Abstract
The important role of the cytoskeletal scaffold is increasingly recognized in host-pathogen interactions. The cytoskeleton potentially functions as a weapon for both the plants defending themselves against fungal or oomycete parasites, and for the pathogens trying to overcome the resisting barrier of the plants. This concept, however, had not been investigated in marine algae so far. We are opening this scientific chapter with our study on the functional implications of the cytoskeleton in 3 filamentous brown algal species infected by the marine oomycete Eurychasma dicksonii. Our observations suggest that the cytoskeleton is involved in host defense responses and in fundamental developmental stages of E. dicksonii in its algal host.
Oomycetes are important plant and animal pathogens and are the cause of significant crop losses every year. Hence, a plethora of studies with different cultivated and model plant species investigate the diversity of parasite infection pathways and host defense responses.Citation1 However, little information is available on the interactions between algae and marine oomycetes, despite the epidemic outbreaks reportedCitation2 and the huge impact on intensive algal aquaculture.Citation3
Eurychasma dicksonii is a biotrophic, intracellular marine oomycete, capable to infect at least 45 species of brown seaweeds in laboratory cultures.Citation4 Molecular data reveal that E. dicksonii has a basal phylogenetic position in the oomycete lineage.Citation5,Citation6 The basic stages of the infection are known: the attachment of the parasite spore to the host cell wall, the penetration of its cytoplasm into the host cell, the formation of a multinucleated, unwalled thallus, and zoosporogenesis.Citation6 Hitherto, though, there was no knowledge about the role of cytoskeleton in the context of infection, which stimulated our research.
In land plants, reorganization of the cytoskeleton is part of the reaction to infection by fungal pathogens. The rearrangement of the cytoplasm and the relocation of the nuclei and other organelles are accompanied by rapid rearrangements of the cytoskeletal elements.Citation7 The plant cytoskeleton shows an extreme plasticity in order to serve the intracellular realignment.
At the same time, this indicates that the plant cytoskeleton could be the parasite’s target by producing anti-cytoskeletal compounds in an effort to overcome plant resistance, a mechanism known in several fungal and oomycete pathogens of higher plants.Citation8,Citation9
Consequently, the changes in microtubule (MT) organization are associated with both the plant defense and/or susceptibility toward oomycetes, respectively.Citation10 Therefore, our research on the organization and role of cytoskeleton in the host and the parasite sheds some light into the enormous variability in the specificity of the recognition, defense, and infection mechanisms.
Organization of the Host MTs
Our experiments of tubulin immunolocalization in the 3 algal species Ectocarpus siliculosus, Ectocarpus crouaniorum, and Pylaiella littoralis unveiled the MT dynamics in reaction to the intracellular infection by E. dicksonii. The host retains a normal organization of the MT bundles by the centrosome for most of the infection cycle until the start of the parasite zoosporogenesis. This is a particularly surprising result in comparison with most studies led on compatible plant-pathogen interactions. Usually, the host MTs lose their central organization by the centrosome and gradually depolymerize.Citation7,Citation11 Despite the delayed disorganization of the MTs, the brown algal cells investigated here ultimately allowed the completion of E. dicksonii intracellular development. There is, however, stark variability in the severity of symptoms acrossCitation4 and withinCitation12 the 45+ species of brown seaweeds infected by E. dicksonii. It would be enlightening to expand our study to this broader spectrum of interactions, in order to shed light into the possibly long-standing co-evolutionary relationship between E. dicksonii and its brown algal hosts.Citation1
Organization of the Parasite MTs
The arrangement of the MTs at each stage of infection underpins both the colonization of the host cell by the endoparasitic pathogen and the morphogenesis of the zoospores.Citation13,Citation14 In our recent publication, we analyze the typical cytoskeleton of a E. dicksonii spore during the different stages of infection.Citation15 It is clear that the duplication of the MTOC of each spore nucleus precedes the repeated and synchronous divisions. In parallel, we hypothesize that the parasite MTs participate actively in the compartmentalization of the E. dicksonii spores and the formation of the zoosporangium. A similar process was observed during sporangial cleavage in Saprolegnia, where the expansion of a central vacuole is combined with the role of MTs as a physical barrier.Citation16,Citation17
The synoptic table below () represents the fundamental modifications on the arrangement of the parasite MTs over the entire infection process.
Figure 2. Diagrammatic representation of the E. dicksonii infection stages, showing modifications of MT. Organization in both host and parasite. Blue filled circles: nuclei. Green lines: MT bundles. Green dots: MTOCs. Brown rectangle: infected algal cell. Orange: E. dicksonii plasmalemma and cell wall structures.
