Abstract
Distinct amino acid metabolic pathways constitute integral parts of the plant immune system. We have recently identified pipecolic acid (Pip), a lysine-derived non-protein amino acid, as a critical regulator of systemic acquired resistance (SAR) and basal immunity to bacterial infection in Arabidopsis thaliana. In Arabidopsis, Pip acts as an endogenous mediator of defense amplification and priming. For instance, Pip conditions plants for effective biosynthesis of the phenolic defense signal salicylic acid (SA), accumulation of the phytoalexin camalexin, and expression of defense-related genes. Here, we show that tobacco plants respond to leaf infection by the compatible bacterial pathogen Pseudomonas syringae pv tabaci (Pstb) with a significant accumulation of several amino acids, including Lys, branched-chain, aromatic, and amide group amino acids. Moreover, Pstb strongly triggers, alongside the biosynthesis of SA and increases in the defensive alkaloid nicotine, the production of the Lys catabolites Pip and α-aminoadipic acid. Exogenous application of Pip to tobacco plants provides significant protection to infection by adapted Pstb or by non-adapted, hypersensitive cell death-inducing P. syringae pv maculicola. Pip thereby primes tobacco for rapid and strong accumulation of SA and nicotine following bacterial infection. Thus, our study indicates that the role of Pip as an amplifier of immune responses is conserved between members of the rosid and asterid groups of eudicot plants and suggests a broad practical applicability for Pip as a natural enhancer of plant disease resistance.
Introduction
The plant immune system is multi-layered and comprises constitutive and inducible defense strategies.Citation1 The signaling network underlying inducible plant immunity to microbial pathogens is regulated by hormone-like metabolites. The phenolic compound salicylic acid (SA) generally limits plant colonization by pathogens with a biotrophic or hemibiotrophic lifestyle.Citation2 By contrast, the oxylipin jasmonic acid (JA) and the gaseous phytohormone ethylene (ET) cooperatively act to induce defense responses that counteract invasion of plant tissue by necrotrophs.Citation3
Besides the classical SA- and JA/ET-defense pathways, plant immunity to microbial pathogens is regulated by distinct amino acid metabolic pathways.Citation4 Amino acids can serve as precursors for defensive antimicrobial compounds that are either constitutively produced during plant development or accumulate following pathogen inoculation.Citation5,Citation6 Recent findings indicate that the importance of amino acid-associated metabolic pathways for plant defense goes far beyond a precursor function for phytoanticipin or phytoalexin biosynthesis. For example, Asp oxidation and the subsequent formation of pyridine nucleotides represent an important metabolic route for both stomatal and post-invasion immunity of plants plants.Citation7-Citation9 In addition, the oxidation of Arg- and/or Orn-derived polyamines and the metabolism of Pro contribute to the production of reactive oxygen species and to the development of hypersensitive cell death following elicitor treatment or inoculation with avirulent bacteria.Citation10,Citation11 Further, the formation of acetylated amino acids and phytohormone-amino acid conjugates can mediate or negatively regulate plant defense signaling and pathogen resistance.Citation12-Citation14 Also, enhanced production of particular amino acids can directly impact plant resistance to specific pathogen types. For instance, analyses of Arabidopsis dmr1 with mutational defects in homoserine kinase and rsp1 that expresses a feedback-insensitive Asp kinase 2 isoform have indicated that both homoserine and Thr enhance resistance to infection by oomycete but not by fungal or bacterial pathogens pathogens.Citation15,Citation16 However, the mechanisms by which homoserine and Thr provide plant resistance to oomycete pathogens are not yet defined.
In Arabidopsis plants inoculated with the bacterial pathogen Pseudomonas syringae pv maculicola, the non-protein amino acid pipecolic acid (homoproline) accumulates in both locally-infected and in distal leaves.Citation17 Pipecolic acid (Pip) is a Lys-derived metabolite in plants and animals with important physiological functions.Citation4 It represents the major Lys catabolite in rat brain brain,Citation18 and has been recognized as a weak inhibitory neurotransmitter and GABA agonist in animals.Citation19 In Arabidopsis, Pip accumulation is critical for the establishment of systemic acquired resistance (SAR), an inducible immune response initiated in inoculated leaf tissue that confers broad-spectrum immunity to the whole plant foliage.Citation17,Citation20 Since it enriches in petiole exudates of pathogen-treated leaves in a specific manner, a function for Pip in SAR long-distance signaling is probable.Citation17 Importantly, Pip orchestrates SAR establishment via a feedback amplification mechanism. Moreover, it acts as an endogenous mediator of defense priming enabling SAR-induced Arabidopsis plants to more quickly and vigorously activate defense responses such as accumulation of the phytoalexin camalexin, SA biosynthesis, and expression of defense-related genes in the course of a subsequent microbial attack.Citation17 Besides its central role in SAR, Pip positively regulates SA biosynthesis at inoculation sites and significantly contributes to the establishment of basal and gene-for-gene resistance to bacterial pathogens in Arabidopsis. The exogenous application of Pip is sufficient to markedly heighten resistance of Arabidopsis to avirulent and virulent P. syringae and to promote plants into a primed state.Citation17
Results
We recently demonstrated that the inoculation of Arabidopsis leaves with the compatible bacterial pathogen P. syringae pv maculicola (Psm) results in massive changes in the leaf contents of free amino acids.Citation17 In particular, branched-chain amino acids (Val, Leu, Ile), aromatic amino acids (Phe, Tyr, Trp), and Lys markedly accumulated, whereas the levels of Asp, the precursor of Lys, Ile, and several other amino acids, decreased. Most strikingly, the Lys catabolites Pip and α-aminoadipic acid (Aad) that were only faintly detectable in non-inoculated plants accumulated to more than 50-fold after Psm inoculation.Citation17
To examine whether such massive pathogen-induced changes in the metabolism of amino acids do also occur in other plant species, we leaf-inoculated 4-week-old tobacco (Nicotiana tabacum cv Xanthi) plants with P. syringae pv tabaci 6605 (Pstb), a compatible bacterial strain that causes tobacco wildfire disease,Citation21 and determined amino acid levels in leaves 2 d post inoculation (dpi). Infiltration of 10 mM MgCl2 served as a mock-treatment on control plants. Compared with the mock-control plants, Pstb treated tobacco accumulated high levels of Lys, Pip, and Aad in inoculated leaves, indicating that both Lys ana- and catabolism were strongly activated upon Pstb inoculation (). Moreover, the levels of the aromatic, shikimate-derived amino acids Trp, Tyr, and Phe, as well as tyramine, the decarboxylation product of Tyr, significantly increased upon Pstb treatment. In addition, the branched chain amino acids Val, Leu, and Ile, as well as β-Ala and the amide group amino acids Asn and Gln increased to considerable amounts upon bacterial treatment. Moderate Pstb-induced increases were also detected for Ala, Thr, Orn, Pro, and Gly. By contrast, the levels of the 2 quantitatively most prominent amino acids, Asp and Glu, decreased upon Pstb inoculation (). Thus, tobacco leaves inoculated with Pstb undergo similar changes in amino acid metabolism than Psm-inoculated Arabidopsis leaves (; Návarová et al.Citation17).
