Abstract
WRKY transcription factors are one of the largest families of transcriptional regulators found exclusively in plants. They have diverse biological functions in plant disease resistance, abiotic stress responses, nutrient deprivation, senescence, seed and trichome development, embryogenesis, as well as additional developmental and hormone-controlled processes. WRKYs can act as transcriptional activators or repressors, in various homo- and heterodimer combinations. Here we review recent progress on the function of WRKY transcription factors in Arabidopsis and other plant species such as rice, potato, and parsley, with a special focus on abiotic, developmental, and hormone-regulated processes.
Introduction
Reprogramming of a cell in response to external stimuli involves complex changes in gene expression. The activities of a multitude of genes are subject to up- or downregulation and follow defined temporal programs. The perception of an external stimulus leads to the activation of primary response genes. WRKY transcription factors (TFs) are one of the largest families of transcriptional regulators in plants with 72 representatives in Arabidopsis, and more than 100 members in rice, soybean or popular.Citation1-Citation13 Sixty-eight genes have been identified in Sorghum,Citation13 38 in Physcomitrella patens,Citation13 35 in Sellaginella moellendorffii,Citation1 80 in pinus,Citation14 and about 45 in barley.Citation15 WRKYs contain the highly conserved amino acid sequence WRKYGQK and the zinc-finger-like motifs Cys(2)-His(2) or Cys(2)-HisCys, and bind to the TTGAC(C/T) W-box cis-element in the promoter of their target genes.Citation1,Citation2 WRKY TFs are important components of a plant signaling web which regulate many plant processes in response to biotic and abiotic stimuli, but also in response to internal signals which coordinate developmental processes, and include additional DNA-binding and non-DNA-binding proteins as interaction partners.Citation1-Citation5,Citation7,Citation9,Citation10,Citation13,Citation16-Citation26 WRKYs can act as activators or repressors, and by doing so, they establish a TF net that participates in many cytoplasmic and nuclear processes including signaling events from organelles and the cytoplasm to the nucleus. Stress responses often involve hormone signaling, therefore, WRKYs are also part of a complex hormone signaling net. The WRKYs can function up- and downstream of hormones, are involved in the antagonistic functions of salicylic acid (SA) and jasmonic acid (JA)/ethylene (ET), control developmental processes via auxins, cytokinins, and brassinosteroids.Citation2,Citation18,Citation20,Citation21,Citation25 The SA-mediated signaling pathway is associated with resistance to biotrophic and hemibiotrophic pathogens, while JA/ET signaling often correlates with resistance to necrotrophic pathogens.Citation27,Citation28 These phytohormones have different effects on WRKY TFs, e.g., WRKY70 acts as an activator of SA-induced genes and as a repressor of JA-responsive genes, thereby integrating signals from these mutually antagonistic pathways.Citation29 There is increasing evidence for an important control of WRKY genes by abscisic acid (ABA)Citation18,Citation20,Citation30,Citation31 and ETCitation30,Citation32-Citation34 signaling. ABA represents a key signal to regulate plant growth and development as well as plant responses to abiotic and biotic stress. The WRKY domain in WRKY2, WRKY18, WRKY40, WRKY60, and WRKY63 are also reported in transcriptional regulation of ABA-responsive element binding factors (ABFs/AREBs) through W-box sequences present in the promoters of ABFs/AREBs.Citation35 ABA is involved in drought responses, and ABA signaling components in the nucleus are targets of signals from plastids and mitochondria, thereby participating in retrograde signaling in plant cells. Vanderauwera et al.Citation36 proposed that WRKY15 participates in mitochrondrium-to-nucleus signaling. Finally auxinCitation30,Citation31,Citation33,Citation37-Citation41 and cytokininCitation42-Citation44 may control plant developmental processes with the help of WRKYs. Phosphate (Pi) limitation is another example of stress which is counteracted by the plant with the help of WRKY6 and WRKY75 TFs.Citation21 Besides phytohromones, biotic and abiotic stress responses are often associated with the generation of reactive oxygen species (ROS) in plants.Citation23 Several ROS-dependent responses are controlled by WRKYs, including the progression of senescence.Citation5 Finally, WRKYs actively participate in the control of seed, embryo, microphyle, and endosperm development.Citation42,Citation43,Citation45,Citation46 Members of the WRKY family have also been reported to regulate major changes in plant transcriptome during early phases of root colonization with arbuscular mycorrhizal fungi.Citation47 These observations raise the question how so diverse responses can be controlled by WRKYs. We address the question whether a common theme maybe behind these processes. We summarize the current knowledge on this TF family, with the main focus on abiotic stress responses, as well as control of plant development and hormone regulation, and putative common processes.
WRKY: Structure and Classification
The WRKY name is coined from the highly conserved 60 amino acid long WRKY domains of the TFs, which contain a conserved heptapeptide motif WRKYGQK at the N-terminus and a novel zinc-finger-like motif at the C-terminus. Both heptapeptide sequence and zinc-finger-like motif are required for the high binding affinity of WRKY TFs to the consensus cis-acting elements termed W box (TTGACT/C).
In spite of the presence of the highly conserved W box, DNA binding of WRKY TFs varies mainly due to the different number of DNA binding domains and different features of the zinc-finger-like motifs. Based on these features Eulgem et al.Citation26 classified WRKY proteins into 3 groups:
WRKY proteins with 2 WRKY domains belong to group I, whereas most proteins with one WRKY domain belong to group II. Both group I and group II proteins have the same pattern of potential zinc ligands (C–X4–5–C–X22–23–H–X1–H). WRKY proteins having only one WRKY domain but different patterns of zinc finger motifs are categorized into group III. Instead of a C2–H2 pattern, group III WRKY domains contain a C2–HC motif (C–X7–C–X23–H–X1–C). Group II proteins were further divided into IIa, IIb, IIc, IId, and IIe based on the primary amino acid sequence (). The WRKY domain consists of a 4-stranded β-sheet, with the zinc coordinating Cys/His residues forming a zinc-binding pocket. 72 WRKY genes are present in the Arabidopsis genome and approximately 100 WRKY genes in the rice genomes, respectively.Citation48 Out of the 72 Arabidopsis WRKY genes, 32 belong to group I, 26 belong to group II and 14 belong to group III. In spite of different DNA binding domains members of all groups bind sequence-specifically to various W-box elements. The 2 WRKY domains of group I members appear to be functionally distinct. Sequence-specific binding to their target DNA sequences is mediated mainly by the C-terminal WRKY domain. The function of the N-terminal WRKY domain remains unclear.
Figure 1. The consensus WRKY domain for each WRKY group in higher plants. The WRKY motif, the cysteines, and histidines that form the zinc finger are shown in boxes. The 4 β-strands are shown with arrows. I CT and I NT denote the N-terminal and C-terminal WRKY domains from group I WRKY proteins.
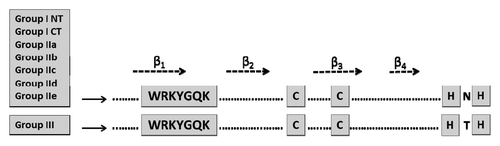
WRKY1 is involved in the SA signaling pathway and partially dependent on NPR1. The crystal structure of the C-terminal part of WRKY1 determined at 1.6 A resolution revealed that this domain is composed of a globular structure with 5 β strands, forming an antiparallel β-sheet. A zinc-binding site is situated at one end of the β-sheet, between strands β4 and β5. Based on this high-resolution crystal structure and site-directed mutagenesis, it was shown that the DNA-binding residues of WRKY1 are located at β2 and β3 strands.Citation49 Ciolkowski et al.Citation50 tested 5 WRKY TF members for their DNA-binding selectivity toward variants of the W-box embedded in neighboring DNA sequences. Their studies demonstrate differences in their binding site preferences which are partially depending on adjacent DNA sequences outside of the W-box core motif. The authors also showed that amino acid substitutions within the DNA-binding region of WRKY11 strongly impinge on its DNA binding activity. Therefore, in spite of the simple binding sequence known for WRKY protein-DNA recognition, more detailed information is necessary to understand the (binding) specificities of the WRKY family members, which might explain the diverse functions of the individual WRKY members.
Origin of WRKY TFs
Besides Arabidopsis, WRKY TFs have been studied in rice, tobacco, oat, cucumber, moss, and other plant species. Further, WRKY-like genes were identified in protists (Giardia lamblia and Dictyostelium discoideum) and green algae such as Chlamydomonas reinhardtii, demonstrating the ancient origin of the gene family. The phylogenetic trees generated from the WRKY domains indicate that the WRKY gene family arose during evolution through gene duplications and that the dramatic amplification of group III WRKY genes in rice is due to tandem and segmental gene duplications, which occurred to a lesser extent in Arabidopsis.Citation1,Citation2,Citation24 Some of the rice WRKY genes in group III are evolutionarily more active than those in Arabidopsis, and may have specific roles in monocotyledonous plants. Wang et al.Citation51 showed that purifying selection played a major role during the evolution of this family. Sites relaxed from purifying selection were identified and mapped onto the structural and functional regions of the WRKY1 protein. These analyses will enhance the understanding of the precise role of natural selection to create the functional diversity in WRKY family.Citation51 More information is required to understand the evolutionary constrains on WRKY gene evolution, and how the functional diversity of the WRKY protein family members can be explained.
Functions of WRKY TFs
Plants devote a large portion of their genome capacity to transcription, with the Arabidopsis and rice genome coding for more than 2100 and 2300 TFs, respectively.Citation52 The WRKY gene family has been suggested to play important roles in the regulation of transcriptional reprogramming associated with plant stress responses. Being unable to move, plants encounter numerous biotic and abiotic stresses at different developmental stages which include drought, salinity, temperature changes from freezing to scorching, decreased availability of essential nutrients resulting in nutrient starvation and variable light conditions. To overcome these unfavorable conditions, plants have developed various intricate mechanisms which occur at multiple levels. Recognition of stress cues and transduction of the signals to activate adaptive responses and regulation of stress-related genes are the key steps leading to plant stress tolerance.Citation53 Induction of stress-related genes occurs mainly at the transcriptional level, and modification of the temporal and spatial expression patterns of specific stress-related genes is an important part of the plant stress response.Citation54 Interestingly, the strong involvement of the plant-specific WRKY TF family in the stress responses demonstrates that plant developed their own way to deal with these challenges.
In general stress signals are perceived by membrane-bound or soluble receptors and/or activate phytohormone-dependent processes. A large number of information demonstrates the involvement of WRKYs in receptor-mediated processes. In Arabidopsis, small peptides encoded by PROPEP genes act as damage-associated molecular patterns that are perceived by 2 leucine-rich repeat receptor kinases, PEPR1 and PEPR2, to amplify defense responses. WRKY33 binds to the promoter of the 2 receptor kinase genes in a stimulus-dependent manner and regulates their expression.Citation55 In addition, besides NB-LRR type R proteins, receptor-like protein (RLP) members participate in plant immunity. Zhang et al.Citation56 have demonstrated that WRKY70 functions downstream of SNC2–1D (suppressor of rpr1–1, constitutive 2), a RLP member. In barley, intracellular milder A (MLA) R proteins function in the nucleus and confer resistance against powdery mildrew fungus. Recognition of the avirulence A10 effector by MLA10 induces nuclear associations between the receptor and WRKY TFs.Citation57 These 3 examples demonstrate the complexity in this scenario, and the involvement of WRKYs at different regulatory levels. Furthermore, the chloroplast-localized protein magnesium-protoporphyrin IX chelatase H subunit function as an ABA receptor (ABAR). ABAR spans the chloroplast envelope and the cytosolic C-terminus of ABAR interacts with WRKY18, WRKY40, and WRKY60, which function as negative regulators of ABA signaling in seed germination and postgermination growth.Citation58 Jiang et al.Citation59 have demonstrated that the Arabidopsis 3-ketoacyl-CoA-thiolase-2 (KAT2), an enzyme of fatty acid β-oxidation, is involved in ABA signaling. KAT2 functions downstream of WRKY40 and thus, WRKY40 may link KAT2 with the ABA-mediated signaling. Finally, herbirvory by Spodoptera littoralis activates the synthesis of JA-isoleucine which binds to a complex consisting of the receptor COI1 and JAZ repressors. This results in the activation of WRKY18 and WRKY40 genes.Citation60
WRKYs are substrates of calcium-dependent protein kinases (CPK). A point mutation in WRKY28 completely abolishes its phosphorylation by CPK4 and CPK11.Citation61 Calmodulin (CaM) is a ubiquitous Ca2+-binding protein known to regulate diverse cellular functions by modulating the activity of various target proteins. Park et al.Citation62 isolated a cDNA encoding WRKY7 as a CaM-binding TF from an Arabidopsis expression library with horseradish peroxidase-conjugated CaM. CaM binds specifically to the Ca2+-dependent CaM-binding domain (CaMBD) of WRKY7, as shown by site-directed mutagenesis, a gel mobility shift assay, a split-ubiquitin assay, and a competition assay using a Ca2+/CaM-dependent enzyme. Furthermore, the CaMBD of WRKY7 is a conserved structural motif (C-motif) found in group IId of the WRKY protein family.
Phosphorylation also plays important roles in WRKY processes. Besides phosphorylation of WRKY28 by CPK4 and CPK11,Citation61 MAP kinase kinase kinase (MEKK) 1 phosphorylates WRKY53 in vitro and phosphorylation increases DNA binding and transcription of a WRKY53-driven promoter construct in vivo. 63Phosphorylation of WRKY33 by 2 pathogen-responsive MAPKs drives phytoalexin biosynthesis in Arabidopsis.Citation64 Xie et al.Citation65 demonstrated that PKS2 (SOS2-like protein kinase 5) phosphorylates NPR1 at its C-terminal region which results in the upregulation of WRKY38 and WRKY62. Ueno et al.Citation66 showed that MAP kinases phosphorylate rice WRKY45. Phosphorylation of the AD protein (a protein with similarities to HPT kinases) increases its binding to the WRKY53 promoter. These examples demonstrate that phosphorylation is an essential signaling event at, up- and downstream of WRKY TFs and the expression of their genes.
A number of reports points to the importance of epigenetic control of WRKY functions. Regulation of leaf senescence includes epigenetic re-programming via histone methylation at WRKY53.Citation67 Also the seed size is controlled by epigenetic and genetic programs which include WRKYs.Citation43 Furthermore, epigenetics controls plant immunity.Citation68 Histone deacetylases are involved in the activation of JA/ET-sensitive defense mechanisms. ATP-dependent chromatin remodelers mediate the constitutive repression of the SA-dependent pathway whereas histone methylation at the WRKY40 promoter affects the activation of this pathway. Interestingly, bacterial infected tissues show a net reduction in DNA methylation, which may affect the disease resistance genes responsible for the surveillance against pathogens. As some epigenetic marks can be erased or maintained and transmitted to offspring, epigenetic mechanisms may provide plasticity for the dynamic control of emerging pathogens without the generation of genetic lesions.Citation68 The expression of WRKY70 is known to be antagonistically regulated by the SA and JA signaling pathways. Thus, this gene encodes a TF functioning at the crossroad of the 2 pathways. The Arabidopsis homolog of Trithorax, ATX1 activates the expression of WRKY70 and is involved in establishing the trimethylation pattern of histone H3 tail lysine 4 (H3K4me3) residues of its nucleosomes. Chromatin immunoprecipitation analyses demonstrated that WRKY70 is a primary target for the ATX1 histone methylase activity, while the SA-responsive gene, PR1, and the JA-responsive gene, THI2.1, are secondary targets. The finding that PR1 and THI2.1 nucleosomes carry H3K4me3-marks unrelated to their transcription states suggests that the defense-response genes PR1 and THI2.1 keep their nucleosomes in 'actively' modified state, perhaps, in preparation for quick-changes of transcription when needed by the cell. Alvarez-Venegas et al.Citation69 proposed that a single epigenetic factor can thereby orchestrate expression of a large number of genes.
