:1584 - 8; http://dx.doi.org/10.4161/psb.22098; PMID: 22301955
- Fester T, Hause G. Accumulation of reactive oxygen species in arbuscular mycorrhizal roots. Mycorrhiza 2005; 15:373 - 9; http://dx.doi.org/10.1007/s00572-005-0363-4; PMID: 15875223
- Harrison MJ. Signaling in the arbuscular mycorrhizal symbiosis. Annu Rev Microbiol 2005; 59:19 - 42; http://dx.doi.org/10.1146/annurev.micro.58.030603.123749; PMID: 16153162
- Jung SC, Martinez-Medina A, Lopez-Raez JA, Pozo MJ. Mycorrhiza-induced resistance and priming of plant defenses. J Chem Ecol 2012; 38:651 - 64; http://dx.doi.org/10.1007/s10886-012-0134-6; PMID: 22623151
- Campos-Soriano L, García-Garrido JM, San Segundo B. Activation of basal defense mechanisms of rice plants by Glomus intraradices does not affect the arbuscular mycorrhizal symbiosis. New Phytol 2010; 188:597 - 614; http://dx.doi.org/10.1111/j.1469-8137.2010.03386.x; PMID: 20659300
- Oldroyd GE, Harrison MJ, Paszkowski U. Reprogramming plant cells for endosymbiosis. Science 2009; 324:753 - 4; http://dx.doi.org/10.1126/science.1171644; PMID: 19423817
- Güimil S, Chang HS, Zhu T, Sesma A, Osbourn A, Roux C, Ioannidis V, Oakeley EJ, Docquier M, Descombes P, et al. Comparative transcriptomics of rice reveals an ancient pattern of response to microbial colonization. Proc Natl Acad Sci U S A 2005; 102:8066 - 70; http://dx.doi.org/10.1073/pnas.0502999102; PMID: 15905328
- Barto EK, Weidenhamer JD, Cipollini D, Rillig MC. Fungal superhighways: do common mycorrhizal networks enhance below ground communication?. Trends Plant Sci 2012; 17:633 - 7; http://dx.doi.org/10.1016/j.tplants.2012.06.007; PMID: 22818769
- Singh A, Singh A, Kumari M, Rai MK, Varma A. Importance of Piriformospora indica - A novel symbiotic mycorrhiza-like fungus: an overview. Indian J Biotechnol 2003; 2:65 - 75
- Oelmüller R, Sherameti I, Tripathi S, Varma A. Piriformospora indica, a cultivable root endophyte with multiple biotechnological applications. Symbiosis 2009; 49:1 - 17; http://dx.doi.org/10.1007/s13199-009-0009-y
- Nongbri PL, Vahabi K, Mrozinska A, Seebald E, Sun C, Sherameti I, Johnson JM, Oelmüller R. Balancing defense and growth - Analyses of the beneficial symbiosis between Piriformospora indica and Arabidopsis thaliana.. Symbiosis 2012; 58:17 - 28; http://dx.doi.org/10.1007/s13199-012-0209-8
- Peškan-Berghöfer T, Shahollari B, Giong PH, Hehl S, Markert C, Blanke V, Kost G, Varma A, Oelmüller R. Association of Piriformospora indica with Arabidopsis thaliana roots represents a novel system to study beneficial plant-microbe interactions and involves early plant protein modifications in the endoplasmic reticulum and at the plasma membrane. Physiol Plant 2004; 122:465 - 77; http://dx.doi.org/10.1111/j.1399-3054.2004.00424.x
- Sherameti I, Venus Y, Drzewiecki C, Tripathi S, Dan VM, Nitz I, Varma A, Grundler FM, Oelmüller R. PYK10, a beta-glucosidase located in the endoplasmatic reticulum, is crucial for the beneficial interaction between Arabidopsis thaliana and the endophytic fungus Piriformospora indica.. Plant J 2008; 54:428 - 39; http://dx.doi.org/10.1111/j.1365-313X.2008.03424.x; PMID: 18248598
- Camehl I, Drzewiecki C, Vadassery J, Shahollari B, Sherameti I, Forzani C, Munnik T, Hirt H, Oelmüller R. The OXI1 kinase pathway mediates Piriformospora indica-induced growth promotion in Arabidopsis.. PLoS Pathog 2011; 7:e1002051; http://dx.doi.org/10.1371/journal.ppat.1002051; PMID: 21625539
