Abstract
Arabidopsis genes MYR1 and MYR2 are regulators of flowering time under low light intensity. These Myb-related genes are expressed as alternative splice variants affected in their coiled-coil and DNA-binding domains. We tested whether alternative splicing could affect dimerization and localization of MYR1 and MYR2, thereby potentially affecting their activity. Using MYR1 as a model for variants within the coiled-coil region, we detected 2 types of homodimers. For MYR2, alternative splicing in the DNA-binding Myb-like domain abolished the ability of MYR2 to dimerize. Alternative splicing in the coiled-coil domain did not affect nuclear localization, as determined by transient expression in tobacco, while alternative splicing in the DNA-binding domain of MYR2 yielded a distinct intranuclear localization pattern that may reflect changes in phosphorylation-dependent protein folding. Thus alternative splicing of these genes may result in changes in dimerization or protein folding resulting in changes in activity and abundance of MYR1 or MYR2 protein.
MYR1 and MYR2 are members of the G2-like family of Myb transcription factors. The G2-like family belongs to the GARP superfamily, which was named after maize GOLDEN2,Citation1 the ARR B-class proteins from Arabidopsis,Citation2 and Chlamydomonas Psr1.Citation3 G2-like proteins possess one copy of the Myb-like DNA-binding domain. The G2-like family consists of about 42 members in Arabidopsis. Previously characterized G2-like proteins include KANADI1–4, APL, and GLK1–2, which are required for establishment of polarity in lateral organs,Citation4,Citation5 phloem identity,Citation6 and chloroplast development,Citation7 respectively. We recently demonstrated that the G2-like paralogous genes MYR1 and MYR2 exhibit phloem-specific expression and are redundant negative regulators of flowering time under low light intensity.Citation8 Together, these reports demonstrate that the G2-like family plays important roles in diverse aspects of plant growth and development.
MYR1 and MYR2 belong to subgroup II comprised of 15 Myb-Coiled-Coil (Myb-CC) transcription factors in Arabidopsis, containing a central CC region, an N-terminal Myb-like DNA-binding domain, and a C-terminal transactivation domain.Citation8,Citation9 The Myb-like DNA-binding domain, as represented by the ARR B-class protein ARR10, consists of 3 α-helices connected by a type-I and type-II β-turn. The α2 helix, type-II β-turn, and α3 helix form an HTH variant motif. The residues on the HTH motif, especially those on helix α3, bind the major groove of target DNA, while the N-terminal flexible arm contacts the minor groove.Citation10 Both MYR1 and MYR2 are expressed as several alternatively spliced isoforms (). Alternative splicing occurs immediately after the highly conserved LHEQLE sequence motif in the CC region (affecting MYR1 and MYR2) and within the type-I β-turn connecting helices α1 and α2 in the Myb-like DNA-binding domain (affecting MYR2 only). All splicing variants are the result of 3′ splice site variation. G2-like genes UNE16Citation11 and At3g24120 also undergo alternative splicing at the same CC site as MYR1 and MYR2.
Figure 1. Alternative splicing of MYR1 and MYR2 affects dimerization and localizations (A) Schematic diagram of alternative splicing in MYR1 and MYR2. Both MYR1 and MYR2 contain 3 domains: Myb-like DNA-binding domain (Myb), coiled-coil domain (CC), and transactivation domain (TA). Conserved alternative splicing at the CC domain of MYR1 and MYR2 results in 3 isoforms. In addition, alternative splicing at the Myb-like DNA-binding domain of MYR2 yields 2 isoforms. (B) MYR1 isoforms produced from alternative splicing at the CC domain form 2 distinct homodimers in yeast and possibly one heterodimer. Yeast AH109 cells co-transformed with 2 plasmid DNAs as shown in the interaction grid were spotted on SD plates lacking tryptophan and leucine (-T -L), tryptophan, leucine, and histidine (-T -L -H), or tryptophan, leucine, and adenine (-T -L -A), and incubated at 30 °C for 3–10 d. (C) MYR2 isoform At3g04030.2, produced from alternative splicing at the Myb domain, forms neither a homodimer nor a heterodimer with At3g04030.2 or At3g04030.3, respectively, while