Abstract
In non-cyanogenic plants, cyanide is a co-product of ethylene and camalexin biosynthesis. To maintain cyanide at non-toxic levels, Arabidopsis plants express the mitochondrial β-cyanoalanine synthase CYS-C1. CYS-C1 knockout leads to an increased level of cyanide in the roots and leaves and a severe defect in root hair morphogenesis, suggesting that cyanide acts as a signaling factor in root development. During compatible and incompatible plant-bacteria interactions, cyanide accumulation and CYS-C1 gene expression are negatively correlated. Moreover, CYS-C1 mutation increases both plant tolerance to biotrophic pathogens and their susceptibility to necrotrophic fungi, indicating that cyanide could stimulate the salicylic acid-dependent signaling pathway of the plant immune system. We hypothesize that CYS-C1 is essential for maintaining non-toxic concentrations of cyanide in the mitochondria to facilitate cyanide’s role in signaling.
Cyanide is a low molecular weight molecule that reacts with keto compounds and Schiff base intermediates to yield cyanohydrins and stable nitrile derivatives, respectively, and it chelates di- and trivalent metal ions in the prosthetic groups of several metalloproteins, affecting their function.Citation1 Cyanide is most lethal in mitochondria, where it binds to the heme iron of cytochrome c oxidase and blocks oxidative phosphorylation, thereby impairing vital functions.Citation2 Despite its toxicity, cyanide is present in a wide range of organisms of all kingdoms including bacteria, fungi, insects, and plants.Citation3-Citation5 Cyanogenic plants synthesize cyanogenic compounds to poison herbivores by releasing cyanide.Citation6,Citation7 In non-cyanogenic plants, cyanide is a co-product of ethylene and camalexin biosynthesis, both of which are molecules involved in plant defense against pathogens.Citation8-Citation10
Ethylene and camalexin are synthesized by the action of the 1-aminocyclopropane-1-carboxylic acid oxidase oxidase and the P450 enzyme PAD3 (CYP71B15), respectively, in the plant cell cytosol,Citation11-Citation13 where cyanide is produced. HCN is gaseous but can partially dissolve in water. It is weakly acidic and can dissociate into H+ and CN– in aqueous solution, especially in more basic solutions. Because the cytosolic pH is close to 7, it is expected that cyanide would be mostly present as hydrogen cyanide (HCN) in this compartment, according to its pKa of 9.3. The moderate lipid solubility and small size of the HCN molecule allow HCN to cross membranes rapidly and enter mitochondria, where the respiration-associated proton pumps transport protons from the matrix to the inner and outer mitochondrial membranes, thus raising the matrix pH to approximately 8. Hence, HCN would dissociate into H+ and CN– at a high level in the mitochondrial matrix, preventing its escape from this organelle, which is its main target. These physicochemical properties, together with its moderate reactivity and its ability to modify protein activity, make cyanide a candidate signaling molecule.
In plants, cyanide has been proposed to act as a regulator of biological processes, such as seed dormancy and germination,Citation4,Citation14,Citation15 and to play a role in resistance to viral and fungal pathogens.Citation16-Citation18 The use of exogenously applied cyanide has led to these conclusions; however, our work has clarified the role of endogenously produced cyanide.
Cyanide must be rapidly detoxified and metabolized by the plant to maintain the concentration below toxic levels. Arabidopsis plants carry the mitochondrial β-cyanoalanine synthase (CAS) CYS-C1,Citation19 which, together with O-acetylserine(thiol)lyase (OASTL), belongs to the family of β-substituted alanine synthase enzymes. CAS is a pyridoxal phosphate-dependent enzyme that uses cysteine to detoxify cyanide by converting cyanide and cysteine into hydrogen sulfide (H2S) and β-cyanoalanine, which is later converted to Asn, Asp, and ammonia by NIT4 nitrilases, recycling the nitrogen for the plant.Citation20,Citation21 The CAS activity and its role in cyanide detoxification have also been described in other plants, such as rice and soybean.Citation22-Citation24 Mutants lacking CAS activity would therefore allow the investigation of the role of endogenously produced cyanide.
The CYS-C1 loss-of-function mutation is not toxic for the plant and leads to an increased level of cyanide in the cys-c1 mutant as well as a root hairless phenotype, suggesting that endogenously produced cyanide plays a signaling role in root development.Citation25 The root hair defect is phenocopied in wild-type plants by the exogenous addition of cyanide to the growth medium and is reversed by the addition of hydroxocobalamin, which is the most commonly used antidote for severe acute cyanide poisoning in humans.Citation25
Because cyanide production is closely linked to the biosynthesis of metabolites involved in the immune response, the effect of cyanide in the plant response to pathogens has been analyzed.Citation26 In particular, it has been observed that cys-c1 plants present an increased susceptibility to the necrotrophic fungus Botrytis cinerea and an increased tolerance to the biotrophic bacterium Pseudomonas syringae pv tomato DC3000 as well as the beet curly top virus. The cys-c1 mutation reduces the respiration rate in leaves, causes the accumulation of reactive oxygen species, and induces the expression of alternative oxidase AOX1a and the pathogenesis-related PR1. Interestingly, no patches of dead cells are observed when this mutant is grown under either a long or short photoperiod. We hypothesize that cyanide, which is transiently accumulated during avirulent bacterial infection and constitutively accumulated in the cys-c1 mutant, generates a mitochondrial signal, the nature of which is yet to be identified. The fact that uncoupling of oxidative respiration, induction of alternative oxidase activity, and accumulation of reactive oxygen species (ROS) are observed in cys-c1 plants suggests that ROS generated in mitochondria could induce a signal that generates a series of events that modulate the salicylic acid-dependent signaling pathway of the plant immune system. ROS generation is essential for the establishment of the signaling pathway leading to resistance to biotrophic pathogens in the initial steps of the interaction,Citation27 and ROS modulate late steps in the SA-dependent response pathway, such as the import of NPR1 into the nucleus or SA biosynthesis and accumulation.Citation28,Citation29 A direct role for cyanide in the generation of this mitochondrial signal cannot be excluded, although this is very unlikely ().