Figure 1. Changes in the levels of free amino acids and amines in N. tabacum cv Xanthi leaves upon inoculation with compatible P. syringae pv tabaci (Pstb) 2 d post inoculation (dpi). Mean values of 3 to 5 replicate samples are given in µg g−1 fresh weight (FW) ± SD. Mock-treatments were performed by infiltration of leaves with a 10 mM MgCl2 solution. Asterisks denote statistically significant differences between Pstb- and MgCl2-samples (2-tailed t-test; ***: p < 0.001; **: p < 0.01; *: p < 0.05). Ratios of the values of the Pstb (P)- and the MgCl2 (M)-samples (P/M) are also given.
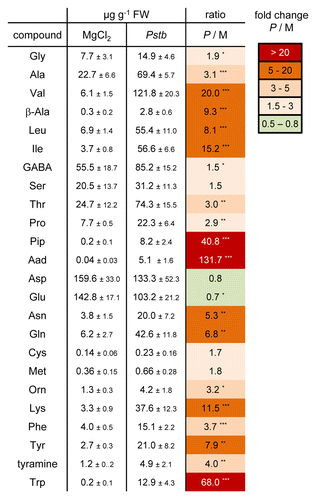
A time-course analyses revealed that the Lys catabolites Pip and Aad did not accumulate before 8 h post inoculation (hpi) with Pstb. However, the biosynthesis of both metabolites was induced between 8 hpi and 24 hpi (). From 24 hpi onwards, the levels of Pip and Aad in Pstb-treated plants were strongly increased compared with mock-control plants, reaching values of 12.7 and 5.5 µg per g fresh weight (FW) at 72 hpi, respectively (). By comparison, a significant albeit quantitatively moderate accumulation of SA could already be detected at 8 hpi, indicating that SA accumulation precedes Pip accumulation at bacterial inoculation sites (). From 24 hpi, SA was produced to higher amounts and reached levels of about 9 µg g−1 FW. The kinetic characteristics of Pip, Aad, and SA accumulation in Pstb-treated tobacco leaves are comparable to those in Psm-inoculated Arabidopsis leaves, as the present work and previous results from our laboratory indicate (, , and ; Mishina et al.;Citation22 Návarová et al.Citation17).
Figure 2. Time course of (A) pipecolic acid (Pip) and (B) α-aminoadipic acid (Aad) accumulation in tobacco leaves inoculated with compatible Pstb at indicated times after inoculation. Dark squares: Pstb-inoculated leaves; light diamonds: mock-treated leaves. Values represent the mean ± SD of 3 replicate samples. Asterisks denote statistically significant differences between Pstb- and mock-samples (***: p < 0.001; **: p < 0.01; 2-tailed t-test).
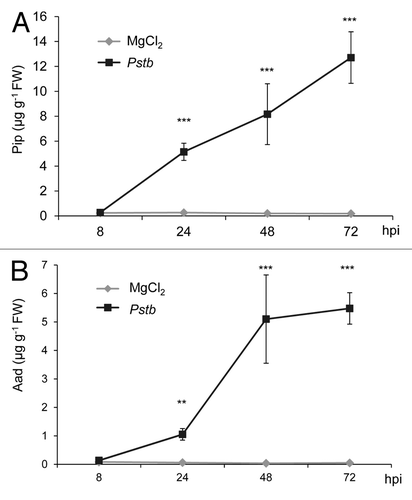
Figure 3. Time course of (A) salicylic acid (SA) accumulation and (B) nicotine production in tobacco leaves inoculated with compatible Pstb at indicated times after inoculation. Light diamonds: mock-treated leaves; dark squares: Pstb-inoculated leaves. Values represent the mean ± SD of 3 replicate samples. Asterisks denote statistically significant differences between Pstb- and mock-samples (***: p < 0.001; **: p < 0.01; *: p < 0.05; 2-tailed t-test).
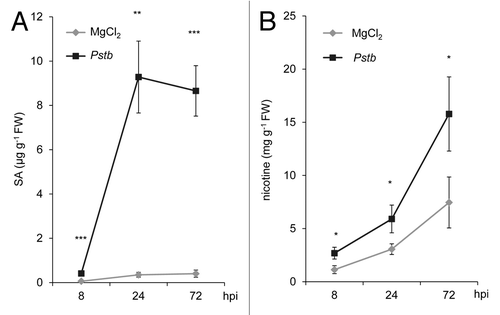
We also monitored the levels of nicotine, the most prominent pyridine alkaloid of N. tabacum,Citation23 in Pstb- and mock-treated tobacco leaves. The basal leaf levels of nicotine of the tobacco Xanthi cultivar amounted to about 1 mg g−1 FW, and the levels moderately rose both in pressure infiltrated mock-control and pathogen-treated plants over time (). Remarkably, the leaves of Pstb-treated plants contained significantly higher levels of nicotine than those of mock-control plants, albeit the relative induction of nicotine production by Pstb was lower than the induction of SA, Pip, or Aad biosynthesis (Figs. 2 and 3). Together, this data indicate that the levels of nicotine moderately rise in response to both a mechanical (mock infiltration) and a bacterial stimulus.
Exogenous application of Pip is sufficient to markedly enhance resistance to the compatible Psm and the incompatible, hypersensitive response (HR)-inducing Psm avrRpm1 strain in wild-type Arabidopsis plants, and fully complements the defects of the Pip-deficient ald1 mutant in basal and Rpm1-mediated resistance.Citation17 We therefore tested whether exogenous Pip would enhance resistance of tobacco to bacterial pathogens. When 10 µmol Pip was applied via the root system to individual tobacco plants, an uptake of the amino acid into the shoot occurred, and the leaf levels of Pip increased from faint basal values of control plants (< 0.1 µg g−1 FW) to about 7.5 µg g−1 FW 1 d after application (), which approximately corresponded to the levels of Pip in Pstb-inoculated leaves (). To compare basal disease resistance to bacteria between Pip- and H2O-watered control plants, we performed leaf inoculations with Pstb and determined bacterial multiplication at 5 dpi. Strikingly, pre-treatment of tobacco with Pip suppressed Pstb multiplication in leaves by about 1 order of magnitude (), and significantly reduced the severe necrotic disease symptoms observed in control plants after Pstb infection (). These results indicate that Pip strongly enhances basal resistance of tobacco to compatible Pstb. We also inoculated Psm into tobacco leaves, a P. syringae strain virulent to Arabidopsis but incapable of tobacco infection, inducing an HR in leaves.Citation24 Psm multiplied significantly but to a fundamentally lower extent than Pstb in tobacco leaves within 5 dpi, and this moderate growth capability of Psm was essentially absent when plants were pre-treated with Pip (). Thus, exogenous Pip enhances resistance of both tobacco and Arabidopsis plants to compatible and HR-inducing bacterial pathogens.