Abiotic Stress
Compared with the research on biotic stress, little is known about the involvement of these TFs in abiotic stress responses. The WRKY gene family is induced in response to different abiotic stresses and emerged as an important candidate for imparting stress tolerance. The tight regulation and fine-tuning of WRKY proteins during plant stress responses contribute to the establishment of complex signaling webs and the important roles of WRKY proteins in plant abiotic stress responses make them potential candidates for imparting stress tolerance. Recent studies have demonstrated that many WRKY genes are strongly and rapidly induced in response to certain abiotic stresses, such as wounding, drought or salinity, cold and heat or osmotic stress. A single WRKY protein is often involved in several stress responses, and some of them are even involved in abiotic and biotic stresses. In recent years, numerous groups have demonstrated that manipulation of WRKY TF levels in knockout or overexpressor plants affects specific stress responses. Which stress response is associated with a particular WRKY is puzzling at present. It might be helpful in the future to perform comparative analyses of knockout and overexpressor lines under various stress conditions to pinpoint down the specific roles of the WRKYs in a given stress response.
Drought and Salt Stress
Environmental stresses such as drought and salinity do not only induce imbalances of ionic and osmotic homeostasis in the cell but also impair photosynthesis, cellular energy depletion, and the redox homeostasis. The involvement of WRKYs in regulatory systems that link sensing and signaling of environmental conditions to cellular adaptive responses is much broader than anticipated so far. WRKY TFs are considered as one of the master regulators for molecular reprogramming to enhance stress tolerance of plants. Several WRKYs are reported to be involved in drought and salt stress in Arabidopsis, rice,Citation19 and other plant species. Many established tolerance mechanisms to drought and salinity stress are related to ABA signaling.
Almost 2000 drought-responsive genes were identified in Arabidopsis under progressive soil drought stress,Citation33 and several of them code for WRKY TFs. Most of the drought-regulated genes recovered to normal expression levels within 3 h after rewatering. About 2/3 of the drought-responsive genes were also regulated by ABA and the ABA analog PBI425, and the list includes WRKY TF genes. Analysis of promoter motifs confirms that many of the drought-responsive genes are affected by ABA signaling. The endogenous ABA level and that of the ABA catabolites increased under drought stress and they recovered to normal levels 3 h after rehydration. Besides ABA, also other plant hormones such as JA, auxin, cytokinin, ET, brassinosteroids, and gibberellins were affected by the drought stress.Citation33 Furthermore, there is an extensive cross-talk between responses to drought and other environmental factors including light and biotic stresses, and drought stress affects the hormone homeostasis.Citation33 Zou et al.Citation70 investigated WRKY21 from the creosote bush Larrea tridentata, a xerophytic evergreen C3 shrub thriving in vast arid areas of North America. WRKY21 is highly expressed under drought stress and functions as an activator the ABA signaling pathway. Elevated expression of WRKY57 improved drought tolerance of Arabidopsis by elevation of ABA levels.Citation71 Transgenic Arabidopsis seedlings overexpressing the grapebine VvWRKY11 showed higher tolerance to water stress induced by mannitol. Thus, VvWRKY11 is involved in the response to dehydration stress.Citation72 Consistent with these observations expression of wild soybean WRKY20 in Arabidopsis enhances drought tolerance and regulates ABA signaling.Citation73 Compared with the wild type, GsWRKY20 overexpression lines were more sensitive to ABA in stomatal closure, and exhibited a greater tolerance to drought stress, a decreased water loss rate, and a decreased stomatal density. Babitha et al.Citation74 expressed the AtbHLH17 and AtWRKY28 TF genes which are known to be upregulated under drought and oxidative stress, in Arabidopsis. The transgenic lines exhibited enhanced tolerance to NaCl, mannitol, and oxidative stress. Under mannitol stress condition a higher root growth was observed in the transgenic lines. Several downstream target genes were upregulated under various stress conditions in the overexpressor lines and several of the responsive genes had either W-box sequences or bHLH cis elements in their promoter regions. These examples demonstrate that the WRKYs are powerful tools for the generation of drought-resistance plants.
Involvement of WRKY factors in plant salt adaptation was shown for WRKY25 and WRKY33 which increased salt tolerance and ABA sensitivity independently of the SOS-pathway when overexpressed in A. thaliana.Citation75 Similarly, overexpression of OsWRKY45 in rice resulted in enhanced salt and drought tolerance, in addition to increased disease resistance. GsWRKY20 from wild soybean was induced by ABA, salt, cold, and drought, suggesting an involvement of this TF in several abiotic stress-related responses. Chen et al.Citation22 analyzed the relationship of WRKY18, -40 and -60 in salt and biotic stress-exposed Arabidopsis seedlings. WRKY18 and WRKY60 have a positive effect on the plant´ ABA sensitivity for inhibition of seed germination and root growth. The same 2 WRKY genes also enhance plant sensitivity to salt and osmotic stress. WRKY40, on the other hand, antagonizes WRKY18 and WRKY60 in the effect on plant sensitivity to ABA and abiotic stress in germination and growth assays. Both WRKY18 and WRKY40 are rapidly induced by ABA, while induction of WRKY60 by ABA is delayed. ABA-inducible expression of WRKY60 is almost completely abolished in the wrky18 and wrky40 mutants. WRKY18 and WRKY40 recognize a cluster of W-box sequences in the WRKY60 promoter and activate WRKY60 expression in protoplasts. Thus, WRKY60 might be a direct target gene of WRKY18 and WRKY40 in ABA signaling. Using a stable transgenic reporter/effector system, both WRKY18 and WRKY60 act as weak transcriptional activators while WRKY40 is a transcriptional repressor in plant cells. These examples demonstrate that the 3 related WRKY TFs form a highly interacting regulatory network that modulates gene expression in both plant defense and stress responses by acting as either transcription activator or repressor.Citation22 Finally, Vanderauwera et al.Citation36 characterized WRKY15 which modulates plant growth and salt/osmotic stress responses in Arabidopsis. WRKY15-overexpressing plants linked an endoplasmic reticulum-to-nucleus communication to a disrupted mitochondrial stress response under salt-stress conditions. This example demonstrates that a WRKY15-mediated salt stress response may be integrated into a signaling network that includes communication between different cellular organelles. Van Aken et al.Citation76 showed that AtWRKY40 and AtWRKY63, which are involved in stress responses, modulate the expression of stress-responsive nuclear genes encoding mitochondrial and chloroplast proteins. This provides additional evidence for the involvement of WRKYs in a communication system between the organelles. The crosstalk between the organelles may be more pronounced or relevant when the plants are exposed to (salt) stress. This again reinforces that comparative studies with different wrky knockout and WRKY overexpressor lines might be helpful to understand the complete scenario.
Cold/Heat Stress
In Arabidopsis, 939 cold-regulated genes have been reported with 655 upregulated and 284 downregulated genes.Citation77 A large number of early cold-responsive genes encode TFs. Among them are 8 WRKY genes ().Citation77 In particular, the expression of 6 cold-inducible WRKY genes was enhanced in ice1,Citation77 a mutant with a lesion in a gene that is strongly induced under cold stress (Citation78 and references therein), and the altered gene expression occurred almost exclusively at early time points. This demonstrates that WRKYs are major early cold-regulated genes in Arabidopsis. Luo et al.Citation73 showed that wild soybean WRKY20 is cold inducible and enhances drought tolerance in Arabidopsis and regulates ABA signaling.
Table 1. Names of cold regulated WRKY genes with altered transcription in ice1
Mature pollen is very sensitive to cold stress in chilling-sensitive plants. Zou et al.Citation79 showed that WRKY34 is involved in pollen viability, although the exact mechanism is unclear. Cold treatment increased WRKY34 expression in the wild type, and promoter-GUS analysis revealed that WRKY34 expression is pollen-specific. Enhanced green fluorescent protein-tagged WRKY34 was localized in the nuclei. Pollen harboring the wrky34 allele showed higher viability than pollen with the WRKY34 allele after cold treatment. Further functional analysis indicated that WRKY34 was involved in pollen development regulated by the pollen-specific MIKC* class of MADS-domain TFs under cold stress, and cold-insensitivity of mature wrky34 pollen might be partly attributable to the enhanced expression of transcriptional activator CBFs in the mutants. Thus, the WRKY34 TF negatively mediates cold sensitivity of mature Arabidopsis pollen and might be involved in the CBF signal cascade in mature pollen. This suggests that additional work is required to completely understand the role of WRKYs in cold stress and cold stress adaptation ()
Arabidopsis WRKY39 provides a nice example for a TF that is induced by heat stress.Citation80 WRKY39 is a member of the group II WRKY proteins and responds to both abiotic and biotic stress. Heat-treated seeds and wrky39 knock-down mutants had increased susceptibility to heat stress, showing reduced germination, decreased survival, and elevated electrolyte leakage compared with wild-type plants. In contrast, WRKY39 overexpressing plants exhibited enhanced thermotolerance compared with wild-type plants.Citation80 Therefore, WRKYs are involved in both cold and heat acclimation of plants.
Phosphate Limitation
Phosphorus (P), an essential macronutrient required for plant growth and development, makes up about 0.2% of a plant’s dry weight. It is involved in regulation of key metabolic pathways as well as several enzymatic reactions in plants and animals. P is a crucial component of major organic molecules such as nucleic acids, ATP, and membrane phospholipids. It is present in soils in the form of inorganic phosphate (Pi), which has low availability and poor mobility. To cope with Pi limitations, plants have evolved complex adaptive responses that include morphological and physiological modifications to improve their acquisition, use, and remobilization of P.Citation81-Citation83 Among the molecular determinants of Pi stress responses, TFs are believed to play a critical role in regulating adaptive mechanisms. Several TFs have been reported which are induced during Pi deprivation like WRKY6, -42, and -75.Citation84-Citation86
Devaiah et al.Citation87 identified WRKY75 as an important component of the Pi stress responses. WRKY75 was found to be nuclear localized and induced differentially in the plant during Pi deficiency. Suppression of WRKY75 expression through RNAi silencing resulted in early accumulation of anthocyanin, indicating that the RNAi plants were more susceptible to Pi stress.Citation85 WRKY75 is a positive regulator of several Pi starvation-induced genes including phosphatase, Mt4/TPS1-like genes and high affinity Pi transporters.Citation86
Chen et al.Citation84 studied the role of WRKY6 under low Pi stress conditions. WRKY6 is involved in Arabidopsis responses to low-Pi stress through regulating PHOSPHATE1 (PHO1) expression. PHO1 plays an important role in Pi translocation from root to shoot.Citation87 PHO1 is predominantly expressed in the stellar cells of the root and the lower part of the hypocotyls.Citation87 Low Pi treatment reduced WRKY6 binding to the PHO1 promoter, which indicates that PHO1 regulation by WRKY6 is Pi dependent and that low Pi treatment may release inhibition of PHO1 expression.Citation84 These studies provide important information about the link of WRKYs to nutrient-limitation stress. It remains to be tested whether these responses are specific for Pi, and whether comparable regulatory circuits exist for other essential plant nutrients. Interestingly, although WRKY75 and WRKY6 participate in the Pi-deficiency response, they regulated different target genes. Whether they function in different and independent signaling networks or whether they are connected to each other remains to be determined.
Sugar Sensing and Metabolism
WRKY45 and WRKY65 are involved in regulating genes which respond to carbon starvation.Citation88 Three rice WRKY genes are also upregulated in sucrose-starved rice suspension cultures.Citation89 Interestingly, an Arabidopsis line overexpressing the rice WRKY72 gene showed increased sensitivity of sugar starvation stress.Citation11 Hammargren et al.Citation90 showed that sugar regulates the expression of the Arabidopsis NUCLEOSIDE DIPHSOPHATE KINASE 3a (NDPK3a) gene. NDPK3a is located in mitochondria. Sugar metabolism is intricately connected with mitochondria through the conversion of sugars to ATP, and through the production of carbon skeletons that can be used in anabolic processes. Sugar molecules also take part in signaling cascades. An analysis of the NDPK3a promoter identified 2 boxes that have previously been reported to be involved in sugar signaling in barley by binding to the SUSIBA2 protein. SUSIBA2 is a WRKY TF. T-DNA insertions in WRKY4 and WRKY34 showed a possible involvement of WRKY TFs in sugar induction of NDPK3a. Sugar is also reported to be involved in retrograde signaling to the nucleus.Citation91,Citation92 Therefore, this regulatory circuit may contribute to couple retrograde signaling to sugar metabolism. Furthermore, a long-standing discussion suggests a link between Pi availability and sugar metabolism in beneficial symbiotic interactions. Since WRKYs are involved in both processes and integrate information from the different organelles involved in sugar metabolism, they might as well have a more general regulatory role in coordinating the cellular metabolism.
Oxidative Stress
Since WRKY TFs are involved in various stress responses, it is not surprising that they also play an important role in oxidative stress. Expression of several WRKY genes is upregulated in response to ROS or ROS-generating stimuli, imbalances in redox homeostasis, as well as endogenous ROS-dependent developmental programs such as senescence.Citation3,Citation93-Citation96 At least 8 Arabidopsis WRKY genes (WRKY30, -75, -48, -39, -6, -, -22, -53, -8) have been demonstrated to be upregulated in response to H2O2 treatments.Citation22,Citation96-Citation98 Overexpression of several WRKY genes which are upregulated in response to ROS-generating abiotic stresses confer also stress tolerance to plants.
WRKY25 expression is controlled by the transcriptional regulator Zat12, and Zat12 expression, in turn, is transcriptionally enhanced during osmotic, drought, salinity, temperature, oxidative or high-light stress and wounding.Citation99,Citation100WRKY28 is known to be upregulated under drought and oxidative stress. Babitha et al.Citation74 showed that Arabidopsis lines overexpressing the 2 TFs HLH17 and WRKY28 exhibited enhanced tolerance to NaCl, mannitol and oxidative stress. Under mannitol stress higher root growth was observed in these transgenic lines. The performance of the overexpressor lines was also better than the wild-type when they were exposed to gradual long-term desiccation stress. Genes having W-box elements in their promoters showed higher expression levels in the overexpressor lines than in the wild type under oxidative stress conditions. Hence, simultaneous overexpression of the 2 TFs HLH17 and WRKY28 results in upregulation of many downstream target genes and substantially improves the oxidative stress tolerance of the plants.
Scarpeci et al.Citation101 exposed plants to methyl viologen (MV), a superoxide anion propagator in the light, and identified superoxide-responsive genes with unknown function, for TFs and signal transduction components. The W-box sequence was present in the promoters of a number of genes which responded to superoxide. Band shift assays showed that oxidative treatments enhanced the specific binding of leaf protein extracts to this motif. In addition, a GUS reporter gene fused to the WRKY30 promoter, which contains the W-box motif, was induced by MV and H2O2. Thus, WRKY TF genes are rapidly activated by superoxide anion and may function as part of the early antioxidant response in Arabidopsis leaves.