At3g04030.3 can form a homodimer. For different MYR2 isoform assays (C, at right) yeast AH109 was co-transformed with 2 plasmid DNAs, as exemplified by the DB-At3g04030.3/AD-At3g04030.3 assay using plasmid combinations 1 through 5: 1, DB-At3g04030.3, AD-At3g04030.3; 2, DB-At3g04030.3, AD; 3, DB, AD-At3g04030.3; 4, DB, AD; 5, positive control (pGBKT7–53 + pGADT7-RecT), and grown on drop-out plates as shown. (D-H) Subcellular localization of GFP-MYR2 isoforms and mutants transiently expressed in Nicotiana benthamiana. MYR2 isoform GFP-At3g04030.3 was evenly distributed throughout the nucleus in all cells (D). In most cells (79%), GFP-At3g04030.2 was also evenly distributed throughout the nucleus. However, in 21% of cells (n = 1771), a portion of GFP-At3g04030.2 localized to a single spot or multiple spots (inset in red box) in the nucleus (E and H, left panel). Insets in white boxes (D, E) show GFP/DAPI overlay images for nuclei. Point mutants K70A (F) or K74A (not shown) of GFP-At3g04030.3 mimic the nuclear spot localization pattern seen for GFP-At3g04030.2. The double mutant (K70A K74A, K70A K78A, and K74A K78A) forms of GFP-At3g04030.3 aggregate outside of the nucleus in some cells, while triple mutant (K70A K74A K78A) GFP-At3g04030.3 forms extra-nuclear aggregates in all GFP-positive cells (G). Nucleus in (G) is surrounded by dashed white line. T72E (At3g04030.3) (H, center panel; n = 445) or T71E (At3g04030.2) (H, right panel; n = 715) mutations enhanced the localization of GFP to one or multiple spots in the nucleus. Categories of GFP localization (H) are: 1, evenly distributed throughout the nucleus; 2, localized to a single nuclear spot; 3, localized to multiple nuclear spots. Bar in (D) = 10 µm for (D-G).
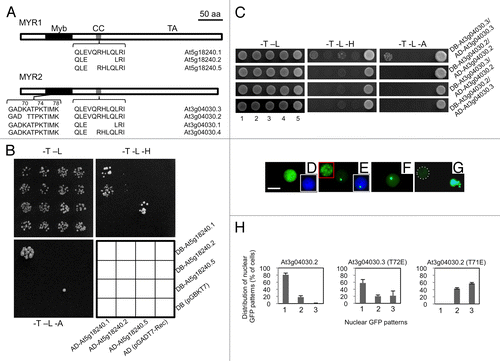
Although the majority of intron-containing genes in Arabidopsis produce alternatively spliced transcripts,Citation12 physiological roles for isoforms derived from alternative splicing are currently known for only a few plant genes. For a recent review see ref. Citation13. Distinct functions of protein isoforms can result from altered protein–protein, protein–DNA, or protein–ligand interactions as well as changes in post-translational modifications, stability, or localization.Citation14 As alternative splicing of MYR1 and MYR2 affects domains involved in protein–protein interaction (CC domain) or localization and DNA binding (Myb-like domain) we used yeast two-hybrid (Y2H) analysis and transient expression of GFP fusions to study isoform dimerization and localization, 2 factors that could influence activities of MYR1 and MYR2.
Alternative splicing within the CC domains of MYR1 and MYR2 is conserved (). Hence, only MYR1 was used to study interactions among CC-related isoforms. In yeast, the longest MYR1 CC domain (residing within aa residues 119–233 from At5g18240.1 used for Y2H involving MYR1) homodimerized and supported the most rapid yeast growth on both –His and –Ade plates, while homodimerization of the polypeptide with a 2-aa deletion from the CC region (At5g18240.5) supported slower yeast growth (). In contrast, a 6-aa deletion from the CC domain (At5g18240.2) prevented homodimerization detectable in 3–10 d incubation, although with long-term incubation (16 d) yeast growth was detectable on both –His and –Ade plates (data not shown). Interestingly, a heterodimer involving At5g18240.1 and At5g18240.2 was detectable, but only on –His plates and only with one AD/DB fusion combination (). For MYR2, the Myb-like and CC domains (aa residues 1–344) but not the C-terminal activation domain were used to test the effects of alternative splicing in the Myb-like domain. The MYR2 isoform At3g04030.2 formed neither a homodimer nor a heterodimer with At3g04030.2 or At3g04030.3, respectively, while At3g04030.3 formed a homodimer (). As the CC domain was identical for both of these MYR2 isoforms, effects on dimerization were due to differences in the Myb-like domain. The varying abilities of the MYR1 CC isoforms to support yeast reporter activation as homodimers suggests that relative levels of MYR1 isoforms in plants cells may affect strength of dimerization and interactions with the as yet unknown targets of MYR1.