Figure 4. Exogenous pipecolic acid supplied to tobacco plants via the roots is transported to leaves and leads to a slight rise of leaf Aad levels. Leaf contents of (A) Pip and (B) Aad in leaves of tobacco plants 1 d after supplying 10 µmol Pip (10 ml of a 1 mM solution) or 10 ml of H2O via the roots. Light bars: H2O-treated plants; dark bars: Pip-treated plants. Values represent the mean ± SD of 3 replicate samples. Asterisks denote statistically significant differences between samples (***: p < 0.001; 2-tailed t-test).
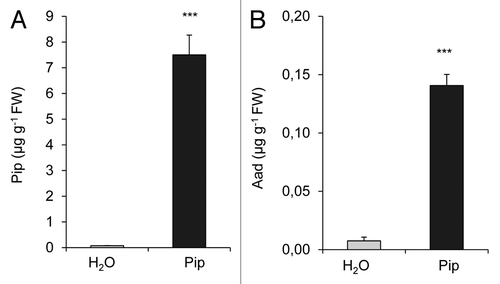
Figure 5. Exogenous Pip enhances disease resistance of tobacco plants to compatible Pstb and non-adapted, HR-inducing Psm. Plant pots were supplied with 10 ml of H2O or 10 ml of 1 mM (≡ 10 µmol) Pip 1 d prior to bacterial inoculation. (A) Bacterial numbers of Pstb (applied in titers of OD 0.001) in leaves at 0 dpi and 5 dpi. The y-axis is depicted in a logarithmic scale. Bars represent the mean ± SD of at least 7 replicate samples. Asterisks denote statistically significant differences between leaf samples of control- and Pip-treated plants (***: p < 0.001; 2-tailed t-test). (B) Representative disease symptoms of Pstb-infected tobacco leaves from H2O and Pip pre-treated plants. (C) Bacterial numbers of Psm (applied in titers of OD 0.005) in leaves at 0 dpi and 5 dpi.
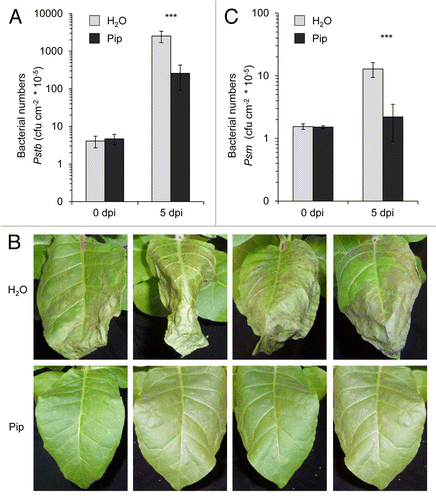
We next wondered by which means Pip would exert its resistance-enhancing activity in tobacco. In Arabidopsis, Pip amplifies defense signaling and primes plants for the early activation of defenses responses such as SA production, accumulation of the indolic phytoalexin camalexin, and expression of defense-related genes.Citation17 We therefore determined the amount of SA early after Pstb inoculation in leaves of control and Pip-pretreated tobacco plants. At 8 h post leaf infiltration, SA levels in mock-treated and Pstb-inoculated control plants amounted to 0.1 and 0.5 µg g−1 FW, respectively, indicating a modest but significant Pstb-induced increase of SA by a factor of about 5 in the absence of exogenous Pip (). The pre-treatment of plants with Pip had only a slight and statistically non-significant effect on SA levels in mock-infiltrated plants. By contrast, exogenous Pip strongly boosted the pathogen-induced biosynthesis of SA, since Pip-fed and afterwards Pstb-inoculated plants strongly accumulated SA to 4.8 µg g−1 FW already at 8 hpi (). Our metabolite analyses also indicated that the rather modest Pstb-induced increase of leaf nicotine levels at 8 hpi was strongly potentiated when plants had been pre-treated with Pip ( and ). Therefore, exogenous Pip is able to prime tobacco plants for a strong accumulation of SA and nicotine at early times after Pstb infection. Interestingly, in both tobacco and Arabidopsis, the priming response induced by Pip affects the biosynthesis of the defense hormone SA and of another defense-relevant plant natural product (nicotine in tobacco and camalexin in Arabidopsis).
Figure 6. Exogenous Pip primes tobacco plants for effective SA and nicotine production upon Pstb inoculation. H2O or 10 µmol Pip were applied to plants through the soil. Leaves were infiltrated 1 d later with Pstb (dark bars) or MgCl2 (light bars), and leaf metabolite levels were scored 8 h later. (A) SA contents in leaves. (B) Leaf nicotine levels. Bars represent the mean ± SD of 3 replicate samples. Different letters above the bars denote statistically significant differences between pairwise compared samples (p < 0.05, 2-tailed t-test).
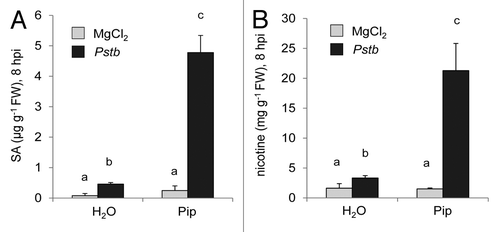
Discussion
L-Pip is a common Lys-derived non-protein amino acid of plants whose levels increase upon plant treatment with growth-affecting chemicals, in response to osmotic stress, and in the course of pathogen infections.Citation17,Citation25-Citation31 In Arabidopsis, the levels of Pip in leaves strongly increase after inoculation with avirulent or virulent P. syringae or following plant treatment with bacterial elicitors such as flagellin or lipopolysaccharide.Citation17 The pathogen-induced synthesis of Pip requires Arabidopsis AGD2-LIKE DEFENSE RESPONSE PROTEIN1 (ALD1),Citation17 an aminotransferase with substrate preference for Lys.Citation32 We have recently demonstrated that Pip is a regulatory metabolite indispensable for SAR in Arabidopsis.Citation4,Citation17 Pip can be mobilized from pathogen-inoculated leaves and orchestrates SAR establishment via a feedback amplification loop in leaves distant from the sites of inoculation. The accumulation of Pip throughout the plant, either via endogenous generation following pathogen inoculation or as a consequence of external application, is sufficient to render Arabidopsis into a state of enhanced disease resistance in which plants are primed for the activation of early defense responses.Citation17 Moreover, in inoculated Arabidopsis leaves, Pip promotes the induction of several defense responses, making the Lys catabolite required for the effective activation of basal and specific resistance to compatible and incompatible, HR-inducing bacterial pathogens.Citation17