Stress also causes perturbation of the ROS homeostasis.Citation36 The ROS-inducible Arabidopsis WRKY15 TF gene modulates plant growth and salt/osmotic stress responses. WRKY15 functions as negative regulator of salt- and osmotic-stress tolerance in Arabidopsis thaliana.Citation36 Overexpression of WRKY15 results in an increased leaf area and a 15–25% increase in plant biomass. Since the cell number did not increase, WRKY15 stimulates cell expansion, and this is caused by an intensified endoreplication. Transgenic plants expression artificial microRNA constructs targeting WRKY15 were smaller than wild-type plants, and contained a decreased leaf cell area.Citation36 Plants with elevated WRKY15 mRNA levels show also increased sensitivity to osmotic and oxidative stress. Expression profiling indicated that the majority of the regulated genes in stress-exposed WRKY15 overexpressors code for mitochondrial proteins, and they are members of the so-called mitochondrial dysfunction regulon.Citation36,Citation102,Citation103 These genes are likely to participate in the mitochondria-to-nucleus retrograde signaling.Citation36 The authors proposed that WRKY15 might function as a general repressor of genes involved in mitochrondiral retrograde signaling. When exposed to stress, WRKY15 might be released from its cognate W-box elements and replaced by other WRKY TFs. In such a model, WRKYs might act in a network of mutually competing participants with temporal replacement by other WRKY members in a stimulus-dependent manner.Citation3,Citation36,Citation104
Hormones such as ABA, the stress-responsive JA/ET and SA but also auxin are often coupled to ROS production and alterations in redox homeostasis. Wildermuth and JonesCitation40 showed that root growth is inhibited at 10–100 μM SA, concentrations that may be of physiological relevance in endogenous stress signaling and responses to rhizosphere SA. This SA root growth inhibition results primarily from a dramatic reduction in cell elongation and is specific to SA. Structurally similar molecules such as 4-hydroxybenzoate do not inhibit root growth at these concentrations. Root cell elongation is associated with ROS accumulation and auxin signaling. Through the use of ROS specific dyes and auxin reporters, Wildermuth and JonesCitation40 showed that root-growth inhibitory SA levels eliminate certain ROS maxima and alter auxin signaling in growing root tips. They further show through analysis of SA signaling mutants that SA-mediated inhibition of root growth does not require the well-established NPR1-SA immune response pathway. Rather, WRKY38, a highly SA responsive TF known to be expressed in the root and to modulate the NPR1 pathway, regulates the SA response. Thus, exogenous SA inhibits root cell elongation, abolishes certain root ROS maxima and alters root auxin signaling, and the SA response involves WRKY38. Another example provides an enzyme catalyzing β-oxidation of fatty acids, 3-ketoacyl-CoA thiolase-2 (KAT2).Citation59 KAT2 positively regulates ABA signaling in all the major ABA responses, including ABA-induced inhibition of seed germination and post-germination growth arrest, and ABA-induced stomatal closure and stomatal opening inhibition in Arabidopsis. KAT2 was shown to be important for ROS production in response to ABA, suggesting that KAT2 regulates ABA signaling at least partly through modulating ROS homeostasis. KAT2 may function downstream of WRKY40, and thus WRKY40 may link KAT2 with the ABA receptor ABAR/CHLH-mediated signaling.Citation59 Since several WRKYs such as WRKY40 are involved in abiotic and biotic stress responses which induce ROS species or causes imbalances in ROS homeostasis, it remains to be studied whether ROS are the immediate triggers that regulate WRKY gene expression.
Biotic stress responses involve ROS production mainly via plasma-membrane located NADPH oxidases. Exposure of plants to pathogens or PAMPs results in ROS production and the activation of WRKY genes involved in innate immunity, such as WRKY18, -40, and -70. It is generally believed that PAMP-induced receptor activation induces a short signal transduction pathway that activates the plasmamembrane-located NADPH oxidase to produce H2O2 in the apoplastic space. Parallel signaling pathways, e.g., via MAP kinase phosphorylation, activate WRKY TFs to induce defense genes. Whether and how these 2 signaling events are connected to each other is unknown. Göhre et al.Citation105 showed a molecular crosstalk between PAMP-triggered immunity and photosynthesis. Activation of defense by PAMPs leads to a rapid decrease in nonphotochemical quenching (NPQ). Conversely, NPQ also influences several responses of PAMP-triggered immunity. In a mutant impaired in NPQ, apoplastic ROS production is enhanced and defense gene expression is differentially affected. Although induction of the early defense markers WRKY22 and WRKY29 is enhanced, induction of the late markers PR1 and PR5 is completely abolished. Therefore, regulation of NPQ is an intrinsic component of the plant's defense program and affects also WRKY gene expression. Gao et al.Citation106 showed that WRKY50 and WRKY51 mediate SA- and low- oleic acid (18:1)-dependent repression of JA signaling. Signaling induced upon a reduction in oleic acid levels simultaneously upregulates SA-mediated responses and inhibits JA-inducible defenses, resulting in enhanced resistance to biotrophs but increased susceptibility to necrotrophs. Knockout mutations in WRKY50 and WRKY51 lowered SA levels. Inactivation of the WRKY genes in the ssi2 (suppressor of SA insensitivity2) background (ssi2 wrky50 and ssi2 wrky51) resulted in plants which contained high ROS levels. Thus, the 3 genes participate in maintaining a balanced ROS homeostasis in the plant under stress.
Besides pathogenic plant/microbe interactions, WRKYs also participate in beneficial symbiosis. Brotman et al.Citation107 reported that during early phases of root colonization by the opportunistic plant symbionts Tricoderma spp., expression of several genes related to osmo-protection and general oxidative stress in roots are upregulated by the fungi. Trichoderma can ameliorate plant growth under conditions of abiotic stress by lowering ameliorating increases in ET levels as well as promoting an elevated antioxidative capacity.
The relationship between ROS produced in response to biotic and abiotic stresses for WRKY expression needs to be investigated. In addition, the spatial distribution of the ROS species, which regulated WRKY genes, need to be analyzed. These studies may contribute to the understanding of the specificity in WRKY functions.
Another intensively studied field is the relationship of WRKYs and ROS during senescence. WRKY53 and WRKY70 are implicated as positive and negative regulators of senescence, respectively. Besseau et al.Citation108 showed that 2 WRKYs, WRKY54 and WRKY30, are involved in this process. The structurally related WRKY54 and WRKY70 exhibit a similar expression pattern during leaf development and appear to have co-operative and partly redundant functions in senescence, as revealed by single and double mutant studies. These 2 negative senescence regulators and the positive regulator WRKY53 interact independently with WRKY30, as shown by yeast 2-hydrid analysis. WRKY30 was expressed during developmental leaf senescence and could be part of a senescence regulatory network with the other WRKYs. Expression in wild-type and SA-deficient mutants suggests a common but not exclusive role for SA in induction of WRKY30, -53, -54, and -70 during senescence. WRKY30 and WRKY53 but not WRKY54 and WRKY70 are also responsive to additional signals such as ROS, which accumulate during senescence and control senescence progression. The results suggest that WRKY53, WRKY54, and WRKY70 may participate in a regulatory network that integrates internal and environmental cues to modulate the onset and the progression of leaf senescence, possibly through an interaction with WRKY30. Rosenvasser et al.Citation109 reported on the induction of WRKY6 in the leaves of Pelargonium cuttings during dark-induced senescence. Besides an increase in the ROS level, the senescence-associated protease homolog genes PeSAG12–1 and PeWRKY6–1 were upregulated during senescence.Citation109 It is not clear at present how these 2 processes are related to each other.
Taken together, a number of independent reports demonstrate that alterations in ROS levels, either in response to biotic or abiotic stress signals or caused by developmental processes, induce various WRKY genes. It remains to be determined how the stress-induced signaling pathways are related to the altered ROS levels in inducing WRKY gene expression. Furthermore, it is also not clear whether the stress stimuli can be replaced by ROS in all instances. ROS with signaling functions are H2O2, singlet oxygen (1O2), hydroxyl radical (OH·) and superoxide anion radical (O2.-) and a given ROS and its location affect its role in signaling.Citation11,Citation94,Citation111,Citation112 Whether all of these ROS species induce WRKYs, whether the cellular location—in which the ROS species are produced—is important, and whether there are differences in the response of the individual WRKYs, remains to be studied as well.
Biotic Stress/Wounding
WRKY TFs play an important role in the regulation of early defense-responses associated with pathogen defense. First, pathogen infection or treatment with pathogen elicitors or SA has been shown to induce rapid expression of WRKY genes from a number of plants.Citation104,Citation113-Citation117 Second, a number of defense-related genes, including the PR genes, contain W-box elements in their promoter regions and these W-box sequences are specifically recognized by WRKY proteins and are necessary for the inducible expression of these genes.Citation104,Citation113-Citation117 Several studies have provided direct evidence for the involvement of specific WRKY proteins in plant defense responses. For example, WRKY1 has been shown as a transcriptional activator mediating fungal elicitor-induced gene expression by binding to W box elements in parsley. In situ RNA hybridization revealed that WRKY1 is rapidly and locally activated in parsley leaf tissue around fungal infection sites. Transient expression studies in parsley protoplasts showed that a specific arrangement of W box elements in the WRKY1 promoter itself is necessary and sufficient for early activation and that WRKY1 binds to such elements.Citation118 Similarly, WRKY22 and WRKY29 have been shown to be induced by the MAPK pathway involved in plant responses to both bacterial and fungal pathogens, and elevated WRKY29 expression in transiently transformed leaves led to reduced disease symptoms.Citation118
WRKY18, WRKY40, and WRKY60 have been intensively studied and they were shown to be induced in response to biotrophic, hemibiotrophic and necrotrophic fungi.Citation20 Their roles in plant defense against microbial pathogen have been analyzed with various knockout mutant combinations and with constitutive overexpression lines, e.g., after infection with the bacterial pathogen Pseudomonas syringae or the fungal pathogen B. cinerea. Among the 3 single knockout mutants analyzed, only wrky18 exhibited a small reduction in bacterial growth, while wrky40 and wrky60 displayed no significant phenotype after infection of the bacterial pathogen. Two double knockout mutants, wrky18 wrky40 and wrky18 wrky60, on the other hand, supported modestly reduced bacterial growth, while the wrky18 wrky40 wrky60 triple knockout mutant had the most reduced bacterial growth when compared with wild-type plants. Constitutive overexpression of WRKY18 led to constitutive expression of the SA-related PR1 gene and enhanced resistance to P. syringae, its co-expression with WRKY40 or WRKY60 resulted in increased growth of the bacterial pathogen. These examples indicate that WRKY proteins can function as positive regulators of plant defense responses and disease resistance. Other examples for the involvement of WRKYs in innate immunity have been intensively described in various recent reviews.Citation6-Citation8,Citation13,Citation16
WRKys also participate in other stress responses such as UV-B stressCitation119 and wounding.Citation22,Citation115 Nicotiana attenuata WRKY3 and WRKY6 responded to wounding, WRKY6 is required for WRK6 elicitation by fatty acid-amino acid conjugates from the larval oral secretions that are released into the ounds during feeding.Citation120 Silencing of both genes makes plants highly vunerable to herbivores and reduce Manduca sexta-elicited JA and JA-isoleucine levels.
Beneficial Plant/Microbe Interactions
The establishment of arbuscular mycorrhizal associations causes major changes in the root transcriptome, induced during the pre-, early, and late stages of root colonization. The most important class of regulated genes during the pre-stage of root colonization was associated to plant stress and in particular to the WRKY TF genes. Apparently, arbuscular mycorrhizal establishment requires a mild activation of defense responses in which WRKY TFs are involved.Citation47 Gallou et al.Citation47 observed 9 WRKY genes which are upregulated during the pre-stage of potato root colonization with Glomus interadices while only one WRKY gene was upregulation at late stages of colonization. No regulation of WRKY is observed at early stage of root colonization. These results suggest an involvement of WRKY genes during the pre-stage of root colonization. It would be interesting to known whether this stage is altered when potato line are used in which the corresponding WRKY genes are silenced or downregulated.
Brotman et al.Citation107 showed that the plant symbionts Trichoderma spp. colonize the apoplast of plant roots. Microarrays analysis of Arabidopsis roots demonstrated a transient repression of the plant immune responses supposedly to allow root colonization. Enhancement in the expression of WRKY18 and WRKY40, which stimulate JA-signaling via suppression of JAZ repressors and negatively regulate the expression of the defense genes FMO1, PAD3, and CYP71A13, was detected in Arabidopsis roots upon Trichoderma colonization and reduced root colonization was observed in the wrky18 wrky40 double mutant line, while partial phenotypic complementation was achieved by overexpressing WRKY40 in the wrky18 wrky40 background. On the other hand, increased colonization rate was found in roots of the fmo1 knockout mutant. Trichoderma spp. stimulates plant growth and resistance to a wide range of adverse environmental conditions. Arabidopsis and cucumber (Cucumis sativus L.) plants treated with Trichoderma prior to salt stress imposition show significantly improved seed germination. In addition, Trichoderma treatment affects the expression of several genes related to osmo-protection and general oxidative stress in roots of both plants. The MDAR gene for monodehydroascorbate reductase is significantly upregulated and, accordingly, the pool of reduced ascorbic acid was found to be increased in Trichoderma-treated plants. 1-Aminocyclopropane-1-carboxylate (ACC)-deaminase silenced Trichoderma mutants were less effective in providing tolerance to salt stress, suggesting that Trichoderma, similarly to ACC deaminase producing bacteria, can ameliorate plant growth under conditions of abiotic stress, by lowering ameliorating increases in ET levels as well as promoting an elevated antioxidative capacity. Much more work is required to understand the role of WRKYs in beneficial plant/microbe interactions, in particular, since beneficial microbes promote nutrient uptake under nutrient-limiting conditions, control sugar metabolism and establish systemic resistance responses, all processes in which WRKYs are involved as well.Citation39,Citation84-Citation86,Citation90,Citation121,Citation122
WRKY and Hormone Signaling
Many WRKYs participate in different hormone signaling pathways or their genes are regulated in response to these phytorhormones. For instance, WRKY18, -40 and -60 participate in JA, SA, and ABA signaling.
Role in ABA Signaling
WRKY genes are activators of ABA signaling, a phytohromone which mediates plant responses to abiotic stress.Citation1 Rushton et al.Citation123 showed that WRKYs act as positive regulators of ABA-mediated stomatal closure and hence drought responses and conversely, many WRKYs are negative regulators of seed germination. In Arabidopsis, WRKYs appear to act downstream of at least 2 ABA receptors: the cytoplasmic PYR ⁄ PYL ⁄ RCAR protein phosphatase 2C-ABA complex and the chloroplast envelope–located ABAR–ABA complex. In vivo and in vitro promoter-binding studies show that the target genes of WRKYs which are involved in ABA signaling include well-known ABA-responsive genes such as ABF2, ABF4, ABI4, ABI5, MYB2, DREB1a, DREB2a, RAB18. Some well-characterized stress-inducible proteins such as RD29A and COR47 are also found in signaling pathways downstream of WRKY TFs.Citation123 ABA signaling is also connected to other phytohromones, but their connetions to WRKYs is less understood. The ArabidopsisWRKY18, -40 and -60 represent nodes in Arabidopsis signaling networks that become activated by numerous stimuli and may integrate signaling from numerous phytohormones including SA, JA, ABA, and gibberellin acid (GA).