- Jacobs S, Zechmann B, Molitor A, Trujillo M, Petutschnig E, Lipka V, Kogel KH, Schäfer P. Broad-spectrum suppression of innate immunity is required for colonization of Arabidopsis roots by the fungus Piriformospora indica.. Plant Physiol 2011; 156:726 - 40; http://dx.doi.org/10.1104/pp.111.176446; PMID: 21474434
- Camehl I, Sherameti I, Venus Y, Bethke G, Varma A, Lee J, Oelmüller R. Ethylene signalling and ethylene-targeted transcription factors are required to balance beneficial and nonbeneficial traits in the symbiosis between the endophytic fungus Piriformospora indica and Arabidopsis thaliana.. New Phytol 2010; 185:1062 - 73; http://dx.doi.org/10.1111/j.1469-8137.2009.03149.x; PMID: 20085621
- Camehl I, Oelmüller R. Do ethylene response factors -9 and -14 repress PR gene expression in the interaction between Piriformospora indica and Arabidopsis?. Plant Signal Behav 2010; 5:932 - 6; http://dx.doi.org/10.4161/psb.5.8.12036; PMID: 20505369
- Nongbri PL, Johnson JM, Sherameti I, Glawischnig E, Halkier BA, Oelmüller R. Indole-3-acetaldoxime-derived compounds restrict root colonization in the beneficial interaction between Arabidopsis roots and the endophyte Piriformospora indica.. Mol Plant Microbe Interact 2012; 25:1186 - 97; http://dx.doi.org/10.1094/MPMI-03-12-0071-R; PMID: 22852809
- Varma A, Savita Verma, Sudha, Sahay N, Butehorn B, Franken P. Piriformospora indica, a cultivable plant-growth-promoting root endophyte. Appl Environ Microbiol 1999; 65:2741 - 4; PMID: 10347070
- Maxwell K, Johnson GN. Chlorophyll fluorescence--a practical guide. J Exp Bot 2000; 51:659 - 68; http://dx.doi.org/10.1093/jexbot/51.345.659; PMID: 10938857
- Wagner R, Dietzel L, Bräutigam K, Fischer W, Pfannschmidt T. The long-term response to fluctuating light quality is an important and distinct light acclimation mechanism that supports survival of Arabidopsis thaliana under low light conditions. Planta 2008; 228:573 - 87; http://dx.doi.org/10.1007/s00425-008-0760-y; PMID: 18542996
- Sherameti I, Tripathi S, Varma A, Oelmüller R. The root-colonizing endophyte Pirifomospora indica confers drought tolerance in Arabidopsis by stimulating the expression of drought stress-related genes in leaves. Mol Plant Microbe Interact 2008; 21:799 - 807; http://dx.doi.org/10.1094/MPMI-21-6-0799; PMID: 18624643
- Rentel MC, Lecourieux D, Ouaked F, Usher SL, Petersen L, Okamoto H, Knight H, Peck SC, Grierson CS, Hirt H, et al. OXI1 kinase is necessary for oxidative burst-mediated signalling in Arabidopsis.. Nature 2004; 427:858 - 61; http://dx.doi.org/10.1038/nature02353; PMID: 14985766
- Staswick PE, Tiryaki I, Rowe ML. Jasmonate response locus JAR1 and several related Arabidopsis genes encode enzymes of the firefly luciferase superfamily that show activity on jasmonic, salicylic, and indole-3-acetic acids in an assay for adenylation. Plant Cell 2002; 14:1405 - 15; http://dx.doi.org/10.1105/tpc.000885; PMID: 12084835
- Staswick PE, Tiryaki I. The oxylipin signal jasmonic acid is activated by an enzyme that conjugates it to isoleucine in Arabidopsis.. Plant Cell 2004; 16:2117 - 27; http://dx.doi.org/10.1105/tpc.104.023549; PMID: 15258265
- Suza WP, Staswick PE. The role of JAR1 in Jasmonoyl-L: -isoleucine production during Arabidopsis wound response. Planta 2008; 227:1221 - 32; http://dx.doi.org/10.1007/s00425-008-0694-4; PMID: 18247047