In the current work, we show that this important role of Pip in defense amplification and inducible immunity is not restricted to Arabidopsis but also holds true for tobacco. In tobacco, like in Arabidopsis, Pip accumulates alongside with α-aminoadipic acid (Aad), another Lys catabolite, and its precursor Lys in leaves inoculated with compatible P. syringae (, ). Moreover, Pip pre-treatment via the soil, which results in physiological increases of Pip levels in the foliage (), strongly increases resistance of tobacco plants to infection by the compatible Pstb and the non-adapted, HR-inducing Psm strain (). Again, this outcome parallels our previous findings of resistance induction by Pip to virulent Psm and HR-inducing Psm avrRpm in Arabidopsis.Citation17 The Pip-triggered elevation of resistance to bacterial pathogens is associated with a marked potentiation of SA production in the first hours after pathogen contact in both tobacco and Arabidopsis (; Návarová et al.Citation17). SA is a plant defense hormone that induces immunity to plant pathogens with biotrophic and hemibiotrophic infection modes in Arabidopsis, tobacco, and other plants.Citation2,Citation33,Citation34 It is therefore likely that the enhanced production of SA early after pathogen contact contributes to the increased Pstb-resistance of Pip-treated tobacco plants (, ). Consistently, transgenic tobacco NahG plants that express a bacterial salicylate hydroxylase and are therefore not able to accumulate SA display lower resistance to Pstb than wild-type plants.Citation35 In the Arabidopsis SA biosynthesis mutant sid2/ics1, exogenous Pip elevates resistance to P. syringae significantly but not to the same extent than in the wild-type, indicating that both SA-independent and SA-dependent defense pathways contribute to Pip-mediated resistance.Citation17
In Arabidopsis, Pip also primes plants for the early expression of several defense-relevant genes as well as for the accumulation of another defense-related metabolite, the indolic phytoalexin camalexin.Citation17 Gene expression analyses suggest that the Pip-dependent conditioning of induced camalexin production in SAR-activated plants relies on partial pre-activation of the camalexin biosynthetic pathway.Citation36 Although camalexin exhibits antibacterial activity in vitro, the camalexin-deficient pad3 mutant is as resistant to P. syringae as the wild-type, suggesting that it does not have an obvious protective function against this pathogen in vivo.Citation37,Citation38 However, a potentiated production of camalexin might be relevant in interactions of Arabidopsis with fungal pathogens.Citation39,Citation40 In tobacco, the pyridine alkaloid nicotine is a prominent secondary metabolite with biological function in anti-herbivore defense.Citation23,Citation41 In our analyses, the levels of nicotine moderately rose upon mock-infiltration and Pstb infection (). Pressure infiltration into tobacco leaves unavoidably resulted in a slight wounding stress around the insertion points of the syringe. Because nicotine production is stimulated after wounding,Citation42 a wounding response after pressure infiltration might be the cause for the observed increase of nicotine contents upon infiltration of the mock-solution. However, since we also detected moderate but significant higher levels of nicotine in Pstb- compared with mock-treated leaves, bacterial inoculation also seems to stimulate nicotine production to a certain extent. Small pathogen-triggered increases of nicotine levels have been reported previously for P. syringae pv tomato-inoculated and tobacco mosaic virus-infected tobacco.Citation43,Citation44 By contrast, infection with the oomycete pathogen Phytophtora nicotianae resulted in decreased nicotine levels.Citation45 Whether a plant protective function of nicotine toward microbial pathogen infection exists is yet indeterminate to our knowledge, although nicotine has been demonstrated to have in vitro antimicrobial activity to several human-pathogenic fungi and bacteria.Citation46 Interestingly, our results show that, similar to camalexin accumulation in Arabidopsis,Citation17 the P. syringae-induced production of nicotine in tobacco is strongly potentiated by Pip (). This is remarkable because it indicates that Pip is able to prime distinct pathways of secondary metabolism in distantly related dicotyledonous plant species.
External feeding of tobacco plants with Pip via the roots led to strong increases of Pip levels in the foliage (~7.5 µg g−1 FW; ), indicating efficient root uptake and transport of the amino acid to leaves. Similar to previous feeding studies in Arabidopsis,Citation17 we also detected small but significant increases of Aad levels after Pip application (~0.14 µg g−1 FW; ). Therefore, it is likely that, similar to Arabidopsis, tobacco possesses an enzymatic pathway involved in the catabolism of Pip to Aad. Arabidopsis contains pipecolate oxidase (PIPOX) that catalyzes the conversion of Pip to δ1-piperideine-6-carboxylic acid, a precursor of Aad.Citation47 Direct catabolism of Pip to Aad might contribute to modulating the levels of Pip in different physiological situations. Microarray data show that the Arabidopsis PIPOX gene is downregulated in leaves upon P. syringae-inoculation (https://www.genevestigator.com/gv), consistent with attenuated conversion of Pip to Aad in defense situations when the accumulation of the Pip signal is necessary.Citation4 However, the pathogen-induced production of Aad predominatly occurs by immediate Lys catabolism via lysine ketoglutarate reductase/saccharopine dehydrogenase, and Aad per se has only minor influence on plant resistance to P. syringae.Citation17,Citation48
According to our previous findings in Arabidopsis, the probably most noticeable role for Pip in plant immunity represents its critical function in SAR.Citation17 In our experiments, however, tobacco plants did not or only slightly elevate their levels of SA or Pip in distant leaf tissue upon localized leaf inoculations with Pstb or Psm, and a reproducible SAR response could not be observed (data not shown). Consistently, Krzymowska et al.Citation24 reported that Psm-inoculated Nicotiana tabacum cv Xanthi failed to induce systemic defense responses, and they reasoned that an early collapse of inoculated leaf areas and concomitant rapid degeneration of vascular tissue might account for this failure. A similar scenario might be responsible for the lack of SAR in the present study because rapid necrotic tissue collapse was macroscopically observed at the sites of both Pstb- or Psm-inoculation from 2 dpi onwards. Therefore, to confirm the signaling function of Pip for SAR in this species, leaf infections with tobacco mosaic virus, a pathogen that typically induces a SAR response in tobacco plants,Citation49 might be performed.