SA Signaling and WRKYs
The Arabidopsis WRKY1 was shown to be involved in SA signaling and is partially dependent on NPR1.Citation49 Also the heat stress-induced WRKY39 is induced in response to treatment with SA or methyljasmonate and positively regulates the cooperation between the SA- and JA-activated signaling pathways that mediate responses to heat stress.Citation80 Kim et al.Citation124 showed that WRKY38 and WRKY62, encoding 2 structurally similar type III WRKY TFs, are induced in a NPR1-dependent manner by SA or by virulent P. syringae. Disease resistance and SA-regulated PR1 gene expression are enhanced in the wrky38 and wrky62 single mutants and, to a greater extent, in the double mutants. Overexpression of WRKY38 or WRKY62 reduces disease resistance and PR1 expression. Thus, WRKY38 and WRKY62 function additively as negative regulators of plant basal defense. Rapid pathogen-induced WRKY33 expression does not require SA signaling, but is dependent on PAD4, a key regulator upstream of SA.Citation125 WRKY33 is essential for defense toward the necrotrophic fungus Botrytis cinerea.Citation126 Loss of WRKY33 function results in inappropriate activation of the SA-related host response and elevated SA levels post infection and in the downregulation of JA-associated responses at later stages. This downregulation appears to involve direct activation of several JA ZIM-domain genes, encoding repressors of the JA-response pathway, by loss of WRKY33 function and by additional SA-dependent WRKY factors. Moreover, genes involved in redox homeostasis, SA signaling, ET-JA-mediated cross-communication, and camalexin biosynthesis were identified as direct targets of WRKY33. Although SA-mediated repression of the JA pathway may contribute to the susceptibility of wrky33 plants to B. cinerea, it is insufficient for WRKY33-mediated resistance. Thus, WRKY33 apparently directly targets other still unidentified components that are also critical for establishing full resistance toward this necrotroph.Citation126,Citation127 Likewise, the expression profile of VvWRKY11 from grapevine in response to treatment with SA or the pathogen Plasmopara viticola is rapid and transient.Citation72 Transgenic Arabidopsis seedlings overexpressing VvWRKY11 showed higher tolerance to water stress induced by mannitol than wild-type plants. These results demonstrated that VvWRKY11 is involved in the response to dehydration stress.Citation72 The stress-induced WRKY25 functions as a negative regulator of SA-mediated defense responses to P. syringae.Citation128 This is consistent with the finding that WRKY25 is a substrate of Arabidopsis MAP kinase 4, a repressor of SA-dependent defense responses.Citation129 The authors showed that MPK4 functions as a regulator of pathogen defense responses, because it is required for both repression of SA-dependent resistance and for activation of JA-dependent defense gene expression. In yeast 2-hybrid experiments the MPK4 substrate MKS1 was isolated. Analyses of transgenic plants and genome-wide transcript profiling indicated that MKS1 is required for full SA-dependent resistance in mpk4 mutants, and that overexpression of MKS1 in wild-type plants is sufficient to activate SA-dependent resistance, but does not interfere with induction of a defense gene by JA. Further yeast 2-hybrid screening revealed that MKS1 interacts with WRKY25 and WRKY33. WRKY25 and WRKY33 were shown to be in vitro substrates of MPK4, and a wrky33 knockout mutant exhibited increased expression of the SA-related defense gene PR1. MKS1 may therefore contribute to MPK4-regulated defense activation by coupling the kinase to specific WRKY TFs.Citation129 Although these examples demonstrate the involvement of SA in WRKY functions, the role of SA and its integration into the kinase pathways is not completely clear.
Van Verk et al.Citation121 showed that increased defense against a variety of pathogens in plants is achieved through systemic acquired resistance (SAR). The broad-spectrum resistance brought about by SAR is mediated through SA. An important step in SA biosynthesis in Arabidopsis is the conversion of chorismate to isochorismate through the action of isochorismate synthase, encoded by the ICS1 gene. Also AVRPPHB SUSCEPTIBLE 3 (PBS3) plays an important role in SA metabolism, as pbs3 mutants accumulate drastically reduced levels of SA-glucoside, a putative storage form of SA. The ICS1 and PBS3 genes were highly expressed in protoplasts overexpressing WRKY28 or WRKY46, respectively. Electrophoretic mobility shift assays identified potential WRKY28 binding sites in the ICS1 promoter, positioned -445 and -460 base pairs upstream of the transcription start site. Mutation of these sites in protoplast transactivation assays showed that these binding sites are functionally important for activation of the ICS1 promoter. Chromatin immunoprecipitation assays with haemagglutinin-epitope-tagged WRKY28 showed that the region of the ICS1 promoter containing the binding sites at -445 and -460 was highly enriched in the immunoprecipitated DNA. These results demonstrate that WRKY28 and WRKY46 are transcriptional activators of ICS1 and PBS3, respectively.Citation121 Recently, Schön et al.Citation130 demonstrated that simultaneous mutation of WRKY18 and WRKY40 renders otherwise susceptible wild-type Arabidopsis plants resistant toward the biotrophic powdery mildew fungus Golovinomyces orontii. Resistance in wrky18 wrky40 double mutant plants is accompanied by massive transcriptional reprogramming, imbalance in SA and JA signaling, altered ENHANCED DISEASE SUSCEPTIBILITY1 (EDS1) expression, and accumulation of the phytoalexin camalexin. Genetic analyses identified SA biosynthesis and EDS1 signaling as well as biosynthesis of the indole-glucosinolate 4MI3G as essential components required for loss-of-WRKY18 WRKY40-mediated resistance toward G. orontii. The analysis of wrky18 wrky40 pad3 mutant plants impaired in camalexin biosynthesis revealed an uncoupling of pre- from postinvasive resistance against G. orontii. Interestingly, WRKY18 and WRKY40 act as positive regulators in effector-triggered immunity, as the wrky18 wrky40 double mutant was found to be strongly susceptible toward the bacterial pathogen P. syringae DC3000 expressing the effector AvrRPS4 but not against other tested Pseudomonas strains. It appears that G. orontii depends on the function of WRKY18 and WRKY40 to successfully infect Arabidopsis wild-type plants while, in the interaction with P. syringae AvrRPS4, they are required to mediate effector-triggered immunity.Citation130
WRKY45 plays also a major role in the SA-induced defense in rice.Citation131 The nuclear ubiquitin proteasome system (UPS) plays a role in regulating the function of WRKY45. Proteasome inhibitors induced accumulation of polyubiquitinated WRKY45 and transient upregulation of WRKY45 target genes in rice cells, suggesting that WRKY45 is constantly degraded by the UPS to suppress defense responses in the absence of defense signals. Mutational analysis of the nuclear localization signal indicated that UPS-dependent WRKY45 degradation occurs in the nuclei. Interestingly, transcriptional activity of WRKY45 after SA treatment was impaired by proteasome inhibition. The same C-terminal region in WRKY45 was essential for both transcriptional activity and UPS-dependent degradation. These results suggest that UPS regulation also plays a role in transcriptional activity of WRKY45.Citation131 Furthermore, knock-down of the rice WRKY45 severely reduces SA/benzothiadiazole (BTH)-induced resistance to the fungal pathogen Magnaporthe oryzae and the bacterial pathogen Xanthomonas oryzae pv oryzae. Conversely, overexpression of WRKY45 induces extremely strong resistance to both of these pathogens.Citation132 A detailed analysis of the transgenic rice plants uncovered a central role of WRKY45 in BTH-induced disease resistance. Furthermore, the SA-activated WRKY45 protein induces the accumulation of its own mRNA. Ueno et al.Citation66 showed that a MAPK cascade is involved in this regulation and phosphorylates WRKY45.
Panicle blast 1 (Pb1) is a panicle blast resistance gene derived from the indica rice cultivar “Modan.” Pb1 encodes a coiled-coil-nucleotide-binding site-leucine-rich repeat (CC-NB-LRR) protein and confers durable, broad-spectrum resistance to M. oryzae races. The Pb1 protein interacts with WRKY45. Pb1-mediated panicle blast resistance is largely compromised when WRKY45 was knocked down in a Pb1-containing rice cultivar. Leaf-blast resistance by Pb1 overexpression was also compromised in WRKY45 knockdown/Pb1 overexpressor rice. Blast infection induced higher accumulation of WRKY45 in the Pb1 overexpressor than in control Nipponbare rice. Overexpression of Pb1-Quad, a coiled-coil domain mutant that had weak interaction with WRKY45, resulted in significantly weaker blast resistance than that of wild-type Pb1. Overexpression of Pb1 with a nuclear export sequence failed to confer blast resistance to rice. These results suggest that the blast resistance of Pb1 depends on its interaction with WRKY45 in the nucleus. In a transient system using rice protoplasts, coexpression of Pb1 enhanced WRKY45 accumulation and increased WRKY45-dependent transactivation activity, suggesting that protection of WRKY45 from ubiquitin proteasome system degradation is possibly involved in Pb1-dependent blast resistance.Citation133 These studies highlight the central role of the rice WRKY45 in panicle blast resistance, and uncovered novel mechanisms of WRKY45 function.
NPR1 regulates PR gene expression by interacting with TGA TFs. NPR1 is essential in balancing the SA- and JA-dependent signal transduction pathways probably through direct or indirect interaction with some WRKY TFs and the NPR1-like protein NPR4. Different reports in different systems also describe an important role of JA in WRKY signaling and function: For instance, overexpression of VvWRKY1 in grapevines induces expression of JA pathway-related genes and confers higher tolerance to the downy mildew.Citation134 On the other hand, Rabara et al.Citation135 reported that dehydration-induced WRKY genes from tobacco and soybean respond to JA treatments in BY-2 cell culture. To fully understand the role of WRKYs in the SA-JA/ET crosstalk, more experients and different pathosystems need to be analyzed. It is also possible that no common scene for the differernt responses in the differernt pathosystems has been evolved.
Auxin and Cytokinin
Gradients of the plant hormone auxin, which depend on its active intercellular transport, are crucial for the maintenance of root meristematic activity. This directional transport is largely orchestrated by a complex interaction of specific influx and efflux carriers that mediate the auxin flow into and out of cells, respectively. Besides these transport proteins, plant-specific polyphenolic compounds known as flavonols have been shown to act as endogenous regulators of auxin transport. However, only limited information is available on how flavonol synthesis is developmentally regulated. Using reduction-of-function and overexpression approaches in parallel, Grunewald et al.Citation38 demonstrate that WRKY23 is needed for proper root growth and development by stimulating the local biosynthesis of flavonols. The expression of WRKY23 itself is controlled by auxin through the Auxin Response Factor 7 (ARF7) and ARF19 transcriptional response pathway. The authors proposed a model in which WRKY23 is part of a transcriptional feedback loop of auxin on its own transport through local regulation of flavonol biosynthesis.
Grunewald et al.Citation37 also analyzed the development of a multicellular embryo from a single zygote. In higher plants, apical-basal patterning mechanisms are crucial to correctly specify root and shoot stem cell niches that will sustain and drive post-embryonic plant growth and development. The auxin-responsive WRKY23 is expressed from early embryogenesis onwards and the timing and localization of its expression overlaps with the root stem cell niche. Knocking down WRKY23 transcript levels or expression of a dominant-negative WRKY23 version via a translational fusion with the SRDX repressor domain affected both apical-basal axis formation as well as installation of the root stem cell niche. WRKY23 expression is affected by 2 well-known root stem cell specification mechanisms, that is, SHORTROOT and MONOPTEROS-BODENLOS signaling. On the basis of these results, Grunewald et al.Citation37 postulated that a tightly controlled WRKY23 expression is involved in the regulation of both auxin-dependent and auxin-independent signaling pathways toward stem cell specification.
Xu et al.Citation39 analyzed Arabidopsis lines which overexpressed a cotton defense-related gene GbWRKY1. This resulted in modification of the root system by enhanced auxin sensitivity to positively regulate the Pi starvation response. GbWRKY1 from Gossypium barbadense was first identified as a defense-related gene and showed moderate similarity with AtWRKY75 from Arabidopsis. Overexpression of GbWRKY1 in Arabidopsis resulted in attenuated Pi starvation stress symptoms, including reduced accumulation of anthocyanin and impaired density of lateral roots in low Pi stress. Overexpression of GbWRKY1 caused plants constitutively exhibited Pi starvation response including increased development of lateral roots, relatively high level of total P and Pi, high expression level of some high-affinity Pi transporters and phosphatases as well as enhanced accumulation of acid phosphatases activity during Pi-sufficiency. It was speculated that GbWRKY1 may act as a positive regulator in the Pi starvation response as well as AtWRKY75. GbWRKY1 is probably involved in the modulation of Pi homeostasis and participates in the Pi allocation and remobilization but does not accumulate more Pi in Pi-deficient conditions, which was different from the fact that AtWRKY75 influenced the Pi status of the plant during Pi deprivation by increasing root surface area and accumulation of more Pi. The overexpression plants were more sensitive to auxin than wild-type and GbWRKY1 may partly influence the LPR1-dependent (low phosphate response 1) Pi starvation signaling pathway and was putatively independent of SUMO E3 ligase SIZ1 and PHR1 (phosphate starvation response 1) in response to Pi starvation. These examples demonstrate that GbWRKY1 has an influence on auxin-regulated root development as well as Pi acquisition.
Yu et al.Citation31 showed that plants can launch resistance mechanisms to stressful environments and acquire a new equilibrium between development and defense. To screen the rice WRKY TF which functions in abiotic stress tolerance and modulates the ABA response, 35S-OsWRKY72 transgenic Arabidopsis plants, whose seed germination was retarded under normal conditions, are more sensitive to mannitol, NaCl, ABA stresses and sugar starvation than vector plants. 35S-OsWRKY72 transgenic Arabidopsis displayed early flowering, reduced apical dominance, lost high temperature-induced hypocotyl elongation response, and enhanced gravitropism response. The expression patterns of 3 auxin-related genes AUX1, AXR1 and BUD1 were significantly altered in rosette leaves and inflorescences of 35S-OsWRKY72 plants, and 2 ABA-related genes ABA2 and ABI4 were induced in 35S-OsWRKY72 seedlings. These results suggest that OsWRKY72 interferes in the signal cross-talk between the ABA signal and auxin transport pathway in transgenic Arabidopsis.
Li et al.Citation30 performed a gene network analysis and functional studies of senescence-associated genes during Arabidopsis leaf senescence. Cytokinin, auxin, nitric oxide as well as Ca2+ delay leaf senescence. By contrast, ET, ABA, SA, and JA as well as oxygen promote leaf senescence. WRKY75 and the Cys2/His2-type TF AZF2 are positive regulators of leaf senescence and loss-of-function of WRKY75 or AZF2 delayed leaf senescence. Li et al.Citation30 also found that silencing of a protein phosphatase, AtMKP2, promoted early senescence. Apparently, WRKY75 couples senescence to a phytohromone network that includes auxin.
WRKYs Involved in Developmental Processes
Seed development and others
Several WRKY genes from different plant species are expressed during different stages of seed development. The WRKY gene DGE1 of orchardgrass (Dactylis glomerata) is expressed during somatic embryogenesis.Citation136 Similarly, ScWRKY1, a group Ia WRKY protein gene, is strongly and transiently expressed in fertilized ovules at the late torpedo stage in wild potato.Citation137 SUSIBA2 is expressed in the endosperm and regulates starch production. Arabidopsis WRKY10, also known as MINISEED3, is expressed in pollen and the globular embryo as well as in developing endosperm from the 2-nuclei stage through the cellularization stage.