- Penninckx IA, Thomma BP, Buchala A, Métraux JP, Broekaert WF. Concomitant activation of jasmonate and ethylene response pathways is required for induction of a plant defensin gene in Arabidopsis.. Plant Cell 1998; 10:2103 - 13; PMID: 9836748
- Vadassery J, Ranf S, Drzewiecki C, Mithöfer A, Mazars C, Scheel D, Lee J, Oelmüller R. A cell wall extract from the endophytic fungus Piriformospora indica promotes growth of Arabidopsis seedlings and induces intracellular calcium elevation in roots. Plant J 2009; 59:193 - 206; http://dx.doi.org/10.1111/j.1365-313X.2009.03867.x; PMID: 19392691
- Huang J, Gu M, Lai Z, Fan B, Shi K, Zhou YH, Yu JQ, Chen Z. Functional analysis of the Arabidopsis PAL gene family in plant growth, development, and response to environmental stress. Plant Physiol 2010; 153:1526 - 38; http://dx.doi.org/10.1104/pp.110.157370; PMID: 20566705
- Edwards K, Cramer CL, Bolwell GP, Dixon RA, Schuch W, Lamb CJ. Rapid transient induction of phenylalanine ammonia-lyase mRNA in elicitor-treated bean cells. Proc Natl Acad Sci U S A 1985; 82:6731 - 5; http://dx.doi.org/10.1073/pnas.82.20.6731; PMID: 16593613
- Liang XW, Dron M, Schmid J, Dixon RA, Lamb CJ. Developmental and environmental regulation of a phenylalanine ammonia-lyase-beta-glucuronidase gene fusion in transgenic tobacco plants. Proc Natl Acad Sci U S A 1989; 86:9284 - 8; http://dx.doi.org/10.1073/pnas.86.23.9284; PMID: 2594769
- Dixon RA, Paiva NL. Stress-Induced Phenylpropanoid Metabolism. Plant Cell 1995; 7:1085 - 97; PMID: 12242399
- Hoeksema JD. Ongoing coevolution in mycorrhizal interactions. New Phytol 2010; 187:286 - 300; http://dx.doi.org/10.1111/j.1469-8137.2010.03305.x; PMID: 20524992
- Smith SE, Smith FA. Fresh perspectives on the roles of arbuscular mycorrhizal fungi in plant nutrition and growth. Mycologia 2012; 104:1 - 13; http://dx.doi.org/10.3852/11-229; PMID: 21933929
- Bucher M, Wegmüller S, Drissner D. Chasing the structures of small molecules in arbuscular mycorrhizal signaling. Curr Opin Plant Biol 2009; 12:500 - 7; http://dx.doi.org/10.1016/j.pbi.2009.06.001; PMID: 19576840
- Zuccaro A, Lahrmann U, Güldener U, Langen G, Pfiffi S, Biedenkopf D, Wong P, Samans B, Grimm C, Basiewicz M, et al. Endophytic life strategies decoded by genome and transcriptome analyses of the mutualistic root symbiont Piriformospora indica.. PLoS Pathog 2011; 7:e1002290; http://dx.doi.org/10.1371/journal.ppat.1002290; PMID: 22022265
- Rafiqi M, Jelonek L, Akum NF, Zhang F, Kogel KH. Effector candidates in the secretome of Piriformospora indica, a ubiquitous plant-associated fungus. Front Plant Sci 2013; 4:228; http://dx.doi.org/10.3389/fpls.2013.00228; PMID: 23874344
- Gutjahr C, Paszkowski U. Weights in the balance: jasmonic acid and salicylic acid signaling in root-biotroph interactions. Mol Plant Microbe Interact 2009; 22:763 - 72; http://dx.doi.org/10.1094/MPMI-22-7-0763; PMID: 19522558
- Lahrmann U, Zuccaro A. Opprimo ergo sum--evasion and suppression in the root endophytic fungus Piriformospora indica.. Mol Plant Microbe Interact 2012; 25:727 - 37; http://dx.doi.org/10.1094/MPMI-11-11-0291; PMID: 22352718
- Penninckx IA, Eggermont K, Terras FR, Thomma BP, De Samblanx GW, Buchala A, Métraux JP, Manners JM, Broekaert WF. Pathogen-induced systemic activation of a plant defensin gene in Arabidopsis follows a salicylic acid-independent pathway. Plant Cell 1996; 8:2309 - 23; PMID: 8989885