Besides the activation of Lys ana-and catabolism, Pstb-infection results in significant increases of several other free, for the most part proteinogenic amino acids in tobacco leaves (). On the whole, these changes parallel the switch in amino acid composition occurring in Arabidopsis leaves following Psm inoculation.Citation17 Particularly, the levels of aromatic (Phe, Tyr, Trp) and aliphatic (Val, Leu, Ile, β-Ala, Ala) amino acids rise in P. syringae-infected leaf tissue of Arabidopsis and tobacco, whereas the levels of the 2 quantitatively predominant amino acids, Asp and Glu, remain constant or decline. This indicates that bacterial infection triggers a similar metabolic program in both plant species. In that regard, only a few species-specific differences are discernible. Pstb-infected tobacco leaves seem to accumulate amide group-containing amino acids (Asn and Gln) more prominently than Psm-infected Arabidopsis leaves. Moreover, whereas in Arabidopsis leaves, tyramine could not be detected by our relatively sensitive GC/MS-based analyses (limit of detection below 10 ng g−1 FW), the Tyr-derived biogenic amine was a prominent constituent (1.2 µg g−1 FW) of non-infected tobacco leaves, further increasing about 4-fold upon Pstb inoculation (; Návarová et al.Citation17). This reflects species-specific differences in amino acid catabolism. In tobacco and several other plant species but not in Arabidopsis, tyramine serves as the precursor for pathogen-inducible hydroxycinnamoyl-tyramine conjugates which have been associated with resistance to microbial attack.Citation50,Citation51
Pathogen-inducible metabolic changes leading to the accumulation of free amino acids have also been observed in P. syringae pv tomato DC3000 infected Arabidopsis or in cereals infected with rust fungi.Citation52,Citation53 What is the physiological role of this massive switch in amino acid-related metabolism in pathogen-inoculated plant tissue? The Lys-derived immune regulator Pip, the Tyr-derived hydroxycinnamoyl-tyramine conjugates, and the Trp-derived phytoalexin camalexin exemplify that at least a subset of amino acids accumulating after pathogen inoculation fulfill an active role in plant immunity, either directly in the regulation of defense responses (Pip) or as precursors for regulatory or antimicrobial substances.Citation4 The identification of Ile-derived isoleucic acid as a resistance-enhancing compoundCitation54 suggests that the observed significant elevations of branched chain amino acids might also contribute to plant immunity. However, besides participating in plant defense, free amino acids might also serve as nutrient sources for the invading pathogen,Citation55 and perturbations of a defined amino acid composition at infection sites can render compatible plant-microbe interactions incompatible.Citation15,Citation16
In conclusion, the present work demonstrates that pipecolic acid induces resistance to bacterial pathogens, and mediates defense amplification and priming responses in tobacco. We have shown that Pip potentiates the pathogen-induced accumulation of SA and nicotine in tobacco leaves, and we naturally cannot rule out that further, non-examined defense responses are also positively affected. Thus, Pip fulfills critical functions in inducible immunity of both ArabidopsisCitation17 and tobacco. The crucifer Arabidopsis and the nightshade family member tobacco belong to the Brassicales and Solanales, respectively. The Angiosperm Phylogeny Group III classification groups the Brassicales into rosids and the Solanales into the asterids, and thus into distinct clades of the core eudicots.Citation56 Moreover, pathogen-induced Pip accumulation has also been reported for rice, potato, and soybean.Citation27 This indicates that the occurrence and the immune regulatory role of Pip are widely conserved between Angiosperm plants. Our findings that exogenous application of naturally occurring Pip via the soil can move distinct plant species into a state of heightened resistance that primarily relies on plant conditioning and not on direct activation of defense responses furthermore suggests the usage of Pip as a natural resistance enhancer in the field, potentially applicable to a broad range of agriculturally relevant plants.
Materials and Methods
Plant growth
Tobacco (Nicotiana tabacum L. cv Xanthi) plants (seed stock NIC 380, IPK Gatersleben, Germany) were cultivated in individual pots containing a mixture of soil (Klasmann-Deilmann, Substrat BP3), vermiculite, and sand (4:1:1) in a controlled environmental chamber with a 16 h day (6:30 AM to 10:30 PM; photon flux density 100 µmol m−2 s−1) / 8 h night cycle and a relative humidity of 65%. Growth temperatures during the day and night period were 22 °C and 18 °C, respectively.
Cultivation of bacteria
Pseudomonas syringae pv tabaci strain 6605 (Pstb) and Pseudomonas syringae pv maculicola strain ES4326 (Psm) were grown in King’s B medium at 28 °C.Citation21,Citation57 For the cultivation of Psm, the KB medium was supplemented with 50 µg l−1 of rifampicin. Overnight log phase cultures were washed 4 times with 10 mM MgCl2 and diluted to different final optical densities (OD) at 600 nm for leaf inoculations.
Plant inoculation
Leaves of 4 of 4-week-old tobacco plants were inoculated with suspensions of Pstb or Psm in 10 mM MgCl2 as described previously.Citation57 The bacterial solutions were pressure-infiltrated from the abaxial side into a sample leaf using a 1 ml syringe without a needle. Mock-control treatments were performed by infiltrating a 10 mM MgCl2 solution.
Determination of bacterial growth
Multiplication of bacteria in tobacco leaves was assessed 5 d after inoculation with bacterial suspensions of OD = 0.001 (Pstb) or OD = 0.005 (Psm). To ensure the uniformity of the infiltration assays, initial bacterial numbers were quantified directly after inoculation (0 dpi). For 1 sample, discs originating from infiltrated areas of 3 different leaves were homogenized in 1 ml 10 mM MgCl2, appropriate dilutions of the homogenate were plated on King’s B medium and colony numbers were counted after incubating the plates at 28 °C for 2–3 d.
Exogenous application of pipecolic acid
One day prior to bacterial inoculation, the soil substrate of individually cultivated tobacco plants was supplemented with 10 ml of a 1 mM (10 µmol) D,L-pipecolic acid solution (S47167; Sigma-Aldrich). Control plants were supplemented with 10 ml of water.
Determination of amino acids
For metabolite analyses, leaves of tobacco plants were inoculated with Pstb (OD 0.005) or mock-infiltrated with 10 mM MgCl2, and leaf discs from infiltrated areas harvested at indicated times after treatment. For amino acid analyses, 4 leaf discs (corresponding to 80–100 mg FW) from different plants were combined and shock-frozen in liquid nitrogen. Leaf amino acid levels were determined by GC/MS using the EZ:faast free amino acid analysis kit (Phenomenex) for sample work-up and derivatization. The procedure is described in detail in Návarová et al.Citation17 Briefly, the frozen, ball-milled leaf material was extracted with 200 µl of 25% acetonitrile in 0.01 N HCl. 100 µl of the extract was analyzed following the protocol of the EZ:faast user’s manual (Phenomenex). The sample was finally dissolved in 50 µl of dichloromethane and subject to GC/MS analysis. Three µl of the sample mixture was separated on a silica capillary column (ZB-AAA 10 min x 0.25mm, Zebron, Phenomenex). The injector temperature was set to 250 °C, and a constant flow of helium (1.2 ml/min) and the following temperature program were used: 70 °C/3min with 8 °C/min to 240 °C, with 20 °C/min to 320 °C/2 min. For quantitative determination of individual amino acids, peaks originating from selected ion chromatograms were integrated: Gly (m/z 116), Ala (m/z 130), Val (m/z 158), β-Ala (m/z 116), Leu (m/z 172), Ile (m/z 172), GABA (m/z 130), Ser (m/z 146), Thr (m/z 101), Pro (m/z 156), Pip (m/z 170), Aad (m/z 244), Asp (m/z 216), Glu (m/z 84), Asn (m/z 69), Gln (m/z 84), Cys (m/z 248), Orn (m/z 156), Lys (m/z 170), Phe (m/z 148), Tyr (m/z 107), Trp (m/z 130), and tyramine (m/z 120). Norvaline (m/z 158) served as an internal standard. Experimentally determined correction factors for each substance were considered. Arg, His, and Met were not analyzable with this method.