Figure 1. Cyanide and the plant immune response. Pathogen attack induces the biosynthesis of hormones, mainly jasmonic acid (JA) and ethylene (ET) in response to necrotrophic pathogens and salicylic acid (SA) in response to biotrophic pathogens, although biotrophes can also induce ET biosynthesis. In our model, cyanide, which is produced concomitantly to ethylene, enters mitochondria where it generates a direct and/or indirect a signal that stimulates the SA-dependent response pathway.
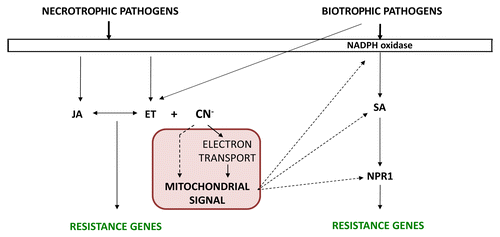
In conclusion, mitochondrial β-cyanoalanine synthase activity is essential for maintaining non-toxic concentrations of cyanide; however, the transient repression of the CYS-C1 gene and the accumulation of cyanide in response to specific pathogens suggest the existence of a signaling mechanism that is dependent on cyanide, probably through the controlled accumulation of ROS in this organelle. The mechanisms underlying this modulation and, especially, the mode of action and specific targets of cyanide are open questions and are the focus of our present research.
Disclosure of Potential Conflicts of Interest
No potential conflicts of interest were disclosed.
Acknowledgments
We would like to thank the European Regional Development Fund, the Ministerio de Economía y Competitividad of Spain (grant no. BIO2010–15201 and CSD2007–00057), and Junta de Andalucía of Spain (grant no. CVI-7190) for their financial support.
References
- Nagahara N, Ito T, Minami M. Mercaptopyruvate sulfurtransferase as a defense against cyanide toxication: molecular properties and mode of detoxification. Histol Histopathol 1999; 14:1277 - 86; PMID: 10506943
- Donato DB, Nichols O, Possingham H, Moore M, Ricci PF, Noller BN. A critical review of the effects of gold cyanide-bearing tailings solutions on wildlife. Environ Int 2007; 33:974 - 84; http://dx.doi.org/10.1016/j.envint.2007.04.007; PMID: 17540445
- Knowles CJ. Microorganisms and cyanide. Bacteriol Rev 1976; 40:652 - 80; PMID: 791236
- Siegien I, Bogatek R. Cyanide action in plants - from toxic to regulatory. Acta Physiol Plant 2006; 28:483 - 97; http://dx.doi.org/10.1007/BF02706632
- Zagrobelny M, Bak S, Møller BL. Cyanogenesis in plants and arthropods. Phytochemistry 2008; 69:1457 - 68; http://dx.doi.org/10.1016/j.phytochem.2008.02.019; PMID: 18353406
- Miller JM, Conn EE. Metabolism of hydrogen cyanide by higher plants. Plant Physiol 1980; 65:1199 - 202; http://dx.doi.org/10.1104/pp.65.6.1199; PMID: 16661359
- Conn EE. Our work with cyanogenic plants. Annu Rev Plant Biol 2008; 59:1 - 19; http://dx.doi.org/10.1146/annurev.arplant.59.032607.092924; PMID: 17988213
- Glawischnig E. Camalexin. Phytochemistry 2007; 68:401 - 6; http://dx.doi.org/10.1016/j.phytochem.2006.12.005; PMID: 17217970
- Wang KLC, Li H, Ecker JR. Ethylene biosynthesis and signaling networks. Plant Cell 2002; 14:S131 - 51; PMID: 12045274
- Yip WK, Yang SF. Cyanide metabolism in relation to ethylene production in plant tissues. Plant Physiol 1988; 88:473 - 6; http://dx.doi.org/10.1104/pp.88.2.473; PMID: 16666329
- Schuler MA, Werck-Reichhart D. Functional genomics of P450s. Annu Rev Plant Biol 2003; 54:629 - 67; http://dx.doi.org/10.1146/annurev.arplant.54.031902.134840; PMID: 14503006
- Panstruga R, Parker JE, Schulze-Lefert P. SnapShot: Plant immune response pathways. Cell 2009; 136:e1 - 3; http://dx.doi.org/10.1016/j.cell.2009.02.020; PMID: 19269372
- Chung MC, Chou SJ, Kuang LY, Charng YY, Yang SF. Subcellular localization of 1-aminocyclopropane-1-carboxylic acid oxidase in apple fruit. Plant Cell Physiol 2002; 43:549 - 54; http://dx.doi.org/10.1093/pcp/pcf067; PMID: 12040102