- Zhu W, Wei W, Fu Y, Cheng J, Xie J, Li G, Yi X, Kang Z, Dickman MB, Jiang DN. A secretory protein of necrotrophic fungus Sclerotinia sclerotiorum that suppresses host resistance. PLoS One 2013; 8:e53901; http://dx.doi.org/10.1371/journal.pone.0053901; PMID: 23342034
- Solano R, Stepanova A, Chao Q, Ecker JR. Nuclear events in ethylene signaling: a transcriptional cascade mediated by ETHYLENE-INSENSITIVE3 and ETHYLENE-RESPONSE-FACTOR1. Genes Dev 1998; 12:3703 - 14; http://dx.doi.org/10.1101/gad.12.23.3703; PMID: 9851977
- Schäfer P, Pfiffi S, Voll LM, Zajic D, Chandler PM, Waller F, Scholz U, Pons-Kühnemann J, Sonnewald S, Sonnewald U, et al. Manipulation of plant innate immunity and gibberellin as factor of compatibility in the mutualistic association of barley roots with Piriformospora indica.. Plant J 2009; 59:461 - 74; http://dx.doi.org/10.1111/j.1365-313X.2009.03887.x; PMID: 19392709
- Chan CW, Wohlbach DJ, Rodesch MJ, Sussman MR. Transcriptional changes in response to growth of Arabidopsis in high external calcium. FEBS Lett 2008; 582:967 - 76; http://dx.doi.org/10.1016/j.febslet.2008.02.043; PMID: 18307990
- Buchanan-Wollaston V, Page T, Harrison E, Breeze E, Lim PO, Nam HG, Lin JF, Wu SH, Swidzinski J, Ishizaki K, et al. Comparative transcriptome analysis reveals significant differences in gene expression and signalling pathways between developmental and dark/starvation-induced senescence in Arabidopsis.. Plant J 2005; 42:567 - 85; http://dx.doi.org/10.1111/j.1365-313X.2005.02399.x; PMID: 15860015
- Lee DJ, Park JY, Ku SJ, Ha YM, Kim S, Kim MD, Oh MH, Kim J. Genome-wide expression profiling of ARABIDOPSIS RESPONSE REGULATOR 7(ARR7) overexpression in cytokinin response. Mol Genet Genomics 2007; 277:115 - 37; http://dx.doi.org/10.1007/s00438-006-0177-x; PMID: 17061125
- Nishimura MT, Stein M, Hou BH, Vogel JP, Edwards H, Somerville SC. Loss of a callose synthase results in salicylic acid-dependent disease resistance. Science 2003; 301:969 - 72; http://dx.doi.org/10.1126/science.1086716; PMID: 12920300
- Berrocal-Lobo M, Molina A, Solano R. Constitutive expression of ETHYLENE-RESPONSE-FACTOR1 in Arabidopsis confers resistance to several necrotrophic fungi. Plant J 2002; 29:23 - 32; http://dx.doi.org/10.1046/j.1365-313x.2002.01191.x; PMID: 12060224
- Lorenzo O, Piqueras R, Sánchez-Serrano JJ, Solano R. ETHYLENE RESPONSE FACTOR1 integrates signals from ethylene and jasmonate pathways in plant defense. Plant Cell 2003; 15:165 - 78; http://dx.doi.org/10.1105/tpc.007468; PMID: 12509529
- Venus Y, Oelmüller R. Arabidopsis ROP1 and ROP6 influence germination time, root morphology, the formation of F-actin bundles, and symbiotic fungal interactions. Mol Plant 2013; 6:872 - 86; http://dx.doi.org/10.1093/mp/sss101; PMID: 23118477
- Wan D, Li R, Zou B, Zhang X, Cong J, Wang R, Xia Y, Li G. Calmodulin-binding protein CBP60g is a positive regulator of both disease resistance and drought tolerance in Arabidopsis.. Plant Cell Rep 2012; 31:1269 - 81; http://dx.doi.org/10.1007/s00299-012-1247-7; PMID: 22466450
- Wang L, Tsuda K, Truman W, Sato M, Nguyen V, Katagiri F, Glazebrook J. CBP60g and SARD1 play partially redundant critical roles in salicylic acid signaling. Plant J 2011; 67:1029 - 41; http://dx.doi.org/10.1111/j.1365-313X.2011.04655.x; PMID: 21615571