WRKYs are found to be involved mainly in seed development. In Arabidopsis, Transparent Testa Glabra 2 (TTG2)/WRKY44 is the first member of the WRKY family with a role in trichome development. WRKY44 effects mucilage and tannin synthesis in the seed coat and also plays an important role in root hair development.Citation1,Citation2,Citation138 ScWRKY1 which is mainly expressed in leaves has also a specific role during embryogenesis.Citation137
Senescence
Leaf senescence is a complex developmental and highly regulated phase that involves both degenerative and nutrient recycling processes. It is characterized by loss of chlorophyll and the degradation of proteins, nucleic acids, lipids, and nutrient remobilization. WRKY TFs play a central role in the regulation of leaf senescence. Expression profiling in Arabidopsis revealed that WRKY TFs are the second largest family of TFs in reprogramming the Arabidopsis genome toward senescence.Citation139
Mutual regulation exists between WRKY22, WRKY6, WRKY53, and WRKY70.Citation140 WRKY22 transcription was suppressed by light and promoted by darkness. Expression of WRKY22 is strongly upregulated by H2O2. Dark-adapted WRKY22 overexpressor lines showed an accelerated and knockout line a delayed senescence phenotype, which was reflected in the regulation of senescence-associated genes.Citation140 This implies an involvement of WRKY22 in abiotic stress and senescence. WRKY22 can influence the expression of its own gene and of WRKY6, WRKY53, and WRKY70, suggesting the involvement of feedback regulatory loops.Citation140 A well-studied WRKY member involved in senescence, WRKY53, is tightly regulated by largely unknown mechanisms and involved in the cross-talk between senescence, biotic and abiotic stress responses.Citation5,Citation97 Expression of WRKY53 is induced by H2O2 and JA and repressed by SA.Citation97 Besides control of senescence, WRKY53 is involved in basal resistance against P. syringae, together with HSPRO2, a protein with similarities to a nematode resistance protein from sugar beet.Citation32 Furthermore, WRKY46 and WRKY70 together with WRKY53 co- ordinates basal resistance to P. synringae, and the 3 TFs appear to have overlapping and synergistic functions.Citation141 In a yeast-2-hybrid screen with WRKY53, Miao and ZentgrafCitation142 isolated the HECT domain E3 ubiquitin ligase UPL5. UPL5 interacts with WRKY53 via its leucine zipper domain, and was able to use WRKY53 as a substrate for polyubiquitination which led to its degradation. Expression of both genes is regulated antagonistically in response to H2O2, JA and plant development. upl5 knockout lines showed the same senescence phenotype as WRKY53 overexpressers. Overexpression of WRKY53 in the upl5 background enhanced the accelerated senescence phenotype of WRKY53 overexpressors. Therefore, UPL5 regulates leaf senescence in Arabidopsis through degradation of WRKY53 and ensures that senescence is executed in the correct time frame.Citation142 Regulation of leaf senescence in Arabidopsis includes epigenetic re-programming via histone methylation at WRKY53.Citation67 A mitogen activated protein kinase kinase kinase (MEKK1) was identified as a protein which binds to the WRKY53 promoter.Citation143 The binding is important for the switch of WRKY53 expression from a leaf age dependent to a systemic plant age dependent expression during bolting time. In addition to its promotor-binding activity, MEKK1 was also able to interact with the WRKY53 protein itself. Complex formation of MEKK1 and WRKY53 occurs predominately in the nucleus of Arabidopsis cells. MEKK1 phosphorylates WRKY53 in vitro and phosphorylation increases DNA-binding and transcription of a WRKY53 promoter driven reporter gene in vivo. These results suggest that MEKK1 is a bifunctional protein: it binds to the promoter of the WRKY53 gene regulating the switch from a leaf age dependent to a plant age dependent expression and it phosopharylates WRKY53 in vitro thereby increasing its DNA binding activity.Citation143 Furthermore, Miao et al.Citation63 identified a novel DNA-binding protein that contains a transcriptional activation domain and a kinase domain with similarities to HPT kinases, and the novel DNA-binding protein also binds to the upstream region of WRKY53. The activation domain protein (AD protein) of the DNA-binding protein can phosphorylate itself and phosphorylation increases its DNA-binding activity to the WRKY53 promoter region. The AD protein interacted also with MEKK1, however, the AD protein was not phosphorylated by MEKK1. Overexpression and knockout of the gene for this novel transcriptional regulator confirmed that the AD protein is a positive regulator of WRKY53 expression. Expression of the AD protein gene can be induced by H2O2 and is repressed by JA treatment, comparable to the regulation of WRKY53.Citation63 Interestingly, the AD protein is also found in plastids.Citation63 Another dual-targeted protein, Whirly1Citation144,Citation145 acts as a repressor of WRKY53 expression. Whirly1 mutants showed accelerated senescence and enhanced transcription of WRKY53 and of downstream genes WRKY33, SAG101, and SAG12. Transgenic Arabidopsis plants overexpressing Whirly1 targeting the protein either to the plastids or to the nucleus or to both compartments indicates that the nuclear isoform of Whirly1 can directly repress WRKY53 gene expression. Overexpressors where Whirly1 accumulates exclusively in plastids had an enhanced level of WRKY53 transcripts. This suggests that the nuclear form of Whirly1 is an upstream regulator of WRKY53 during leaf senescence,Citation140 and that the plastid form of Whirly1 is involved in retrograde signaling positively affecting the expression of WRKY53 in the nucleus.Citation146 More recently, Reusche et al.Citation147 have demonstrated that the width-inducing pathogen Verticillium longisporum triggers premature plant senescence for efficient host plant colonization. The soil-borne fungus enters the plant via the roots and colonizes the xylem. During later stages of the infection process, the fungal hyphae spread to senescence tissue in the leaves and switches from a biotrophic to a necrotrophic life style. The observed chlorosis induced by the fungus represents a premature senescence which is accompanied by the upregulation of WRKY53.Citation147 Taken together, regulation of WRKY53 appears to be a central process for reprogramming cells to senescence and the regulatory circuit is imbedded into MEKK1 signaling and retrograde signaling from plastids via the 2 dual-targeted AD protein and Whirly1.
Conclusion and Perspectives
WRKY proteins belong to a plant-specific family of TFs involved in various processes such as pathogen-induced defense programs, extensive metabolic changes associated with the establishment of defense responses or senescence, trichome development, etc. They participate in controlling the expression of a plethora of genes, by operating as positive and negative regulators and in various combinations. They can also be involved in unrelated stress responses; for example, WRKY18 and WRKY40 negatively regulate EDS1 signaling,Citation148 but positively regulates JA-signaling in response to G. orontii infection. WRKY25 and -33 are involved in biotic and abiotic stress responses; they function as negative regulators after P. syringae infection, but as positive regulators in conferring resistance against NaCl, cold, and heat and oxygenic stress.Citation75,Citation128,Citation149,Citation150 One WRKY protein seems to regulate several plant processes at a time and the mechanisms of regulation is not yet clear. Extensive study of these TF families is needed for better understanding of the signaling pathways involved in WRKY-mediated regulation of defense and developmental processes.
gives an overview of important regulatory circuits summarized in this review. The comparison of the individual panels highlights the regulatory ciruits, in which the individual WRKY proteins participate, and how they are connected to each other. The multiple appearance of an individual WRKY protein in different panels demonstrates the complexity of their involvement in the various signaling pathways. While relatively much information is available for the participation of some WRKYs in biotic stress responses, less is now about their involvement in abiotic stress. We are only at the beginning to understand their role in metabolism and their epigenetic control.
Figure 2A. The role of WRKYs and their genes in abiotic stress (A), biotic stress (B), the cross-talk between biotic and abiotic stress (C), metabolism (D), hormone signaling (E), and epigenetic control (F). The gray square boxes with the number refer to the individual WRKY proteins. WRKY genes are in green. The crosses demonstrate inhibiton of expression. A P in a yellow star refers to phosphorylation. Ub, ubiquitin.
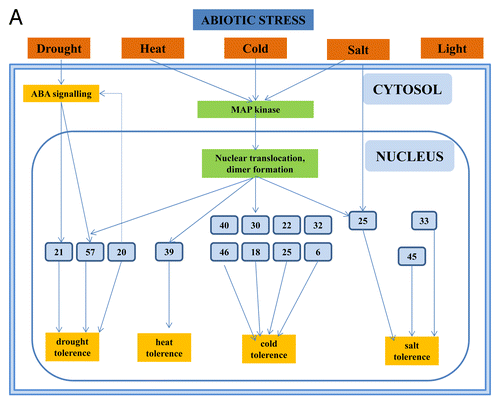
Figure 2B. The role of WRKYs and their genes in abiotic stress (A), biotic stress (B), the cross-talk between biotic and abiotic stress (C), metabolism (D), hormone signaling (E), and epigenetic control (F). The gray square boxes with the number refer to the individual WRKY proteins. WRKY genes are in green. The crosses demonstrate inhibiton of expression. A P in a yellow star refers to phosphorylation. Ub, ubiquitin.
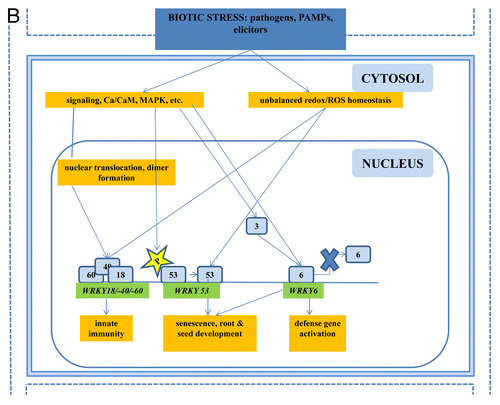
Figure 2C. The role of WRKYs and their genes in abiotic stress (A), biotic stress (B), the cross-talk between biotic and abiotic stress (C), metabolism (D), hormone signaling (E), and epigenetic control (F). The gray square boxes with the number refer to the individual WRKY proteins. WRKY genes are in green. The crosses demonstrate inhibiton of expression. A P in a yellow star refers to phosphorylation. Ub, ubiquitin.
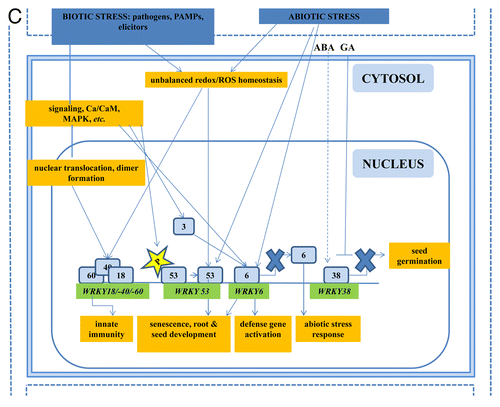
Figure 2D. The role of WRKYs and their genes in abiotic stress (A), biotic stress (B), the cross-talk between biotic and abiotic stress (C), metabolism (D), hormone signaling (E), and epigenetic control (F). The gray square boxes with the number refer to the individual WRKY proteins. WRKY genes are in green. The crosses demonstrate inhibiton of expression. A P in a yellow star refers to phosphorylation. Ub, ubiquitin.
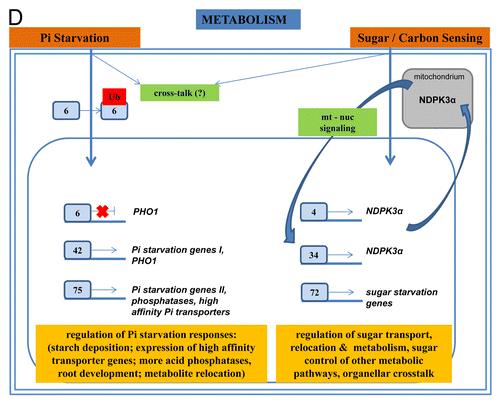
Figure 2E. The role of WRKYs and their genes in abiotic stress (A), biotic stress (B), the cross-talk between biotic and abiotic stress (C), metabolism (D), hormone signaling (E), and epigenetic control (F). The gray square boxes with the number refer to the individual WRKY proteins. WRKY genes are in green. The crosses demonstrate inhibiton of expression. A P in a yellow star refers to phosphorylation. Ub, ubiquitin.
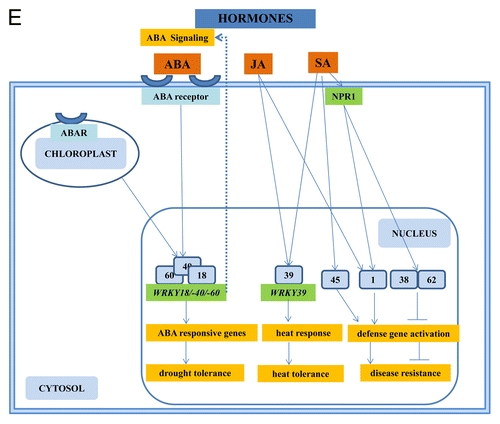
Figure 2F. The role of WRKYs and their genes in abiotic stress (A), biotic stress (B), the cross-talk between biotic and abiotic stress (C), metabolism (D), hormone signaling (E), and epigenetic control (F). The gray square boxes with the number refer to the individual WRKY proteins. WRKY genes are in green. The crosses demonstrate inhibiton of expression. A P in a yellow star refers to phosphorylation. Ub, ubiquitin.
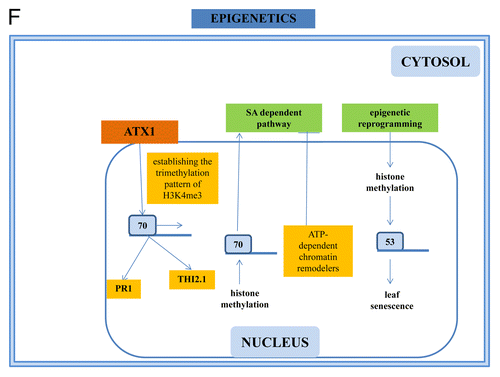
Disclosure of Potential Conflicts of Interest
No potential conflicts of interest were disclosed.