For At3g04030.2, alternative splicing at the Myb-like DNA-binding domain results in a deletion of 3 nucleotides affecting 2 aa residues: deleting K70 and causing an A71T substitution within the type-I β-turn connecting helices α1 and α2 () compared with At3g04030.3. As previously reported for GFP-MYR1,Citation8 GFP-MYR2 based on the longest isoform (At3g04030.3) localized exclusively to and was evenly distributed throughout the nucleus of tobacco epidermal cells (). While alternative splicing within the MYR1 or MYR2 CC domain did not alter this nuclear pattern of localization (data not shown), alternative splicing within the Myb-like domain for isoform At3g04030.2 resulted in the appearance of GFP localized to one or more spots within the nucleus in 21% of GFP-positive cells ( and , left panel), with the remaining cells showing GFP evenly distributed throughout the nucleus as noted for At3g04030.3 (). The varying number of intranuclear GFP spots observed for cells expressing isoform At3g04030.2 suggests that protein aggregation promoted by disruption of the β-turn within the Myb-like domain of MYR2Citation15 rather than localization to a specific intranuclear compartment explains the altered appearance of GFP fused to isoform At3g04030.2 compared with At3g04030.3 ( vs. )
To further examine the influence of the aa changes within the β-turn of MYR2, we tested several mutant versions of At3g04030.3, including mutation of K70, along with nearby basic residues, K74 and K78 (), in all possible combinations, to Ala or Arg, and T72 to Ala or Glu. The latter 2 mutations were introduced to mimic a constitutive non-phosphorylated (T72A) or phosphorylated (T72E) Thr residue. We discovered that the K70A () or K74A (data not shown) substitutions in At3g04030.3 yielded a small percentage of GFP-positive cells with the same altered intranuclear distribution of GFP observed for At3g04030.2, i.e., GFP concentrated in one or more nuclear spots (). Further, Ala substitution of any 2 of residues K70, K74, or K78 resulted in localization of GFP-MYR2 to extra-nuclear aggregates for a small percentage of cells, while Ala substitution of all three Lys residues resulted in near complete loss of nuclear localization and caused GFP-MYR2 to form extra-nuclear aggregates in all GFP-positive cells (). Substitution of any or all aa residues K70, K74, or K78 with Arg produced evenly distributed nuclear localization identical to that observed for At3g04030.3 (data not shown), confirming the importance of basic aa residues at these positions for normal nuclear localization and solubility.
Interestingly, the T72E mutation in At3g04030.3 mimicked the At3g04030.2 localization, and led to a substantial increase in the proportion of GFP-positive cells showing GFP concentrated in nuclear spots (, compare left panel to center panel) and the effect of Thr to Glu substitution was further enhanced by the equivalent mutation (T71E) in At3g04030.2 (, right panel). In contrast, T72A or T71A mutations in At3g04030.3 or At3g04030.2, respectively, did not change GFP localization from that observed for T72 and T71 versions of At3g04030.3 and At3g04030.2 (data not shown). Considering that T72 is immediately followed by Pro (), it is possible that 72TP is a potential Ser/Thr-Pro phosphorylation motif, a regulatory site implicated in many cellular activities.Citation16 Importantly, this Pro is conserved as the first residue of helix α2 in nearly all GARP superfamily members, approximately half of which possess the Ser/Thr-Pro phosphorylation motif, indicating that cis/trans prolyl isomerization,Citation17 with phosphorylation-dependent regulationCitation16 for many GARPs, is an important regulatory mechanism in this family.
We have demonstrated the potential for alternative splicing of MYR1 and MYR2 to yield proteins with isoform-specific homodimerization characteristics. Additionally, disruptions in the β-turn region within the Myb-like domain of MYR2 could increase the likelihood of improper protein folding and aggregation. The relative levels of alternatively spliced isoforms of MYR1 and MYR2 together with phosphorylation-dependent cis/trans prolyl isomerization may contribute to modulation of MYR1 and MYR2 activities as regulators of flowering time.
Abbreviations: | ||
aa | = | amino acid |
Ade | = | adenine |
CC | = | coiled-coil |
GFP | = | green fluorescent protein |
Y2H | = | yeast two-hybrid |
Disclosure of Potential Conflicts of Interest
No potential conflicts of interest were disclosed.
Acknowledgments
This work was supported by the Office of Science (BER), US Department of Energy, Grant No. DE-FG02–07ER64449, and USDA-NIFA, Project No. VA-135994.