- Zhang Y, Xu S, Ding P, Wang D, Cheng YT, He J, Gao M, Xu F, Li Y, Zhu Z, et al. Control of salicylic acid synthesis and systemic acquired resistance by two members of a plant-specific family of transcription factors. Proc Natl Acad Sci U S A 2010; 107:18220 - 5; http://dx.doi.org/10.1073/pnas.1005225107; PMID: 20921422
- Liu F, Jiang H, Ye S, Chen WP, Liang W, Xu Y, Sun B, Sun J, Wang Q, Cohen JD, et al. The Arabidopsis P450 protein CYP82C2 modulates jasmonate-induced root growth inhibition, defense gene expression and indole glucosinolate biosynthesis. Cell Res 2010; 20:539 - 52; http://dx.doi.org/10.1038/cr.2010.36; PMID: 20354503
- van de Mortel JE, de Vos RC, Dekkers E, Pineda A, Guillod L, Bouwmeester K, van Loon JJ, Dicke M, Raaijmakers JM. Metabolic and transcriptomic changes induced in Arabidopsis by the rhizobacterium Pseudomonas fluorescens SS101. Plant Physiol 2012; 160:2173 - 88; http://dx.doi.org/10.1104/pp.112.207324; PMID: 23073694
- Hampton CR, Bowen HC, Broadley MR, Hammond JP, Mead A, Payne KA, Pritchard J, White PJ. Cesium toxicity in Arabidopsis.. Plant Physiol 2004; 136:3824 - 37; http://dx.doi.org/10.1104/pp.104.046672; PMID: 15489280
- Swindell WR. The association among gene expression responses to nine abiotic stress treatments in Arabidopsis thaliana.. Genetics 2006; 174:1811 - 24; http://dx.doi.org/10.1534/genetics.106.061374; PMID: 17028338
- Ascencio-Ibáñez JT, Sozzani R, Lee TJ, Chu TM, Wolfinger RD, Cella R, Hanley-Bowdoin L. Global analysis of Arabidopsis gene expression uncovers a complex array of changes impacting pathogen response and cell cycle during geminivirus infection. Plant Physiol 2008; 148:436 - 54; http://dx.doi.org/10.1104/pp.108.121038; PMID: 18650403
- Mohr PG, Cahill DM. Suppression by ABA of salicylic acid and lignin accumulation and the expression of multiple genes, in Arabidopsis infected with Pseudomonas syringae pv. tomato. Funct Integr Genomics 2007; 7:181 - 91; http://dx.doi.org/10.1007/s10142-006-0041-4; PMID: 17149585
- Ahlfors R, Brosché M, Kollist H, Kangasjärvi J. Nitric oxide modulates ozone-induced cell death, hormone biosynthesis and gene expression in Arabidopsis thaliana.. Plant J 2009; 58:1 - 12; http://dx.doi.org/10.1111/j.1365-313X.2008.03756.x; PMID: 19054359
- Clay NK, Adio AM, Denoux C, Jander G, Ausubel FM. Glucosinolate metabolites required for an Arabidopsis innate immune response. Science 2009; 323:95 - 101; http://dx.doi.org/10.1126/science.1164627; PMID: 19095898
- Kwon SJ, Jin HC, Lee S, Nam MH, Chung JH, Kwon SI, Ryu CM, Park OK. GDSL lipase-like 1 regulates systemic resistance associated with ethylene signaling in Arabidopsis.. Plant J 2009; 58:235 - 45; http://dx.doi.org/10.1111/j.1365-313X.2008.03772.x; PMID: 19077166
- Sattler SE, Mène-Saffrané L, Farmer EE, Krischke M, Mueller MJ, DellaPenna D. Nonenzymatic lipid peroxidation reprograms gene expression and activates defense markers in Arabidopsis tocopherol-deficient mutants. Plant Cell 2006; 18:3706 - 20; http://dx.doi.org/10.1105/tpc.106.044065; PMID: 17194769
- Guan Y, Nothnagel EA. Binding of arabinogalactan proteins by Yariv phenylglycoside triggers wound-like responses in Arabidopsis cell cultures. Plant Physiol 2004; 135:1346 - 66; http://dx.doi.org/10.1104/pp.104.039370; PMID: 15235117
- Armengaud P, Breitling R, Amtmann A. The potassium-dependent transcriptome of Arabidopsis reveals a prominent role of jasmonic acid in nutrient signaling. Plant Physiol 2004; 136:2556 - 76; http://dx.doi.org/10.1104/pp.104.046482; PMID: 15347784