References
- Rushton PJ, Somssich IE, Ringler P, Shen QJ. WRKY transcription factors. Trends Plant Sci 2010; 15:247 - 58; http://dx.doi.org/10.1016/j.tplants.2010.02.006; PMID: 20304701
- Agarwal P, Reddy MP, Chikara J. WRKY: its structure, evolutionary relationship, DNA-binding selectivity, role in stress tolerance and development of plants. Mol Biol Rep 2011; 38:3883 - 96; http://dx.doi.org/10.1007/s11033-010-0504-5; PMID: 21107718
- Ulker B, Somssich IE. WRKY transcription factors: from DNA binding towards biological function. Curr Opin Plant Biol 2004; 7:491 - 8; http://dx.doi.org/10.1016/j.pbi.2004.07.012; PMID: 15337090
- Eulgem T, Somssich IE. Networks of WRKY transcription factors in defense signaling. Curr Opin Plant Biol 2007; 10:366 - 71; http://dx.doi.org/10.1016/j.pbi.2007.04.020; PMID: 17644023
- Zentgraf U, Laun T, Miao Y. The complex regulation of WRKY53 during leaf senescence of Arabidopsis thaliana.. Eur J Cell Biol 2010; 89:133 - 7; http://dx.doi.org/10.1016/j.ejcb.2009.10.014; PMID: 20004496
- Park CJ, Han SW, Chen X, Ronald PC. Elucidation of XA21-mediated innate immunity. Cell Microbiol 2010; 12:1017 - 25; http://dx.doi.org/10.1111/j.1462-5822.2010.01489.x; PMID: 20590657
- Ishihama N, Yoshioka H. Post-translational regulation of WRKY transcription factors in plant immunity. Curr Opin Plant Biol 2012; 15:431 - 7; http://dx.doi.org/10.1016/j.pbi.2012.02.003; PMID: 22425194
- Bhattacharjee S, Garner CM, Gassmann W. New clues in the nucleus: transcriptional reprogramming in effector-triggered immunity. Front Plant Sci 2013; 13:4 - 364; PMID: 24062762
- Chen L, Song Y, Li S, Zhang L, Zou C, Yu D. The role of WRKY transcription factors in plant abiotic stresses. Biochim Biophys Acta 2012; 1819:120 - 8; http://dx.doi.org/10.1016/j.bbagrm.2011.09.002; PMID: 21964328
- Gregersen PL, Culetic A, Boschian L, Krupinska K. Plant senescence and crop productivity. Plant Mol Biol 2013; 82:603 - 22; http://dx.doi.org/10.1007/s11103-013-0013-8; PMID: 23354836
- Song Y, Ai CR, Jing SJ, Yu DQ. Research progress on function analysis of rice WRKY gene. Rice Sci 2010; 17:60 - 72; http://dx.doi.org/10.1016/S1672-6308(08)60105-5
- Schmutz J, Cannon SB, Schlueter J, Ma J, Mitros T, Nelson W, Hyten DL, Song Q, Thelen JJ, Cheng J, et al. Genome sequence of the palaeopolyploid soybean. Nature 2010; 463:178 - 83; http://dx.doi.org/10.1038/nature08670; PMID: 20075913
- Pandey SP, Somssich IE. The role of WRKY transcription factors in plant immunity. Plant Physiol 2009; 150:1648 - 55; http://dx.doi.org/10.1104/pp.109.138990; PMID: 19420325
- Liu JJ, Ekramoddoullah AK. Identification and characterization of the WRKY transcription factor family in Pinus monticola.. Genome 2009; 52:77 - 88; http://dx.doi.org/10.1139/G08-106; PMID: 19132074
- Mangelsen E, Kilian J, Berendzen KW, Kolukisaoglu UH, Harter K, Jansson C, Wanke D. Phylogenetic and comparative gene expression analysis of barley (Hordeum vulgare) WRKY transcription factor family reveals putatively retained functions between monocots and dicots. BMC Genomics 2008; 9:194; http://dx.doi.org/10.1186/1471-2164-9-194; PMID: 18442363
- Fu ZQ, Dong X. Systemic acquired resistance: turning local infection into global defense. Annu Rev Plant Biol 2013; 64:839 - 63; http://dx.doi.org/10.1146/annurev-arplant-042811-105606; PMID: 23373699
- Grover A, Mittal D, Negi M, Lavania D. Generating high temperature tolerant transgenic plants: Achievements and challenges. Plant Sci 2013; 205-206:38 - 47; http://dx.doi.org/10.1016/j.plantsci.2013.01.005; PMID: 23498861
- Antoni R, Rodriguez L, Gonzalez-Guzman M, Pizzio GA, Rodriguez PL. News on ABA transport, protein degradation, and ABFs/WRKYs in ABA signaling. Curr Opin Plant Biol 2011; 14:547 - 53; http://dx.doi.org/10.1016/j.pbi.2011.06.004; PMID: 21742545
- Golldack D, Lüking I, Yang O. Plant tolerance to drought and salinity: stress regulating transcription factors and their functional significance in the cellular transcriptional network. Plant Cell Rep 2011; 30:1383 - 91; http://dx.doi.org/10.1007/s00299-011-1068-0; PMID: 21476089
- Rushton DL, Tripathi P, Rabara RC, Lin J, Ringler P, Boken AK, Langum TJ, Smidt L, Boomsma DD, Emme NJ, et al. WRKY transcription factors: key components in abscisic acid signalling. Plant Biotechnol J 2012; 10:2 - 11; http://dx.doi.org/10.1111/j.1467-7652.2011.00634.x; PMID: 21696534
- Nilsson L, Müller R, Nielsen TH. Dissecting the plant transcriptome and the regulatory responses to phosphate deprivation. Physiol Plant 2010; 139:129 - 43; http://dx.doi.org/10.1111/j.1399-3054.2010.01356.x; PMID: 20113436
- Chen H, Lai Z, Shi J, Xiao Y, Chen Z, Xu X. Roles of arabidopsis WRKY18, WRKY40 and WRKY60 transcription factors in plant responses to abscisic acid and abiotic stress. BMC Plant Biol 2010; 10:281; http://dx.doi.org/10.1186/1471-2229-10-281; PMID: 21167067
- Miller G, Shulaev V, Mittler R. Reactive oxygen signaling and abiotic stress. Physiol Plant 2008; 133:481 - 9; http://dx.doi.org/10.1111/j.1399-3054.2008.01090.x; PMID: 18346071
- Yamasaki K, Kigawa T, Inoue M, Watanabe S, Tateno M, Seki M, Shinozaki K, Yokoyama S. Structures and evolutionary origins of plant-specific transcription factor DNA-binding domains. Plant Physiol Biochem 2008; 46:394 - 401; http://dx.doi.org/10.1016/j.plaphy.2007.12.015; PMID: 18272381
- Guo Y, Gan S. Leaf senescence: signals, execution, and regulation. Curr Top Dev Biol 2005; 71:83 - 112; http://dx.doi.org/10.1016/S0070-2153(05)71003-6; PMID: 16344103
- Eulgem T, Rushton PJ, Robatzek S, Somssich IE. The WRKY superfamily of plant transcription factors. Trends Plant Sci 2000; 5:199 - 206; http://dx.doi.org/10.1016/S1360-1385(00)01600-9; PMID: 10785665
- Penninckx IAMA, Thomma BPHJ, Buchala A, Métraux J-P, Broekaert WF. Concomitant activation of jasmonate and ethylene response pathways is required for induction of a plant defensin gene in Arabidopsis. Plant Cell 1998; 10:2103 - 13; PMID: 9836748
- Thomma BP, Nürnberger T, Joosten MH. Of PAMPs and effectors: the blurred PTI-ETI dichotomy. Plant Cell 2011; 23:4 - 15; http://dx.doi.org/10.1105/tpc.110.082602; PMID: 21278123
- Li J, Brader G, Palva ET. The WRKY70 transcription factor: a node of convergence for jasmonate-mediated and salicylate-mediated signals in plant defense. Plant Cell 2004; 16:319 - 31; http://dx.doi.org/10.1105/tpc.016980; PMID: 14742872
- Li Z, Peng J, Wen X, Guo H. Gene network analysis and functional studies of senescence-associated genes reveal novel regulators of Arabidopsis leaf senescence. J Integr Plant Biol 2012; 54:526 - 39; http://dx.doi.org/10.1111/j.1744-7909.2012.01136.x; PMID: 22709441
- Yu S, Ligang C, Liping Z, Diqiu Y. Overexpression of OsWRKY72 gene interferes in the abscisic acid signal and auxin transport pathway of Arabidopsis. J Biosci 2010; 35:459 - 71; http://dx.doi.org/10.1007/s12038-010-0051-1; PMID: 20826955
- Murray SL, Ingle RA, Petersen LN, Denby KJ. Basal resistance against Pseudomonas syringae in Arabidopsis involves WRKY53 and a protein with homology to a nematode resistance protein. Mol Plant Microbe Interact 2007; 20:1431 - 8; http://dx.doi.org/10.1094/MPMI-20-11-1431; PMID: 17977154
- Huang D, Wu W, Abrams SR, Cutler AJ. The relationship of drought-related gene expression in Arabidopsis thaliana to hormonal and environmental factors. J Exp Bot 2008; 59:2991 - 3007; http://dx.doi.org/10.1093/jxb/ern155; PMID: 18552355
- Koyama T, Nii H, Mitsuda N, Ohta M, Kitajima S, Ohme-Takagi M, Sato F. A regulatory cascade involving class II ETHYLENE RESPONSE FACTOR transcriptional repressors operates in the progression of leaf senescence. Plant Physiol 2013; 162:991 - 1005; http://dx.doi.org/10.1104/pp.113.218115; PMID: 23629833
- Antoni R, Rodriguez L, Gonzalez-Guzman M, Pizzio GA, Rodriguez PL. News on ABA transport, protein degradation, and ABFs/WRKYs in ABA signaling. Curr Opin Plant Biol 2011; 14:547 - 53; http://dx.doi.org/10.1016/j.pbi.2011.06.004; PMID: 21742545
- Vanderauwera S, Vandenbroucke K, Inzé A, van de Cotte B, Mühlenbock P, De Rycke R, Naouar N, Van Gaever T, Van Montagu MC, Van Breusegem F. AtWRKY15 perturbation abolishes the mitochondrial stress response that steers osmotic stress tolerance in Arabidopsis. Proc Natl Acad Sci U S A 2012; 109:20113 - 8; http://dx.doi.org/10.1073/pnas.1217516109; PMID: 23169634
- Grunewald W, De Smet I, De Rybel B, Robert HS, van de Cotte B, Willemsen V, Gheysen G, Weijers D, Friml J, Beeckman T. Tightly controlled WRKY23 expression mediates Arabidopsis embryo development. EMBO Rep 2013; 14:1136 - 42; http://dx.doi.org/10.1038/embor.2013.169; PMID: 24157946
- Grunewald W, De Smet I, Lewis DR, Löfke C, Jansen L, Goeminne G, Vanden Bossche R, Karimi M, De Rybel B, Vanholme B, et al. Transcription factor WRKY23 assists auxin distribution patterns during Arabidopsis root development through local control on flavonol biosynthesis. Proc Natl Acad Sci U S A 2012; 109:1554 - 9; http://dx.doi.org/10.1073/pnas.1121134109; PMID: 22307611
- Xu L, Jin L, Long L, Liu L, He X, Gao W, Zhu L, Zhang X. Overexpression of GbWRKY1 positively regulates the Pi starvation response by alteration of auxin sensitivity in Arabidopsis. Plant Cell Rep 2012; 31:2177 - 88; http://dx.doi.org/10.1007/s00299-012-1328-7; PMID: 22890372
- Wildermuth M, Jones A. The role of salicylic acid in Arabidopsis root growth. In: 20th International Conference On Arabidopsis Research. Abstract, 2009.
- Zhang Z, Li Q, Li Z, Staswick PE, Wang M, Zhu Y, He Z. Dual regulation role of GH3.5 in salicylic acid and auxin signaling during Arabidopsis-Pseudomonas syringae interaction. Plant Physiol 2007; 145:450 - 64; http://dx.doi.org/10.1104/pp.107.106021; PMID: 17704230
- Jiang CJ, Shimono M, Sugano S, Kojima M, Liu X, Inoue H, Sakakibara H, Takatsuji H. Cytokinins act synergistically with salicylic acid to activate defense gene expression in rice. Mol Plant Microbe Interact 2013; 26:287 - 96; http://dx.doi.org/10.1094/MPMI-06-12-0152-R; PMID: 23234404
- Li J, Nie X, Tan JL, Berger F. Integration of epigenetic and genetic controls of seed size by cytokinin in Arabidopsis. Proc Natl Acad Sci U S A 2013; 110:15479 - 84; http://dx.doi.org/10.1073/pnas.1305175110; PMID: 24003120
- Huang T, Duman JG. Cloning and characterization of a thermal hysteresis (antifreeze) protein with DNA-binding activity from winter bittersweet nightshade, Solanum dulcamara.. Plant Mol Biol 2002; 48:339 - 50; http://dx.doi.org/10.1023/A:1014062714786; PMID: 11905961
- Kang X, Li W, Zhou Y, Ni M. A WRKY transcription factor recruits the SYG1-like protein SHB1 to activate gene expression and seed cavity enlargement. PLoS Genet 2013; 9:e1003347; http://dx.doi.org/10.1371/journal.pgen.1003347; PMID: 23505389
- Ngo QA, Baroux C, Guthörl D, Mozerov P, Collinge MA, Sundaresan V, Grossniklaus U. The Armadillo repeat gene ZAK IXIK promotes Arabidopsis early embryo and endosperm development through a distinctive gametophytic maternal effect. Plant Cell 2012; 24:4026 - 43; http://dx.doi.org/10.1105/tpc.112.102384; PMID: 23064319
- Gallou A, Declerck S, Cranenbrouck S. Transcriptional regulation of defence genes and involvement of the WRKY transcription factor in arbuscular mycorrhizal potato root colonization. Funct Integr Genomics 2012; 12:183 - 98; http://dx.doi.org/10.1007/s10142-011-0241-4; PMID: 21811781
- Wu KL, Guo ZJ, Wang HH, Li J. The WRKY family of transcription factors in rice and Arabidopsis and their origins. DNA Res 2005; 12:9 - 26; http://dx.doi.org/10.1093/dnares/12.1.9; PMID: 16106749
- Duan MR, Nan J, Liang YH, Mao P, Lu L, Li L, Wei C, Lai L, Li Y, Su XD. DNA binding mechanism revealed by high resolution crystal structure of Arabidopsis thaliana WRKY1 protein. Nucleic Acids Res 2007; 35:1145 - 54; http://dx.doi.org/10.1093/nar/gkm001; PMID: 17264121
- Ciolkowski I, Wanke D, Birkenbihl RP, Somssich IE. Studies on DNA-binding selectivity of WRKY transcription factors lend structural clues into WRKY-domain function. Plant Mol Biol 2008; 68:81 - 92; http://dx.doi.org/10.1007/s11103-008-9353-1; PMID: 18523729
- Wang Q, Wang M, Zhang X, Hao B, Kaushik SK, Pan Y. WRKY gene family evolution in Arabidopsis thaliana.. Genetica 2011; 139:973 - 83; http://dx.doi.org/10.1007/s10709-011-9599-4; PMID: 21805321
- Riaño-Pachón DM, Ruzicic S, Dreyer I, Mueller-Roeber B. PlnTFDB: an integrative plant transcription factor database. BMC Bioinformatics 2007; 8:42; http://dx.doi.org/10.1186/1471-2105-8-42; PMID: 17286856
- Chinnusamy V, Schumaker K, Zhu JK. Molecular genetic perspectives on cross-talk and specificity in abiotic stress signalling in plants. J Exp Bot 2004; 55:225 - 36; http://dx.doi.org/10.1093/jxb/erh005; PMID: 14673035