- Bethke PC, Libourel IG, Reinöhl V, Jones RL. Sodium nitroprusside, cyanide, nitrite, and nitrate break Arabidopsis seed dormancy in a nitric oxide-dependent manner. Planta 2006; 223:805 - 12; http://dx.doi.org/10.1007/s00425-005-0116-9; PMID: 16151848
- Cohn MA, Hughes JA. Seed dormancy in red rice: v. Response to azide, hydroxylamine, and cyanide. Plant Physiol 1986; 80:531 - 3; http://dx.doi.org/10.1104/pp.80.2.531; PMID: 16664656
- Chivasa S, Carr JP. Cyanide restores N gene-mediated resistance to tobacco mosaic virus in transgenic tobacco expressing salicylic acid hydroxylase. Plant Cell 1998; 10:1489 - 98; PMID: 9724695
- Wong CE, Carson RA, Carr JP. Chemically induced virus resistance in Arabidopsis thaliana is independent of pathogenesis-related protein expression and the NPR1 gene. Mol Plant Microbe Interact 2002; 15:75 - 81; http://dx.doi.org/10.1094/MPMI.2002.15.1.75; PMID: 11858174
- Seo S, Mitsuhara I, Feng J, Iwai T, Hasegawa M, Ohashi Y. Cyanide, a coproduct of plant hormone ethylene biosynthesis, contributes to the resistance of rice to blast fungus. Plant Physiol 2011; 155:502 - 14; http://dx.doi.org/10.1104/pp.110.162412; PMID: 21075959
- Watanabe M, Kusano M, Oikawa A, Fukushima A, Noji M, Saito K. Physiological roles of the beta-substituted alanine synthase gene family in Arabidopsis. Plant Physiol 2008; 146:310 - 20; http://dx.doi.org/10.1104/pp.107.106831; PMID: 18024555
- Floss HG, Hadwiger L, Conn EE. Enzymatic formation of beta-cyanoalanine from cyanide. Nature 1965; 208:1207 - 8; http://dx.doi.org/10.1038/2081207a0; PMID: 4958370
- Piotrowski M. Primary or secondary? Versatile nitrilases in plant metabolism. Phytochemistry 2008; 69:2655 - 67; http://dx.doi.org/10.1016/j.phytochem.2008.08.020; PMID: 18842274
- Yi H, Jez JM. Assessing functional diversity in the soybean β-substituted alanine synthase enzyme family. Phytochemistry 2012; 83:15 - 24; http://dx.doi.org/10.1016/j.phytochem.2012.08.003; PMID: 22986002
- Yi H, Juergens M, Jez JM. Structure of soybean β-cyanoalanine synthase and the molecular basis for cyanide detoxification in plants. Plant Cell 2012; 24:2696 - 706; http://dx.doi.org/10.1105/tpc.112.098954; PMID: 22739827
- Lai KW, Yau CP, Tse YC, Jiang L, Yip WK. Heterologous expression analyses of rice OsCAS in Arabidopsis and in yeast provide evidence for its roles in cyanide detoxification rather than in cysteine synthesis in vivo. J Exp Bot 2009; 60:993 - 1008; http://dx.doi.org/10.1093/jxb/ern343; PMID: 19181864
- García I, Castellano JM, Vioque B, Solano R, Gotor C, Romero LC. Mitochondrial beta-cyanoalanine synthase is essential for root hair formation in Arabidopsis thaliana. Plant Cell 2010; 22:3268 - 79; http://dx.doi.org/10.1105/tpc.110.076828; PMID: 20935247
- García I, Rosas T, Bejarano ER, Gotor C, Romero LC. Transient transcriptional regulation of the CYS-C1 gene and cyanide accumulation upon pathogen infection in the plant immune response. Plant Physiol 2013; 162:2015 - 27; http://dx.doi.org/10.1104/pp.113.219436; PMID: 23784464
- Torres MA, Dangl JL. Functions of the respiratory burst oxidase in biotic interactions, abiotic stress and development. Curr Opin Plant Biol 2005; 8:397 - 403; http://dx.doi.org/10.1016/j.pbi.2005.05.014; PMID: 15939662
- Kinkema M, Fan W, Dong X. Nuclear localization of NPR1 is required for activation of PR gene expression. Plant Cell 2000; 12:2339 - 50; PMID: 11148282
- Xing F, Li Z, Sun A, Xing D. Reactive oxygen species promote chloroplast dysfunction and salicylic acid accumulation in fumonisin B1-induced cell death. FEBS Lett 2013; 587:2164 - 72; http://dx.doi.org/10.1016/j.febslet.2013.05.034; PMID: 23711368