- Thilmony R, Underwood W, He SY. Genome-wide transcriptional analysis of the Arabidopsis thaliana interaction with the plant pathogen Pseudomonas syringae pv. tomato DC3000 and the human pathogen Escherichia coli O157:H7. Plant J 2006; 46:34 - 53; http://dx.doi.org/10.1111/j.1365-313X.2006.02725.x; PMID: 16553894
- Wang MY, Liu XT, Chen Y, Xu XJ, Yu B, Zhang SQ, Li Q, He ZH. Arabidopsis acetyl-amido synthetase GH3.5 involvement in camalexin biosynthesis through conjugation of indole-3-carboxylic acid and cysteine and upregulation of camalexin biosynthesis genes. J Integr Plant Biol 2012; 54:471 - 85; http://dx.doi.org/10.1111/j.1744-7909.2012.01131.x; PMID: 22624950
- McManus EJ, Collins BJ, Ashby PR, Prescott AR, Murray-Tait V, Armit LJ, Arthur JS, Alessi DR. The in vivo role of PtdIns(3,4,5)P3 binding to PDK1 PH domain defined by knockin mutation. EMBO J 2004; 23:2071 - 82; http://dx.doi.org/10.1038/sj.emboj.7600218; PMID: 15116068
- Scott B, Takemoto D, Tanaka A. Fungal endophyte production of reactive oxygen species is critical for maintaining the mutualistic symbiotic interaction between Epichloe festucae and perennial ryegrass. Plant Signal Behav 2007; 2:171 - 3; http://dx.doi.org/10.4161/psb.2.3.3725; PMID: 19704747
- Schardl CL. Epichloë festucae and related mutualistic symbionts of grasses. Fungal Genet Biol 2001; 33:69 - 82; http://dx.doi.org/10.1006/fgbi.2001.1275; PMID: 11456460
- Schardl CL, Leuchtmann A, Spiering MJ. Symbioses of grasses with seedborne fungal endophytes. Annu Rev Plant Biol 2004; 55:315 - 40; http://dx.doi.org/10.1146/annurev.arplant.55.031903.141735; PMID: 15377223
- Murashige T, Skoog F. A revised medium for rapid growth and bio assays with tobacco tissue cultures. Physiol Plant 1962; 15:473 - 97; http://dx.doi.org/10.1111/j.1399-3054.1962.tb08052.x
- Verma S, Varma A. Piriformospora indica, gen. et sp. nov., a new root-colonizing fungus. Mycologia 1998; 90:896 - 903; http://dx.doi.org/10.2307/3761331
- Pham GH, Kumari R, Singh A, Sachdev M, Prasad R, Kaldorf M, Buscot F, Oelmüller R, Peškan T, Weiss M, et al. Axenic cultures of Piriformospora indica. In: Varma A, Abbott L, Werner D, Hampp R, eds. Plant Surface Microbiology. Springer-Verlag, 2004; 593-616.
- Johnson JM, Sherameti I, Ludwig A, Nongbri PL, Sun C, Lou B, Varma A, Oelmüller R. Protocols for Arabidopsis thaliana and Piriformospora indica co-cultivation – A model system to study plant beneficial traits. Endocyt. Cell Res 2011; 21:101 - 13 http://zs.thulb.uni-jena.de/receive/jportal_jparticle_00247947
- Vahabi K, Johnson JM, Drzewiecki C, Oelmüller R. Fungal staining tools to study the interaction between the beneficial endophyte Piriformospora indica with Arabidopsis thaliana roots. Endocytobiosis Cell Res 2011; 21:77 - 88
- Pfaffl MW. A new mathematical model for relative quantification in real-time RT-PCR. Nucleic Acids Res 2001; 29:e45; http://dx.doi.org/10.1093/nar/29.9.e45; PMID: 11328886
- Porra RJ, Thompson WA, Kriedemann PE. Determination of accurate extinction coefficients and simultaneous equations for assaying chlorophylls a and b extracted with four different solvents; verification of the concentration of chlorophyll standards by atomic absorption spectroscopy. Biochim Biophys Acta 1989; 975:384 - 94; http://dx.doi.org/10.1016/S0005-2728(89)80347-0