- Rushton PJ, Somssich IE. Transcriptional control of plant genes responsive to pathogens. Curr Opin Plant Biol 1998; 1:311 - 5; http://dx.doi.org/10.1016/1369-5266(88)80052-9; PMID: 10066598
- Logemann E, Birkenbihl RP, Rawat V, Schneeberger K, Schmelzer E, Somssich IE. Functional dissection of the PROPEP2 and PROPEP3 promoters reveals the importance of WRKY factors in mediating microbe-associated molecular pattern-induced expression. New Phytol 2013; 198:1165 - 77; http://dx.doi.org/10.1111/nph.12233; PMID: 23496690
- Zhang Y, Yang Y, Fang B, Gannon P, Ding P, Li X, Zhang Y. Arabidopsis snc2-1D activates receptor-like protein-mediated immunity transduced through WRKY70. Plant Cell 2010; 22:3153 - 63; http://dx.doi.org/10.1105/tpc.110.074120; PMID: 20841424
- Shen QH, Saijo Y, Mauch S, Biskup C, Bieri S, Keller B, Seki H, Ulker B, Somssich IE, Schulze-Lefert P. Nuclear activity of MLA immune receptors links isolate-specific and basal disease-resistance responses. Science 2007; 315:1098 - 103; http://dx.doi.org/10.1126/science.1136372; PMID: 17185563
- Shang Y, Yan L, Liu ZQ, Cao Z, Mei C, Xin Q, Wu FQ, Wang XF, Du SY, Jiang T, et al. The Mg-chelatase H subunit of Arabidopsis antagonizes a group of WRKY transcription repressors to relieve ABA-responsive genes of inhibition. Plant Cell 2010; 22:1909 - 35; http://dx.doi.org/10.1105/tpc.110.073874; PMID: 20543028
- Jiang CJ, Shimono M, Sugano S, Kojima M, Yazawa K, Yoshida R, Inoue H, Hayashi N, Sakakibara H, Takatsuji H. Abscisic acid interacts antagonistically with salicylic acid signaling pathway in rice-Magnaporthe grisea interaction. Mol Plant Microbe Interact 2010; 23:791 - 8; http://dx.doi.org/10.1094/MPMI-23-6-0791; PMID: 20459318
- Schweizer F, Bodenhausen N, Lassueur S, Masclaux FG, Reymond P. Differential contribution of transcription factors to Arabidopsis thaliana defense against Spodoptera littoralis.. Front Plant Sci 2013; 4:13; http://dx.doi.org/10.3389/fpls.2013.00013; PMID: 23382734
- Gao X, He P. Nuclear dynamics of Arabidopsis calcium-dependent protein kinases in effector-triggered immunity. Plant Signal Behav 2013; 8:e23868; http://dx.doi.org/10.4161/psb.23868; PMID: 23425856
- Park CY, Lee JH, Yoo JH, Moon BC, Choi MS, Kang YH, Lee SM, Kim HS, Kang KY, Chung WS, et al. WRKY group IId transcription factors interact with calmodulin. FEBS Lett 2005; 579:1545 - 50; http://dx.doi.org/10.1016/j.febslet.2005.01.057; PMID: 15733871
- Miao Y, Smykowski A, Zentgraf U. A novel upstream regulator of WRKY53 transcription during leaf senescence in Arabidopsis thaliana.. Plant Biol (Stuttg) 2008; 10:Suppl 1 110 - 20; http://dx.doi.org/10.1111/j.1438-8677.2008.00083.x; PMID: 18721316
- Mao G, Meng X, Liu Y, Zheng Z, Chen Z, Zhang S. Phosphorylation of a WRKY transcription factor by two pathogen-responsive MAPKs drives phytoalexin biosynthesis in Arabidopsis. Plant Cell 2011; 23:1639 - 53; http://dx.doi.org/10.1105/tpc.111.084996; PMID: 21498677
- Xie C, Zhou X, Deng X, Guo Y. PKS5, a SNF1-related kinase, interacts with and phosphorylates NPR1, and modulates expression of WRKY38 and WRKY62. J Genet Genomics 2010; 37:359 - 69; http://dx.doi.org/10.1016/S1673-8527(09)60054-0; PMID: 20621018
- Ueno Y, Yoshida R, Kishi-Kaboshi M, Matsushita A, Jiang CJ, Goto S, Takahashi A, Hirochika H, Takatsuji H. MAP kinases phosphorylate rice WRKY45. [Epub ahead of print] Plant Signal Behav 2013; 8:e24510; http://dx.doi.org/10.4161/psb.24510; PMID: 23603961
- Ay N, Irmler K, Fischer A, Uhlemann R, Reuter G, Humbeck K. Epigenetic programming via histone methylation at WRKY53 controls leaf senescence in Arabidopsis thaliana. Plant J 2009; 58:333 - 46; http://dx.doi.org/10.1111/j.0960-7412.2009.03782.x; PMID: 19143996
- Alvarez ME, Nota F, Cambiagno DA. Epigenetic control of plant immunity. Mol Plant Pathol 2010; 11:563 - 76; http://dx.doi.org/10.1111/j.1364-3703.2010.00621.x; PMID: 20618712
- Alvarez-Venegas R, Abdallat AA, Guo M, Alfano JR, Avramova Z. Epigenetic control of a transcription factor at the cross section of two antagonistic pathways. Epigenetics 2007; 2:106 - 13; http://dx.doi.org/10.4161/epi.2.2.4404; PMID: 17965588
- Zou X, Seemann JR, Neuman D, Shen QJ. A WRKY gene from creosote bush encodes an activator of the abscisic acid signaling pathway. J Biol Chem 2004; 279:55770 - 9; http://dx.doi.org/10.1074/jbc.M408536200; PMID: 15504732
- Jiang Y, Liang G, Yu D. Activated expression of WRKY57 confers drought tolerance in Arabidopsis. Mol Plant 2012; 5:1375 - 88; http://dx.doi.org/10.1093/mp/sss080; PMID: 22930734
- Liu H, Yang W, Liu D, Han Y, Zhang A, Li S. Ectopic expression of a grapevine transcription factor VvWRKY11 contributes to osmotic stress tolerance in Arabidopsis. Mol Biol Rep 2011; 38:417 - 27; http://dx.doi.org/10.1007/s11033-010-0124-0; PMID: 20354906
- Luo X, Bai X, Sun X, Zhu D, Liu B, Ji W, Cai H, Cao L, Wu J, Hu M, et al. Expression of wild soybean WRKY20 in Arabidopsis enhances drought tolerance and regulates ABA signalling. J Exp Bot 2013; 64:2155 - 69; http://dx.doi.org/10.1093/jxb/ert073; PMID: 23606412
- Babitha KC, Ramu SV, Pruthvi V, Mahesh P, Nataraja KN, Udayakumar M. Co-expression of AtbHLH17 and AtWRKY28 confers resistance to abiotic stress in Arabidopsis. Transgenic Res 2013; 22:327 - 41; http://dx.doi.org/10.1007/s11248-012-9645-8; PMID: 22948308
- Jiang Y, Deyholos MK. Functional characterization of Arabidopsis NaCl-inducible WRKY25 and WRKY33 transcription factors in abiotic stresses. Plant Mol Biol 2009; 69:91 - 105; http://dx.doi.org/10.1007/s11103-008-9408-3; PMID: 18839316
- Van Aken O, Zhang B, Law S, Narsai R, Whelan J. AtWRKY40 and AtWRKY63 modulate the expression of stress-responsive nuclear genes encoding mitochondrial and chloroplast proteins. Plant Physiol 2013; 162:254 - 71; http://dx.doi.org/10.1104/pp.113.215996; PMID: 23509177
- Lee B-H, Henderson DA, Zhu J-K. The Arabidopsis cold-responsive transcriptome and its regulation by ICE1. Plant Cell 2005; 17:3155 - 75; http://dx.doi.org/10.1105/tpc.105.035568; PMID: 16214899
- Marchive C, Léon C, Kappel C, Coutos-Thévenot P, Corio-Costet MF, Delrot S, Lauvergeat V, Wang B, Tao J, Marchive C, et al. Over-expression of VvWRKY1 in grapevines induces expression of jasmonic acid pathway-related genes and confers higher tolerance to the downy mildew. PLoS One 2013; 8:e54185; http://dx.doi.org/10.1371/journal.pone.0054185; PMID: 23342101
- Zou C, Jiang W, Yu D. Male gametophyte-specific WRKY34 transcription factor mediates cold sensitivity of mature pollen in Arabidopsis. J Exp Bot 2010; 61:3901 - 14; http://dx.doi.org/10.1093/jxb/erq204; PMID: 20643804
- Li S, Zhou X, Chen L, Huang W, Yu D. Functional characterization of Arabidopsis thaliana WRKY39 in heat stress. Mol Cells 2010; 29:475 - 83; http://dx.doi.org/10.1007/s10059-010-0059-2; PMID: 20396965
- Vance CP, Uhde-Stone C, Allan DL. Phosphorus acquisition and use: critical adaptations by plants for securing a nonrenewable resource. New Phytol 2003; 157:423 - 47; http://dx.doi.org/10.1046/j.1469-8137.2003.00695.x
- Hammond JP, Broadley MR, White PJ. Genetic responses to phosphorus deficiency. Ann Bot 2004; 94:323 - 32; http://dx.doi.org/10.1093/aob/mch156; PMID: 15292042
- Lambers H, Shane MW, Cramer MD, Pearse SJ, Veneklaas EJ. Root structure and functioning for efficient acquisition of phosphorus: Matching morphological and physiological traits. Ann Bot 2006; 98:693 - 713; http://dx.doi.org/10.1093/aob/mcl114; PMID: 16769731
- Chen YF, Li LQ, Xu Q, Kong YH, Wang H, Wu WH. The WRKY6 transcription factor modulates PHOSPHATE1 expression in response to low Pi stress in Arabidopsis. Plant Cell 2009; 21:3554 - 66; http://dx.doi.org/10.1105/tpc.108.064980; PMID: 19934380
- Devaiah BN, Karthikeyan AS, Raghothama KG. WRKY75 transcription factor is a modulator of phosphate acquisition and root development in Arabidopsis. Plant Physiol 2007; 143:1789 - 801; http://dx.doi.org/10.1104/pp.106.093971; PMID: 17322336
- Devaiah BN, Raghothama KG. Transcriptional regulation of pi starvation responses by WRKY75. Plant Signal Behav 2007; 2:424 - 5; http://dx.doi.org/10.4161/psb.2.5.4418; PMID: 19704621
- Hamburger D, Rezzonico E, MacDonald-Comber Petétot J, Somerville C, Poirier Y. Identification and characterization of the Arabidopsis PHO1 gene involved in phosphate loading to the xylem. Plant Cell 2002; 14:889 - 902; http://dx.doi.org/10.1105/tpc.000745; PMID: 11971143
- Contento AL, Kim SJ, Bassham DC. Transcriptome profiling of the response of Arabidopsis suspension culture cells to Suc starvation. Plant Physiol 2004; 135:2330 - 47; http://dx.doi.org/10.1104/pp.104.044362; PMID: 15310832
- Wang HJ, Wan AR, Hsu CM, Lee KW, Yu SM, Jauh GY. Transcriptomic adaptations in rice suspension cells under sucrose starvation. Plant Mol Biol 2007; 63:441 - 63; http://dx.doi.org/10.1007/s11103-006-9100-4; PMID: 17115300
- Hammargren J, Rosenquist S, Jansson C, Knorpp C. A novel connection between nucleotide and carbohydrate metabolism in mitochondria: sugar regulation of the Arabidopsis nucleoside diphosphate kinase 3a gene. Plant Cell Rep 2008; 27:529 - 34; http://dx.doi.org/10.1007/s00299-007-0486-5; PMID: 18057937
- Pesaresi P, Schneider A, Kleine T, Leister D. Interorganellar communication. Curr Opin Plant Biol 2007; 10:600 - 6; http://dx.doi.org/10.1016/j.pbi.2007.07.007; PMID: 17719262
- Dietz KJ, Vogel MO, Viehhauser A. AP2/EREBP transcription factors are part of gene regulatory networks and integrate metabolic, hormonal and environmental signals in stress acclimation and retrograde signalling. Protoplasma 2010; 245:3 - 14; http://dx.doi.org/10.1007/s00709-010-0142-8; PMID: 20411284
- Davletova S, Rizhsky L, Liang H, Shengqiang Z, Oliver DJ, Coutu J, Shulaev V, Schlauch K, Mittler R. Cytosolic ascorbate peroxidase 1 is a central component of the reactive oxygen gene network of Arabidopsis. Plant Cell 2005; a 17:268 - 81; http://dx.doi.org/10.1105/tpc.104.026971; PMID: 15608336
- Gadjev I, Vanderauwera S, Gechev TS, Laloi C, Minkov IN, Shulaev V, Apel K, Inzé D, Mittler R, Van Breusegem F. Transcriptomic footprints disclose specificity of reactive oxygen species signaling in Arabidopsis. Plant Physiol 2006; 141:436 - 45; http://dx.doi.org/10.1104/pp.106.078717; PMID: 16603662
- Rizhsky L, Davletova S, Liang H, Mittler R. The zinc finger protein Zat12 is required for cytosolic ascorbate peroxidase 1 expression during oxidative stress in Arabidopsis. J Biol Chem 2004; 279:11736 - 43; http://dx.doi.org/10.1074/jbc.M313350200; PMID: 14722088
- Vanderauwera S, Zimmermann P, Rombauts S, Vandenabeele S, Langebartels C, Gruissem W, Inzé D, Van Breusegem F. Genome-wide analysis of hydrogen peroxide-regulated gene expression in Arabidopsis reveals a high light-induced transcriptional cluster involved in anthocyanin biosynthesis. Plant Physiol 2005; 139:806 - 21; http://dx.doi.org/10.1104/pp.105.065896; PMID: 16183842
- Miao Y, Zentgraf U. The antagonist function of Arabidopsis WRKY53 and ESR/ESP in leaf senescence is modulated by the jasmonic and salicylic acid equilibrium. Plant Cell 2007; 19:819 - 30; http://dx.doi.org/10.1105/tpc.106.042705; PMID: 17369373
- Zhou X, Jiang Y, Yu D. WRKY22 transcription factor mediates dark-induced leaf senescence in Arabidopsis. Mol Cells 2011; 31:303 - 13; http://dx.doi.org/10.1007/s10059-011-0047-1; PMID: 21359674
- Davletova S, Schlauch K, Coutu J, Mittler R. The zinc-finger protein Zat12 plays a central role in reactive oxygen and abiotic stress signaling in Arabidopsis. Plant Physiol 2005; b 139:847 - 56; http://dx.doi.org/10.1104/pp.105.068254; PMID: 16183833
- Mittler R, Kim Y, Song L, Coutu J, Coutu A, Ciftci-Yilmaz S, Lee H, Stevenson B, Zhu JK. Gain- and loss-of-function mutations in Zat10 enhance the tolerance of plants to abiotic stress. FEBS Lett 2006; 580:6537 - 42; http://dx.doi.org/10.1016/j.febslet.2006.11.002; PMID: 17112521
- Scarpeci TE, Zanor MI, Carrillo N, Mueller-Roeber B, Valle EM. Generation of superoxide anion in chloroplasts of Arabidopsis thaliana during active photosynthesis: a focus on rapidly induced genes. Plant Mol Biol 2008; 66:361 - 78; http://dx.doi.org/10.1007/s11103-007-9274-4; PMID: 18158584
- Van Aken O, Pecenková T, van de Cotte B, De Rycke R, Eeckhout D, Fromm H, De Jaeger G, Witters E, Beemster GT, Inzé D, et al. Mitochondrial type-I prohibitins of Arabidopsis thaliana are required for supporting proficient meristem development. Plant J 2007; 52:850 - 64; http://dx.doi.org/10.1111/j.1365-313X.2007.03276.x; PMID: 17883375
- Skirycz A, De Bodt S, Obata T, De Clercq I, Claeys H, De Rycke R, Andriankaja M, Van Aken O, Van Breusegem F, Fernie AR, et al. Developmental stage specificity and the role of mitochondrial metabolism in the response of Arabidopsis leaves to prolonged mild osmotic stress. Plant Physiol 2010; 152:226 - 44; http://dx.doi.org/10.1104/pp.109.148965; PMID: 19906889