References
- Hall LN, Rossini L, Cribb L, Langdale JA. GOLDEN 2: a novel transcriptional regulator of cellular differentiation in the maize leaf. Plant Cell 1998; 10:925 - 36; PMID: 9634581
- Sakai H, Aoyama T, Bono H, Oka A. Two-component response regulators from Arabidopsis thaliana contain a putative DNA-binding motif. Plant Cell Physiol 1998; 39:1232 - 9; http://dx.doi.org/10.1093/oxfordjournals.pcp.a029325; PMID: 9891419
- Wykoff DD, Grossman AR, Weeks DP, Usuda H, Shimogawara K. Psr1, a nuclear localized protein that regulates phosphorus metabolism in Chlamydomonas. Proc Natl Acad Sci U S A 1999; 96:15336 - 41; http://dx.doi.org/10.1073/pnas.96.26.15336; PMID: 10611385
- Kerstetter RA, Bollman K, Taylor RA, Bomblies K, Poethig RS. KANADI regulates organ polarity in Arabidopsis. Nature 2001; 411:706 - 9; http://dx.doi.org/10.1038/35079629; PMID: 11395775
- Emery JF, Floyd SK, Alvarez J, Eshed Y, Hawker NP, Izhaki A, Baum SF, Bowman JL. Radial patterning of Arabidopsis shoots by class III HD-ZIP and KANADI genes. Curr Biol 2003; 13:1768 - 74; http://dx.doi.org/10.1016/j.cub.2003.09.035; PMID: 14561401
- Bonke M, Thitamadee S, Mähönen AP, Hauser MT, Helariutta Y. APL regulates vascular tissue identity in Arabidopsis.. Nature 2003; 426:181 - 6; http://dx.doi.org/10.1038/nature02100; PMID: 14614507
- Fitter DW, Martin DJ, Copley MJ, Scotland RW, Langdale JA. GLK gene pairs regulate chloroplast development in diverse plant species. Plant J 2002; 31:713 - 27; http://dx.doi.org/10.1046/j.1365-313X.2002.01390.x; PMID: 12220263
- Zhao C, Hanada A, Yamaguchi S, Kamiya Y, Beers EP. The Arabidopsis Myb genes MYR1 and MYR2 are redundant negative regulators of flowering time under decreased light intensity. Plant J 2011; 66:502 - 15; http://dx.doi.org/10.1111/j.1365-313X.2011.04508.x; PMID: 21255164
- Rubio V, Linhares F, Solano R, Martín AC, Iglesias J, Leyva A, Paz-Ares J. A conserved MYB transcription factor involved in phosphate starvation signaling both in vascular plants and in unicellular algae. Genes Dev 2001; 15:2122 - 33; http://dx.doi.org/10.1101/gad.204401; PMID: 11511543
- Hosoda K, Imamura A, Katoh E, Hatta T, Tachiki M, Yamada H, Mizuno T, Yamazaki T. Molecular structure of the GARP family of plant Myb-related DNA binding motifs of the Arabidopsis response regulators. Plant Cell 2002; 14:2015 - 29; http://dx.doi.org/10.1105/tpc.002733; PMID: 12215502
- Pagnussat GC, Yu HJ, Ngo QA, Rajani S, Mayalagu S, Johnson CS, Capron A, Xie LF, Ye D, Sundaresan V. Genetic and molecular identification of genes required for female gametophyte development and function in Arabidopsis. Development 2005; 132:603 - 14; http://dx.doi.org/10.1242/dev.01595; PMID: 15634699
- Marquez Y, Brown JW, Simpson C, Barta A, Kalyna M. Transcriptome survey reveals increased complexity of the alternative splicing landscape in Arabidopsis. Genome Res 2012; 22:1184 - 95; http://dx.doi.org/10.1101/gr.134106.111; PMID: 22391557
- Carvalho RF, Feijão CV, Duque P. On the physiological significance of alternative splicing events in higher plants. Protoplasma 2013; 250:639 - 50; http://dx.doi.org/10.1007/s00709-012-0448-9; PMID: 22961303
- Stamm S, Ben-Ari S, Rafalska I, Tang Y, Zhang Z, Toiber D, Thanaraj TA, Soreq H. Function of alternative splicing. Gene 2005; 344:1 - 20; http://dx.doi.org/10.1016/j.gene.2004.10.022; PMID: 15656968
- Marcelino AM, Gierasch LM. Roles of beta-turns in protein folding: from peptide models to protein engineering. Biopolymers 2008; 89:380 - 91; http://dx.doi.org/10.1002/bip.20960; PMID: 18275088
- Thorpe JR, Mosaheb S, Hashemzadeh-Bonehi L, Cairns NJ, Kay JE, Morley SJ, Rulten SL. Shortfalls in the peptidyl-prolyl cis-trans isomerase protein Pin1 in neurons are associated with frontotemporal dementias. Neurobiol Dis 2004; 17:237 - 49; http://dx.doi.org/10.1016/j.nbd.2004.07.008; PMID: 15474361
- Lu KP, Finn G, Lee TH, Nicholson LK. Prolyl cis-trans isomerization as a molecular timer. Nat Chem Biol 2007; 3:619 - 29; http://dx.doi.org/10.1038/nchembio.2007.35; PMID: 17876319