Determination of SA and nicotine
Determination of the levels of free SA and nicotine was performed using vapor-phase extraction and subsequent GC/MS analysis as described by Mishina and ZeierCitation58 and Návarová et al.Citation17 Briefly, about 120 mg of frozen, ball-milled leaf tissue was extracted with 600 µl of H2O:1-propanol:HCl (1:2:0.005), and 100 ng D4-salicylic acid was added as an internal standard. The mixture was partitioned with 1 ml of methylene chloride and centrifuged at 14000 rpm for phase separation. The organic phase was removed, dried over Na2SO4, and 2 µl of 2 M trimethylsilyldiazomethane in hexane (Sigma-Aldrich) was added to convert carboxylic acids into their corresponding methyl esters. The sample was subjected to a vapor phase extraction procedure using a volatile collector trap packed with Porapak-Q absorbent (VCT-1/4X3-POR-Q; Analytical Research Systems) and an evaporation temperature of 200 °C. Samples were eluted from the collector trap with 1 ml of methylene chloride. The sample volume was reduced to 50 µl in a stream of nitrogen, and the sample was subjected to GC/MS analysis. Five µl of the sample was separated on a gas chromatograph (GC 7890A; Agilent Technologies) equipped with a fused silica capillary column (ZB-5MS 30 min x 0.25mm, Zebron, Phenomenex), and mass spectra were recorded with a combined 5975C mass spectrometric detector (Agilent Technologies) in the EI mode. The GC injector temperature was set to 250 °C. A constant flow of helium (1.2 ml/min) was applied, and the following temperature program was used: 50 °C/3min with 8 °C/min to 240 °C, with 20 °C/min to 320 °C/3 min. For quantitative determination of metabolites, peaks originating from selected ion chromatograms (m/z = 120 for SA, m/z = 124 for D4-SA, m/z = 162 for nicotine) were integrated. Experimentally determined correction factors for SA and nicotine were considered for the calculation of the metabolite levels.
Reproducibility of experiments and statistical analyses
The presented data resulted from single biological experiments. Results were repeated twice in biologically independent experiments with similar outcomes. Statistical analyses were performed using the Student t test.
Abbreviations: | ||
Aad | = | α-aminoadipic acid |
ALD1 | = | AGD2-LIKE DEFENSE RESPONSE PROTEIN1 |
dpi | = | days post inoculation |
ET | = | ethylene |
FW | = | fresh weight |
GABA | = | γ-aminobutyric acid |
hpi | = | hours post inoculation |
HR | = | hypersensitive response |
JA | = | jasmonic acid |
Pip | = | pipecolic acid |
PIPOX | = | pipecolate oxidase |
Psm | = | Pseudomonas syringae pv. maculicola |
Pstb | = | Pseudomonas syringae pv tabaci 6605 |
SA | = | salicylic acid |
SAR | = | systemic acquired resistance |
SD | = | standard deviation |
Acknowledgments
This work was supported by the German Research Foundation (DFG - Cluster of Excellence on Plant Sciences – CEPLAS). We thank Yuki Ichinose (Okayama University) for the donation of the P. syringae pv tabaci strain 6605.
Disclosure of Potential Conflicts of Interest
No potential conflicts of interest were disclosed.
References
- Spoel SH, Dong X. How do plants achieve immunity? Defence without specialized immune cells. Nat Rev Immunol 2012; 12:89 - 100; http://dx.doi.org/10.1038/nri3141; PMID: 22273771
- Wildermuth MC, Dewdney J, Wu G, Ausubel FM. Isochorismate synthase is required to synthesize salicylic acid for plant defence. Nature 2001; 414:562 - 5; http://dx.doi.org/10.1038/35107108; PMID: 11734859
- Memelink J. Regulation of gene expression by jasmonate hormones. Phytochemistry 2009; 70:1560 - 70; http://dx.doi.org/10.1016/j.phytochem.2009.09.004; PMID: 19796781
- Zeier J. New insights into the regulation of plant immunity by amino acid metabolic pathways. Plant Cell Environ 2013; http://dx.doi.org/10.1111/pce.12122; PMID: 23611692
- Bednarek P, Osbourn A. Plant-microbe interactions: chemical diversity in plant defense. Science 2009; 324:746 - 8; http://dx.doi.org/10.1126/science.1171661; PMID: 19423814
- Ahuja I, Kissen R, Bones AM. Phytoalexins in defense against pathogens. Trends Plant Sci 2012; 17:73 - 90; http://dx.doi.org/10.1016/j.tplants.2011.11.002; PMID: 22209038
- Macho AP, Boutrot F, Rathjen JP, Zipfel C. Aspartate oxidase plays an important role in Arabidopsis stomatal immunity. Plant Physiol 2012; 159:1845 - 56; http://dx.doi.org/10.1104/pp.112.199810; PMID: 22730426
- Zhang X, Mou Z. Extracellular pyridine nucleotides induce PR gene expression and disease resistance in Arabidopsis.. Plant J 2009; 57:302 - 12; http://dx.doi.org/10.1111/j.1365-313X.2008.03687.x; PMID: 18798871
- Pétriacq P, de Bont L, Hager J, Didierlaurent L, Mauve C, Guérard F, Noctor G, Pelletier S, Renou JP, Tcherkez G, et al. Inducible NAD overproduction in Arabidopsis alters metabolic pools and gene expression correlated with increased salicylate content and resistance to Pst-AvrRpm1.. Plant J 2012; 70:650 - 65; http://dx.doi.org/10.1111/j.1365-313X.2012.04920.x; PMID: 22268572
- Yoda H, Yamaguchi Y, Sano H. Induction of hypersensitive cell death by hydrogen peroxide produced through polyamine degradation in tobacco plants. Plant Physiol 2003; 132:1973 - 81; http://dx.doi.org/10.1104/pp.103.024737; PMID: 12913153
- Cecchini NM, Monteoliva MI, Alvarez ME. Proline dehydrogenase contributes to pathogen defense in Arabidopsis. Plant Physiol 2011; 155:1947 - 59; http://dx.doi.org/10.1104/pp.110.167163; PMID: 21311034
- Staswick PE, Tiryaki I. The oxylipin signal jasmonic acid is activated by an enzyme that conjugates it to isoleucine in Arabidopsis.. Plant Cell 2004; 16:2117 - 27; http://dx.doi.org/10.1105/tpc.104.023549; PMID: 15258265