- Turck F, Zhou A, Somssich IE. Stimulus-dependent, promoter-specific binding of transcription factor WRKY1 to Its native promoter and the defense-related gene PcPR1-1 in Parsley. Plant Cell 2004; 16:2573 - 85; http://dx.doi.org/10.1105/tpc.104.024810; PMID: 15367720
- Göhre V, Jones AM, Sklenář J, Robatzek S, Weber AP. Molecular crosstalk between PAMP-triggered immunity and photosynthesis. Mol Plant Microbe Interact 2012; 25:1083 - 92; http://dx.doi.org/10.1094/MPMI-11-11-0301; PMID: 22550958
- Gao QM, Venugopal S, Navarre D, Kachroo A. Low oleic acid-derived repression of jasmonic acid-inducible defense responses requires the WRKY50 and WRKY51 proteins. Plant Physiol 2011; 155:464 - 76; http://dx.doi.org/10.1104/pp.110.166876; PMID: 21030507
- Brotman Y, Landau U, Cuadros-Inostroza Á, Tohge T, Fernie AR, Chet I, Viterbo A, Willmitzer L. Trichoderma-plant root colonization: escaping early plant defense responses and activation of the antioxidant machinery for saline stress tolerance. PLoS Pathog 2013; 9:e1003221; http://dx.doi.org/10.1371/journal.ppat.1003221; PMID: 23516362
- Besseau S, Li J, Palva ET. WRKY54 and WRKY70 co-operate as negative regulators of leaf senescence in Arabidopsis thaliana.. J Exp Bot 2012; 63:2667 - 79; http://dx.doi.org/10.1093/jxb/err450; PMID: 22268143
- Rosenvasser S, Mayak S, Friedman H. Increase in reactive oxygen species (ROS) and in senescence-associated gene transcript (SAG) levels during dark-induced senescence of Pelargonium cuttings, and the effect of gibberellic acid. Plant Sci 2006; 170:873 - 9; http://dx.doi.org/10.1016/j.plantsci.2005.12.010
- Wagner D, Przybyla D, Op den Camp R, Kim C, Landgraf F, Lee KP, Würsch M, Laloi C, Nater M, Hideg E, et al. The genetic basis of singlet oxygen-induced stress responses of Arabidopsis thaliana. Science 2004; 306:1183 - 5; http://dx.doi.org/10.1126/science.1103178; PMID: 15539603
- Laloi C, Przybyla D, Apel K. A genetic approach towards elucidating the biological activity of different reactive oxygen species in Arabidopsis thaliana. J Exp Bot 2006; 57:1719 - 24; http://dx.doi.org/10.1093/jxb/erj183; PMID: 16720605
- Mehterov N, Balazadeh S, Hille J, Toneva V, Mueller-Roeber B, Gechev T. Oxidative stress provokes distinct transcriptional responses in the stress-tolerant atr7 and stress-sensitive loh2 Arabidopsis thaliana mutants as revealed by multi-parallel quantitative real-time PCR analysis of ROS marker and antioxidant genes. Plant Physiol Biochem 2012; 59:20 - 9; http://dx.doi.org/10.1016/j.plaphy.2012.05.024; PMID: 22710144
- Rushton PJ, Torres JT, Parniske M, Wernert P, Hahlbrock K, Somssich IE. Interaction of elicitor-induced DNA-binding proteins with elicitor response elements in the promoters of parsley PR1 genes. EMBO J 1996; 15:5690 - 700; PMID: 8896462
- Chen C, Chen Z. Isolation and characterization of two pathogen- and salicylic acid-induced genes encoding WRKY DNA-binding proteins from tobacco. Plant Mol Biol 2000; 42:387 - 96; http://dx.doi.org/10.1023/A:1006399311615; PMID: 10794538
- Hara K, Yagi M, Kusano T, Sano H. Rapid systemic accumulation of transcripts encoding a tobacco WRKY transcription factor upon wounding. Mol Gen Genet 2000; 263:30 - 7; http://dx.doi.org/10.1007/PL00008673; PMID: 10732671
- Dong J, Chen C, Chen Z. Expression profiles of the Arabidopsis WRKY gene superfamily during plant defense response. Plant Mol Biol 2003; 51:21 - 37; http://dx.doi.org/10.1023/A:1020780022549; PMID: 12602888
- Kalde M, Barth M, Somssich IE, Lippok B. Members of the Arabidopsis WRKY group III transcription factors are part of different plant defense signaling pathways. Mol Plant Microbe Interact 2003; 16:295 - 305; http://dx.doi.org/10.1094/MPMI.2003.16.4.295; PMID: 12744458
- Thomas H, Morgan WG, Thomas AM, Ougham HJ. Expression of the stay-green character introgressed into Lolium temulentum Ceres from a senescence mutant of Festuca pratensis. Theor Appl Genet 1999; 99:92 - 9; http://dx.doi.org/10.1007/s001220051212
- Kilian J, Whitehead D, Horak J, Wanke D, Weinl S, Batistic O, D’Angelo C, Bornberg-Bauer E, Kudla J, Harter K. The AtGenExpress global stress expression data set: protocols, evaluation and model data analysis of UV-B light, drought and cold stress responses. Plant J 2007; 50:347 - 63; http://dx.doi.org/10.1111/j.1365-313X.2007.03052.x; PMID: 17376166
- Skibbe M, Qu N, Galis I, Baldwin IT. Induced plant defenses in the natural environment: Nicotiana attenuata WRKY3 and WRKY6 coordinate responses to herbivory. Plant Cell 2008; 20:1984 - 2000; http://dx.doi.org/10.1105/tpc.108.058594; PMID: 18641266
- van Verk MC, Bol JF, Linthorst HJ. WRKY transcription factors involved in activation of SA biosynthesis genes. BMC Plant Biol 2011; 11:89; http://dx.doi.org/10.1186/1471-2229-11-89; PMID: 21595875
- Encinas-Villarejo S, Maldonado AM, Amil-Ruiz F, de los Santos B, Romero F, Pliego-Alfaro F, Muñoz-Blanco J, Caballero JL. Evidence for a positive regulatory role of strawberry (Fragaria x ananassa) Fa WRKY1 and Arabidopsis At WRKY75 proteins in resistance. J Exp Bot 2009; 60:3043 - 65; http://dx.doi.org/10.1093/jxb/erp152; PMID: 19470657
- Rushton DL, Tripathi P, Rabara RC, Lin J, Ringler P, Boken AK, Langum TJ, Smidt L, Boomsma DD, Emme NJ, et al. WRKY transcription factors: key components in abscisic acid signalling. Plant Biotechnol J 2012; 10:2 - 11; http://dx.doi.org/10.1111/j.1467-7652.2011.00634.x; PMID: 21696534
- Kim KC, Lai Z, Fan B, Chen Z. Arabidopsis WRKY38 and WRKY62 transcription factors interact with histone deacetylase 19 in basal defense. Plant Cell 2008; 20:2357 - 71; http://dx.doi.org/10.1105/tpc.107.055566; PMID: 18776063
- Lippok B, Birkenbihl RP, Rivory G, Brümmer J, Schmelzer E, Logemann E, Somssich IE. Expression of AtWRKY33 encoding a pathogen- or PAMP-responsive WRKY transcription factor is regulated by a composite DNA motif containing W box elements. Mol Plant Microbe Interact 2007; 20:420 - 9; http://dx.doi.org/10.1094/MPMI-20-4-0420; PMID: 17427812
- Birkenbihl RP, Diezel C, Somssich IE. Arabidopsis WRKY33 is a key transcriptional regulator of hormonal and metabolic responses toward Botrytis cinerea infection. Plant Physiol 2012; 159:266 - 85; http://dx.doi.org/10.1104/pp.111.192641; PMID: 22392279
- Birkenbihl R, Somssich I. The WRKY33 transcription factor of Arabidopsis is involved in balancing the hormone signaling pathways and adjusting the metabolic response to Botrytis infection. In: 20th International Conference on Arabidopsis Research. Abstract, 2009.
- Zheng Z, Mosher SL, Fan B, Klessig DF, Chen Z. Functional analysis of Arabidopsis WRKY25 transcription factor in plant defense against Pseudomonas syringae. BMC Plant Biol 2007; 7:2; http://dx.doi.org/10.1186/1471-2229-7-2; PMID: 17214894
- Andreasson E, Jenkins T, Brodersen P, Thorgrimsen S, Petersen NH, Zhu S, Qiu JL, Micheelsen P, Rocher A, Petersen M, et al. The MAP kinase substrate MKS1 is a regulator of plant defense responses. EMBO J 2005; 24:2579 - 89; http://dx.doi.org/10.1038/sj.emboj.7600737; PMID: 15990873
- Schön M, Töller A, Diezel C, Roth C, Westphal L, Wiermer M, Somssich IE. Analyses of wrky18 wrky40 plants reveal critical roles of SA/EDS1 signaling and indole-glucosinolate biosynthesis for Golovinomyces orontii resistance and a loss-of resistance towards Pseudomonas syringae pv. tomato AvrRPS4. Mol Plant Microbe Interact 2013; 26:758 - 67; http://dx.doi.org/10.1094/MPMI-11-12-0265-R; PMID: 23617415
- Matsushita A, Inoue H, Goto S, Nakayama A, Sugano S, Hayashi N, Takatsuji H. The nuclear ubiquitin proteasome degradation affects WRKY45 function in the rice defense program. Plant J 2012; 73:302 - 13; http://dx.doi.org/10.1111/tpj.12035; PMID: 23013464
- Nakayama A, Fukushima S, Goto S, Matsushita A, Shimono M, Sugano S, Jiang CJ, Akagi A, Yamazaki M, Inoue H, et al. Genome-wide identification of WRKY45-regulated genes that mediate benzothiadiazole-induced defense responses in rice. BMC Plant Biol 2013; 13:150; http://dx.doi.org/10.1186/1471-2229-13-150; PMID: 24093634
- Inoue H, Hayashi N, Matsushita A, Xinqiong L, Nakayama A, Sugano S, Jiang CJ, Takatsuji H. Blast resistance of CC-NB-LRR protein Pb1 is mediated by WRKY45 through protein-protein interaction. Proc Natl Acad Sci U S A 2013; 110:9577 - 82; http://dx.doi.org/10.1073/pnas.1222155110; PMID: 23696671
- Marchive C, Léon C, Kappel C, Coutos-Thévenot P, Corio-Costet MF, Delrot S, Lauvergeat V. Over-expression of VvWRKY1 in grapevines induces expression of jasmonic acid pathway-related genes and confers higher tolerance to the downy mildew. PLoS One 2013; 8:e54185; http://dx.doi.org/10.1371/journal.pone.0054185; PMID: 23342101
- Rabara RC, Tripathi P, Lin J, Rushton PJ. Dehydration-induced WRKY genes from tobacco and soybean respond to jasmonic acid treatments in BY-2 cell culture. Biochem Biophys Res Commun 2013; 431:409 - 14; http://dx.doi.org/10.1016/j.bbrc.2012.12.156; PMID: 23333328
- Alexandrova KS, Conger BV. Isolation of two somatic embryogenesis-related genes from orchardgrass (Dactylis glomerata). Plant Sci 2002; 162:301 - 7; http://dx.doi.org/10.1016/S0168-9452(01)00571-4
- Lagacé M, Matton DP. Characterization of a WRKY transcription factor expressed in late torpedo-stage embryos of Solanum chacoense.. Planta 2004; 219:185 - 9; http://dx.doi.org/10.1007/s00425-004-1253-2; PMID: 15045588
- Ishida T, Hattori S, Sano R, Inoue K, Shirano Y, Hayashi H, Shibata D, Sato S, Kato T, Tabata S, et al. Arabidopsis TRANSPARENT TESTA GLABRA2 is directly regulated by R2R3 MYB transcription factors and is involved in regulation of GLABRA2 transcription in epidermal differentiation. Plant Cell 2007; 19:2531 - 43; http://dx.doi.org/10.1105/tpc.107.052274; PMID: 17766401
- Guo Y, Cai Z, Gan S. Transcriptome of Arabidopsis leaf senescence. Plant Cell Environ 2004; 27:521 - 49; http://dx.doi.org/10.1111/j.1365-3040.2003.01158.x
- Miao Y, Jiang J, Ren Y, Zhao Z. The single-stranded DNA-binding protein WHIRLY1 represses WRKY53 expression and delays leaf senescence in a developmental stage-dependent manner in Arabidopsis. Plant Physiol 2013; 163:746 - 56; http://dx.doi.org/10.1104/pp.113.223412; PMID: 23922267
- Hu Y, Dong Q, Yu D. Arabidopsis WRKY46 coordinates with WRKY70 and WRKY53 in basal resistance against pathogen Pseudomonas syringae. Plant Sci 2012; 185-186:288 - 97; http://dx.doi.org/10.1016/j.plantsci.2011.12.003; PMID: 22325892
- Miao Y, Zentgraf U. A HECT E3 ubiquitin ligase negatively regulates Arabidopsis leaf senescence through degradation of the transcription factor WRKY53. Plant J 2010; 63:179 - 88; http://dx.doi.org/10.1111/j.1365-313X.2010.04233.x; PMID: 20409006
- Miao Y, Laun TM, Smykowski A, Zentgraf U. Arabidopsis MEKK1 can take a short cut: it can directly interact with senescence-related WRKY53 transcription factor on the protein level and can bind to its promoter. Plant Mol Biol 2007; 65:63 - 76; http://dx.doi.org/10.1007/s11103-007-9198-z; PMID: 17587183
- Krause K, Kilbienski I, Mulisch M, Rödiger A, Schäfer A, Krupinska K. DNA-binding proteins of the Whirly family in Arabidopsis thaliana are targeted to the organelles. FEBS Lett 2005; 579:3707 - 12; http://dx.doi.org/10.1016/j.febslet.2005.05.059; PMID: 15967440
- Grabowski E, Miao Y, Mulisch M, Krupin-ska K. Whirly1 in barley leaves is located in chloroplasts and nuclei of the same cell. Plant Physiol 2008; 147:1 - 5; http://dx.doi.org/10.1104/pp.108.122796
- Miao Y, Isemer R, Krupinska. Two dual-targeted proteins antagonistically regulate WRKY53 dependent leaf senescence in Arabidopsis. 21ST INTERNATIONAL CONFERENCE ON ARABIDOPSIS RESEARCH, 2010. 501736920
- Reusche M, Klásková J, Thole K, Truskina J, Novák O, Janz D, Strnad M, Spíchal L, Lipka V, Teichmann T. Stabilization of cytokinin levels enhances Arabidopsis resistance against Verticillium longisporum. Mol Plant Microbe Interact 2013; 26:850 - 60; http://dx.doi.org/10.1094/MPMI-12-12-0287-R; PMID: 23594348
- Pandey SP, Roccaro M, Schön M, Logemann E, Somssich IE. Transcriptional reprogramming regulated by WRKY18 and WRKY40 facilitates powdery mildew infection of Arabidopsis. Plant J 2010; 64:912 - 23; http://dx.doi.org/10.1111/j.1365-313X.2010.04387.x; PMID: 21143673
- Li S, Fu Q, Chen L, Huang W, Yu D. Arabidopsis thaliana WRKY25, WRKY26, and WRKY33 coordinate induction of plant thermotolerance. Planta 2011; 233:1237 - 52; http://dx.doi.org/10.1007/s00425-011-1375-2; PMID: 21336597
- Zheng Z, Qamar SA, Chen Z, Mengiste T. Arabidopsis WRKY33 transcription factor is required for resistance to necrotrophic fungal pathogens. Plant J 2006; 48:592 - 605; http://dx.doi.org/10.1111/j.1365-313X.2006.02901.x; PMID: 17059405