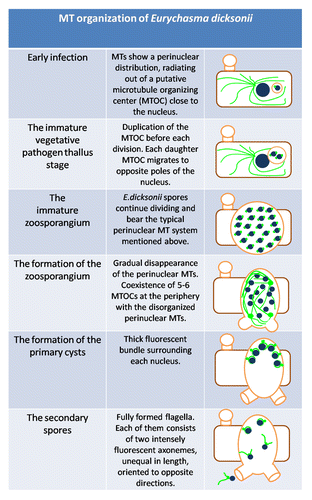
Organization of Actin Filaments (AFs)
Staining with rhodamine-phalloidin (Rh-Ph) revealed the actin organization of E. dicksonii.Citation15 In E. dicksonii spores, actin is organized mainly in the form of prominent plaques (). At the stage of zoosporogenesis, the actin distribution is modified. shows a typical image, where diffuse AFs are accompanied by a reduced number of actin dots. The potential contribution of the cytoskeletal elements in the positioning of the cleavage planes has already been discussed in the case of Phytophthora cinnamomi,Citation18 while in Phytophthora infestans the role of actin in hyphal growth has been examined.Citation19 In combination with the MT modifications during the formation of the mature zoosporangium and the encystment of the primary cysts as explained above, we assume that the E. dicksonii cytoskeleton contributes to this fundamental procedure.
Figure 1. Actin labeling of E. dicksonii infecting Pylaiella littoralis. (A) Prominent plaques of actin in a pathogen spore attached to the host cell wall. Arrow shows fine AFs accumulated in adhesorium-pad site. Inset: combination of DIC and DNA staining with Hoechst 33258 of the same spore. (B). AFs crossing the parasite cytoplasm in an early zoosporangium. Inset: DIC of the related image. (C) DIC of E. dicksonii spore attached to the surface of Macrocystispyrifera. Arrow shows the cell wall modifications. (D) Staining with Aniline Blue of E. dicksonii infecting Ectocarpus crouaniorum. Arrows show the attached spore and the accumulation of β-1,3glucans on the cell wall at the penetration site. The scale bar is 5μm.
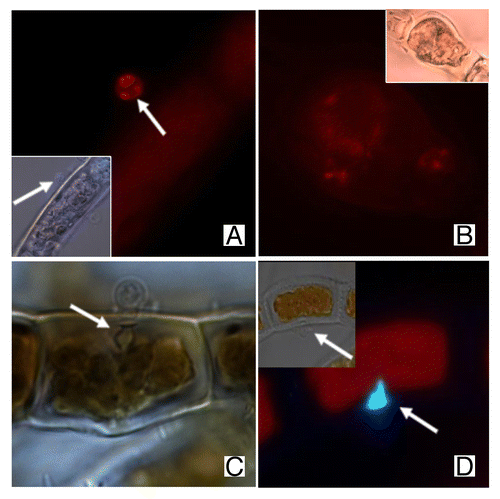
In some recent preliminary experiments, we applied Aniline Blue to stain β-1,3glucans such as callose. Interesting cell wall modifications are apparent at the penetration site under the light microscope (). Thus, parallel to the cytoskeleton organization, the algal cell wall shows a distinct thickening just beneath the empty pathogen spore () similar to the cell wall papillae reported in plants.Citation20 Based on recent results by Hardham et al. (2008),Citation21 we infer that the cytoskeleton plays a protagonistic role and facilitates the accumulation of cell wall material in the wounded point.
The results from the experiments performed so far encourage further study of the infection and defense mechanisms of the brown algae infected by the basal oomycete E. dicksonii. Could we ultimately crack the mysteries of algal immune systems?
Disclosure of Potential Conflicts of Interest
No potential conflicts of interest were disclosed.
References
- Lebeda A, Pink DAC, Astley A. Aspects of the interactions between wild Lactuca spp. and related genera and lettuce downy mildew (Bremialactucae). In: Spencer-Phillips PTN, Gisi U, Lebeda A, eds. Advances in Downy Mildew Research. Dordrecht: Kluwer, 2002:85-117.