- Adio AM, Casteel CL, De Vos M, Kim JH, Joshi V, Li B, Juéry C, Daron J, Kliebenstein DJ, Jander G. Biosynthesis and defensive function of Nδ-acetylornithine, a jasmonate-induced Arabidopsis metabolite. Plant Cell 2011; 23:3303 - 18; http://dx.doi.org/10.1105/tpc.111.088989; PMID: 21917546
- González-Lamothe R, El Oirdi M, Brisson N, Bouarab K. The conjugated auxin indole-3-acetic acid-aspartic acid promotes plant disease development. Plant Cell 2012; 24:762 - 77; http://dx.doi.org/10.1105/tpc.111.095190; PMID: 22374398
- van Damme M, Zeilmaker T, Elberse J, Andel A, de Sain-van der Velden M, van den Ackerveken G. Downy mildew resistance in Arabidopsis by mutation of HOMOSERINE KINASE.. Plant Cell 2009; 21:2179 - 89; http://dx.doi.org/10.1105/tpc.109.066811; PMID: 19622802
- Stuttmann J, Hubberten HM, Rietz S, Kaur J, Muskett P, Guerois R, Bednarek P, Hoefgen R, Parker JE. Perturbation of Arabidopsis amino acid metabolism causes incompatibility with the adapted biotrophic pathogen Hyaloperonospora arabidopsidis.. Plant Cell 2011; 23:2788 - 803; http://dx.doi.org/10.1105/tpc.111.087684; PMID: 21784950
- Návarová H, Bernsdorff F, Döring A-C, Zeier J. Pipecolic acid, an endogenous mediator of defense amplification and priming, is a critical regulator of inducible plant immunity. Plant Cell 2012; 24:5123 - 41; http://dx.doi.org/10.1105/tpc.112.103564; PMID: 23221596
- Chang YF. Pipecolic acid pathway: the major lysine metabolic route in the rat brain. Biochem Biophys Res Commun 1976; 69:174 - 80; http://dx.doi.org/10.1016/S0006-291X(76)80288-4; PMID: 1259753
- Charles AK. Pipecolic acid receptors in rat cerebral cortex. Neurochem Res 1986; 11:521 - 5; http://dx.doi.org/10.1007/BF00965321; PMID: 3014361
- Shah J, Zeier J. Long-distance communication and signal amplification in systemic acquired resistance. Front Plant Sci 2013; 4:30; http://dx.doi.org/10.3389/fpls.2013.00030; PMID: 23440336
- Taguchi F, Shimizu R, Nakajima R, Toyoda K, Shiraishi T, Ichinose Y. Differential effects of flagellins from Pseudomonas syringae pv. tabaci, tomato and glycinea on plant defense response. Plant Physiol Biochem 2003; 41:165 - 74; http://dx.doi.org/10.1016/S0981-9428(02)00018-9
- Mishina TE, Griebel T, Geuecke M, Attaran E, Zeier J. New insights into the molecular events underlying systemic acquired resistance. In: Lorito M, Woo SL, Scala F, eds. Biology of Plant-Microbe Interactions. Vol 6. St. Paul, MN: International Society for Molecular Plant-Microbe Interactions, 2008.
- Cai B, Jack AM, Lewis RS, Dewey RE, Bush LP. (R)-nicotine biosynthesis, metabolism and translocation in tobacco as determined by nicotine demethylase mutants. Phytochemistry 2013; In press http://dx.doi.org/10.1016/j.phytochem.2013.06.012; PMID: 23849545
- Krzymowska M, Konopka-Postupolska D, Sobczak M, Macioszek V, Ellis BE, Hennig J. Infection of tobacco with different Pseudomonas syringae pathovars leads to distinct morphotypes of programmed cell death. Plant J 2007; 50:253 - 64; http://dx.doi.org/10.1111/j.1365-313X.2007.03046.x; PMID: 17355437
- Morrison RI. The isolation of L-pipecolinic acid from Trifolium repens.. Biochem J 1953; 53:474 - 8; PMID: 13032096
- Zacharius RM, Thompson JF, Steward FC. The detection, isolation and identification of L(-)-pipecolic acid in the non-protein fraction of beans (Phaseolus vulgaris). J Am Chem Soc 1954; 76:2908 - 12; http://dx.doi.org/10.1021/ja01640a015
- Pálfi G, Dézsi L. Pipecolic acid as an indicator of abnormal protein metabolism in diseased plants. Plant Soil 1968; 29:285 - 91; http://dx.doi.org/10.1007/BF01348946
- Gupta RN, Spenser ID. Biosynthesis of the piperidine nucleus. The mode of incorporation of lysine into pipecolic acid and into piperidine alkaloids. J Biol Chem 1969; 244:88 - 94; PMID: 5813006
- Fujioka S, Sakurai A. Conversion of lysine to L-pipecolic acid induces flowering in Lemna paucicostata 151. Plant Cell Physiol 1997; 38:1278 - 80; http://dx.doi.org/10.1093/oxfordjournals.pcp.a029116
- Yatsu L, Boynton D. Pipecolic acid in leaves of strawberry plant as influenced by treatments affecting growth. Science 1959; 130:864 - 5; http://dx.doi.org/10.1126/science.130.3379.864; PMID: 17777486
- Moulin M, Deleu C, Larher F, Bouchereau A. The lysine-ketoglutarate reductase-saccharopine dehydrogenase is involved in the osmo-induced synthesis of pipecolic acid in rapeseed leaf tissues. Plant Physiol Biochem 2006; 44:474 - 82; http://dx.doi.org/10.1016/j.plaphy.2006.08.005; PMID: 17023168
- Song JT, Lu H, Greenberg JT. Divergent roles in Arabidopsis thaliana development and defense of two homologous genes, aberrant growth and death2 and AGD2-LIKE DEFENSE RESPONSE PROTEIN1, encoding novel aminotransferases. Plant Cell 2004; 16:353 - 66; http://dx.doi.org/10.1105/tpc.019372; PMID: 14729919
- Friedrich L, Vernooij B, Gaffney T, Morse A, Ryals J. Characterization of tobacco plants expressing a bacterial salicylate hydroxylase gene. Plant Mol Biol 1995; 29:959 - 68; http://dx.doi.org/10.1007/BF00014969; PMID: 8555459
- Achuo EA, Audenaert K, Meziane H, Höfte M. The salicylic acid-dependent defence pathway is effective against different pathogens in tomato and tobacco. Plant Pathol 2004; 53:65 - 72; http://dx.doi.org/10.1111/j.1365-3059.2004.00947.x
- Chun HJ, Park HC, Koo SC, Lee JH, Park CY, Choi MS, Kang CH, Baek D, Cheong YH, Yun DJ, et al. Constitutive expression of mammalian nitric oxide synthase in tobacco plants triggers disease resistance to pathogens. Mol Cells 2012; 34:463 - 71; http://dx.doi.org/10.1007/s10059-012-0213-0; PMID: 23124383
- Gruner K, Griebel T, Návarová H, Attaran E, Zeier J. Reprogramming of plants during systemic acquired resistance. Front Plant Sci 2013; 4:252; http://dx.doi.org/10.3389/fpls.2013.00252; PMID: 23874348