- Küpper FC, Müller DG. Massive occurrence of the heterokont and fungal parasites Anisolpidium, Eurychasma and Chytridium in Pylaiella littoralis (Ectocarpales, Phaeophyceae). Nova Hedwigia 1999; 69:381 - 9
- Gachon CMM, Sime-Ngando T, Strittmatter M, Chambouvet A, Kim GH. Algal diseases: spotlight on a black box. Trends Plant Sci 2010; 15:633 - 40; http://dx.doi.org/10.1016/j.tplants.2010.08.005; PMID: 20833575
- Müller DG, Küpper FC, Küpper H. Infection experiments reveal broad host ranges of Eurychasma dicksonii (Oomycota) and Chytridium polysiphoniae (Chytridiomycota), two eukaryotic parasites in marine brown algae (Phaeophyceae). Phycological Res 1999; 47:217 - 23; http://dx.doi.org/10.1111/j.1440-1835.1999.tb00301.x
- Küpper FC, Maier I, Müller DG, Loiseaux-De Goer S, Guillou L. Phylogenetic affinities of two eukaryotic pathogens of marine macroalgae, Eurychasma dicksonii (Wright) Magnus and Chytridium polysiphoniae Cohn. Cryptogam, Algol 2006; 27:165 - 84
- Sekimoto S, Beakes GW, Gachon CMM, Müller DG, Küpper FC, Honda D. The development, ultrastructural cytology, and molecular phylogeny of the basal oomycete Eurychasma dicksonii, infecting the filamentous phaeophyte algae Ectocarpus siliculosus and Pylaiella littoralis. Protist 2008; 159:299 - 318; http://dx.doi.org/10.1016/j.protis.2007.11.004; PMID: 18243049
- Takemoto D, Jones DA, Hardham AR. GFP-tagging of cell components reveals the dynamics of subcellular re-organization in response to infection of Arabidopsis by oomycete pathogens. Plant J 2003; 33:775 - 92; http://dx.doi.org/10.1046/j.1365-313X.2003.01673.x; PMID: 12609049
- Hardham AR, Jones DA, Takemoto D. Cytoskeleton and cell wall function in penetration resistance. Curr Opin Plant Biol 2007; 10:342 - 8; http://dx.doi.org/10.1016/j.pbi.2007.05.001; PMID: 17627866
- Kobayashi I, Kobayashi Y. Microtubules and pathogen defense. In: Nick P, ed. Plant cell monographs: plant microtubules. Berlin: Springer-Verlag, 2008:121-140.
- Hardham AR. Microtubules and biotic interactions. Plant J 2013; 75:278 - 89; http://dx.doi.org/10.1111/tpj.12171; PMID: 23480445
- Cahill D, Rookes J, Michalczyk A, McDonald K, Drake A. Microtubule dynamics in compatible and incompatible interactions of soybean hypocotyl cells with Phytophthora sojae.. Plant Pathol 2002; 51:629 - 40; http://dx.doi.org/10.1046/j.0032-0862.2002.00758.x
- Gachon CMM, Strittmatter M, Müller DG, Kleinteich J, Küpper FC. Detection of differential host susceptibility to the marine oomycete pathogen Eurychasma dicksonii by real-time PCR: not all algae are equal. Appl Environ Microbiol 2009; 75:322 - 8; http://dx.doi.org/10.1128/AEM.01885-08; PMID: 19011072
- Holloway SA, Heath IB. Morphogenesis and the role of microtubules in synchronous populations of Saprolegnia zoospores. Exp Mycol 1977; 1:9 - 29; http://dx.doi.org/10.1016/S0147-5975(77)80027-3
- Hardham AR. The Asexual Life Cycle. In: Lamour K, Kamoun S, eds. Oomycete Genetics and Genomics: Diversity, Interactions and Research Tools. Oxford: Wiley-Blackwell, 2009:93-119.
- Tsirigoti A, Küpper FC, Gachon CMM, Katsaros C. Cytoskeleton organisation during the infection of three brown algal species, Ectocarpus siliculosus, Ectocarpus crouaniorum and Pylaiella littoralis, by the intracellular marine oomycete Eurychasma dicksonii.. Plant Biol (Stuttg) 2013; http://dx.doi.org/10.1111/plb.12041; PMID: 23692049
- Gay JL, Greenwood AD. Structural aspects of zoospore production in Saprolegnia ferax with particular reference to the cell and vacuolar membranes. In: Madelin MF, ed. The Fungus Spore. London, UK: Butterworths, 1966:95-110.
- Heath IB, Greenwood AD. Ultrastructural observations on the kinetosomes, and golgi bodies during the asexual life cycle of Saprolegnia.. Z Zellforsch Mikrosk Anat 1971; 112:371 - 89; http://dx.doi.org/10.1007/BF02584050; PMID: 5542328
- Hyde GJ, Hardham AR. Confocal microscopy of microtubule arrays in cryosectioned sporangia of Phytophthora cinnamomi.. Exp Mycol 1992; 16:207 - 18; http://dx.doi.org/10.1016/0147-5975(92)90029-Q
- Ketelaar T, Meijer HJG, Spiekerman M, Weide R, Govers F. Effects of latrunculin B on the actin cytoskeleton and hyphal growth in Phytophthora infestans.. Fungal Genet Biol 2012; 49:1014 - 22; http://dx.doi.org/10.1016/j.fgb.2012.09.008; PMID: 23036581
- Luna E, Pastor V, Robert J, Flors V, Mauch-Mani B, Ton J. Callose deposition: a multifaceted plant defense response. Mol Plant Microbe Interact 2011; 24:183 - 93; http://dx.doi.org/10.1094/MPMI-07-10-0149; PMID: 20955078
- Hardham AR, Takemoto D, White RG. Rapid and dynamic subcellular reorganization following mechanical stimulation of Arabidopsis epidermal cells mimics responses to fungal and oomycete attack. BMC Plant Biol 2008; 8:63; http://dx.doi.org/10.1186/1471-2229-8-63; PMID: 18513448