- Tsuji J, Jackson EP, Gage DA, Hammerschmidt R, Somerville SC. Phytoalexin Accumulation in Arabidopsis thaliana during the Hypersensitive Reaction to Pseudomonas syringae pv syringae.. Plant Physiol 1992; 98:1304 - 9; http://dx.doi.org/10.1104/pp.98.4.1304; PMID: 16668792
- Glazebrook J, Ausubel FM. Isolation of phytoalexin-deficient mutants of Arabidopsis thaliana and characterization of their interactions with bacterial pathogens. Proc Natl Acad Sci U S A 1994; 91:8955 - 9; http://dx.doi.org/10.1073/pnas.91.19.8955; PMID: 8090752
- Thomma BP, Nelissen I, Eggermont K, Broekaert WF. Deficiency in phytoalexin production causes enhanced susceptibility of Arabidopsis thaliana to the fungus Alternaria brassicicola.. Plant J 1999; 19:163 - 71; http://dx.doi.org/10.1046/j.1365-313X.1999.00513.x; PMID: 10476063
- Schlaeppi K, Abou-Mansour E, Buchala A, Mauch F. Disease resistance of Arabidopsis to Phytophthora brassicae is established by the sequential action of indole glucosinolates and camalexin. Plant J 2010; 62:840 - 51; http://dx.doi.org/10.1111/j.1365-313X.2010.04197.x; PMID: 20230487
- Steppuhn A, Gase K, Krock B, Halitschke R, Baldwin IT. Nicotine’s defensive function in nature. PLoS Biol 2004; 2:e217; http://dx.doi.org/10.1371/journal.pbio.0020217; PMID: 15314646
- Preston CA, Lewandowski C, Enyedi AJ, Baldwin IT. Tobacco mosaic virus inoculation inhibits wound-induced jasmonic acid-mediated responses within but not between plants. Planta 1999; 209:87 - 95; http://dx.doi.org/10.1007/s004250050609; PMID: 10467034
- Zhang L, Oh Y, Li H, Baldwin IT, Galis I. Alternative oxidase in resistance to biotic stresses: Nicotiana attenuata AOX contributes to resistance to a pathogen and a piercing-sucking insect but not Manduca sexta larvae. Plant Physiol 2012; 160:1453 - 67; http://dx.doi.org/10.1104/pp.112.200865; PMID: 22961128
- Ziebell H, Murphy AM, Groen SC, Tungadi T, Westwood JH, Lewsey MG, Moulin M, Kleczkowski A, Smith AG, Stevens M, et al. Cucumber mosaic virus and its 2b RNA silencing suppressor modify plant-aphid interactions in tobacco. Sci Rep 2011; 1:187; http://dx.doi.org/10.1038/srep00187; PMID: 22355702
- Ibáñez AJ, Scharte J, Bones P, Pirkl A, Meldau S, Baldwin IT, Hillenkamp F, Weis E, Dreisewerd K. Rapid metabolic profiling of Nicotiana tabacum defence responses against Phytophthora nicotianae using direct infrared laser desorption ionization mass spectrometry and principal component analysis. Plant Methods 2010; 6:14; http://dx.doi.org/10.1186/1746-4811-6-14; PMID: 20534155
- Pavia CS, Pierre A, Nowakowski J. Antimicrobial activity of nicotine against a spectrum of bacterial and fungal pathogens. J Med Microbiol 2000; 49:675 - 6; PMID: 10882095
- Goyer A, Johnson TL, Olsen LJ, Collakova E, Shachar-Hill Y, Rhodes D, Hanson AD. Characterization and metabolic function of a peroxisomal sarcosine and pipecolate oxidase from Arabidopsis.. J Biol Chem 2004; 279:16947 - 53; http://dx.doi.org/10.1074/jbc.M400071200; PMID: 14766747
- Galili G, Tang G, Zhu X, Gakiere B. Lysine catabolism: a stress and development super-regulated metabolic pathway. Curr Opin Plant Biol 2001; 4:261 - 6; http://dx.doi.org/10.1016/S1369-5266(00)00170-9; PMID: 11312138
- Ward ER, Uknes SJ, Williams SC, Dincher SS, Wiederhold DL, Alexander DC, Ahl-Goy P, Metraux JP, Ryals JA. Coordinate gene activity in response to agents that induce systemic acquired resistance. Plant Cell 1991; 3:1085 - 94; PMID: 12324583
- Negrel J, Jeandet P. Metabolism of tyramine and feruloyltyramine in TMV inoculated leaves of Nicotiana tabacum.. Phytochemistry 1987; 26:2185 - 90; http://dx.doi.org/10.1016/S0031-9422(00)84681-6
- Newman MA, von Roepenack-Lahaye E, Parr A, Daniels MJ, Dow JM. Induction of hydroxycinnamoyl-tyramine conjugates in pepper by Xanthomonas campestris, a plant defense response activated by hrp gene-dependent and hrp gene-independent mechanisms. Mol Plant Microbe Interact 2001; 14:785 - 92; http://dx.doi.org/10.1094/MPMI.2001.14.6.785; PMID: 11386374
- Ward JL, Forcat S, Beckmann M, Bennett M, Miller SJ, Baker JM, Hawkins ND, Vermeer CP, Lu C, Lin W, et al. The metabolic transition during disease following infection of Arabidopsis thaliana by Pseudomonas syringae pv. tomato.. Plant J 2010; 63:443 - 57; http://dx.doi.org/10.1111/j.1365-313X.2010.04254.x; PMID: 20497374
- Bushnell WR. Structural and physiological alterations in susceptible host tissue. Chapter 15. In: Bushnell WR, Roelfs AP, eds. The cereal rusts. Vol 1. Orlando, FL: Academic Press, 1984.
- von Saint Paul V, Zhang W, Kanawati B, Geist B, Faus-Kessler T, Schmitt-Kopplin P, Schäffner AR. The Arabidopsis glucosyltransferase UGT76B1 conjugates isoleucic acid and modulates plant defense and senescence. Plant Cell 2011; 23:4124 - 45; http://dx.doi.org/10.1105/tpc.111.088443; PMID: 22080599
- Hahn M, Mendgen K. Characterization of in planta-induced rust genes isolated from a haustorium-specific cDNA library. Mol Plant Microbe Interact 1997; 10:427 - 37; http://dx.doi.org/10.1094/MPMI.1997.10.4.427; PMID: 9150592
- Bremer B, Bremer K, Chase MW, Fay MF, Reveal JL, Soltis DE, Soltis PS, Stevens PF. An update of the Angiosperm Phylogeny Group classification for the orders and families of flowering plants: APG III. Bot J Linn Soc 2009; 161:105 - 21; http://dx.doi.org/10.1111/j.1095-8339.2009.00996.x
- Zeier J, Pink B, Mueller MJ, Berger S. Light conditions influence specific defence responses in incompatible plant-pathogen interactions: uncoupling systemic resistance from salicylic acid and PR-1 accumulation. Planta 2004; 219:673 - 83; http://dx.doi.org/10.1007/s00425-004-1272-z; PMID: 15098125
- Mishina TE, Zeier J. The Arabidopsis flavin-dependent monooxygenase FMO1 is an essential component of biologically induced systemic acquired resistance. Plant Physiol 2006; 141:1666 - 75; http://dx.doi.org/10.1104/pp.106.081257; PMID: 16778014