Abstract
Nicotiana benthamiana is a potential host to several plant pathogens, and immature leaves of N. benthamiana are susceptible to Phytophthora infestans. In contrast, mature leaves of N. benthamiana are weakly susceptible and show basal resistance to P. infestans. We screened a gene-silenced mature plant showing high resistance to P. infestans, designated as DS2 (Disease suppression 2). The deduced amino acid sequence of cDNA responsible for DS2 encoded a putative aminoacylase. Growth of P. infestans decreased in DS2 plants. Trypan blue staining revealed inhibited hyphae growth of P. infestans with an increased number of dead cells under the penetration site in DS2 plants. Consistent with growth inhibition of P. infestans, defense responses such as reactive oxygen generation and expression of a salicylic acid-dependent PR-1a increased markedly in DS2 plants compared with that of control plants. DS2 phenotype was compromised in NahG plants, suggesting DS2 phenotype depends on the salicylic acid signaling pathway. Accelerated defense response was observed in DS2 plants elicited by INF1 elicitin as well as by NbMEK2DD, which is the constitutive active form of NbMEK2, and act as a downstream regulator of INF1 perception. On the other hand, INF1- and NbMEK2DD-induced defense responses were prevented by DS2-overexpressing transgenic tobacco. These results suggest that DS2 negatively regulates plant defense responses against P. infestans via NbMEK2 and SA-dependent signaling pathway in N. benthamiana.
Introduction
Plants have evolved innate immune responses to detect and respond quickly to pathogen infections.Citation1 They use transmembrane pattern recognition receptors that recognize pathogen-associated molecular patterns (PAMPs) at the cell surface. Plants perceive bacterial flagellin, EF-Tu, and fungal chitin oligomers through their cognate receptors FLS2, EFR, and CERK1, respectively.Citation2,Citation3 Plants also recognize avirulent gene products as pathogen effectors by polymorphic receptor resistance proteins, typically containing nucleotide-binding leucine-rich repeat.Citation1 After recognition events, plants activate immune responses including generation of reactive oxygen species (ROS),Citation4 the hypersensitive response (HR),Citation5 production of phytoalexins,Citation6 and induction of pathogenesis-related proteins.Citation7 A common feature of plant active defense responses is the transcriptional activation of a large number of genes upon pathogen infection or treatment with pathogen elicitors.Citation8 Some of the pathogen-induced genes encode proteins with direct antimicrobial activities or enzymes involved in biosynthesis of antimicrobial compounds. Other pathogen-induced genes encode proteins with regulatory functions in signal transduction pathways of plant defense responses.Citation9 Knowledge of molecular mechanisms of disease resistance has increased tremendously in recent years. However, little is known about the molecular basis of disease susceptibility.
Our strategy to isolate candidates for genes required for susceptibility (plant disease susceptibility factors) was to screen knock-down plants showing a disease-resistant phenotype. Virus-induced gene silencing (VIGS) is a powerful tool for analyzing gene function. Based on this principle, we performed VIGS screening of genes related to disease susceptibility using Nicotiana benthamiana and the potato virus X vector system. We previously isolated a plant that showed high resistance to Ralstonia solanacearum, designated as DS1 (Disease suppression 1).Citation10 In this study, we screened a VIGS plant that showed barely noticeable late blight symptoms after inoculation with Phytophthora infestans, and designated it as DS2 (Disease suppression 2). We analyzed and discussed the molecular mechanisms of the DS2 phenotype.
Results
DS2 plants showed enhanced resistance against P. infestans
We previously reported that DS1 (Disease suppression 1) plants showed high resistance to Ralstonia solanacearum, the causal agent of bacterial wilt, which is a serious disease in Solanaceae plants.Citation10 Potato late blight is also an important disease like bacterial wilt disease. Phytophthora infestans is the causal agent of late blight in potato, and a potential pathogen to Nicotiana benthamiana.Citation11 In this study, we focused on resistance against P. infestans. Therefore, we screened a gene-silenced plant showing high resistance to P. infestans using high throughput VIGS, and designated the plant as DS2 (Disease suppression 2) (). Expression of DS2 decreased markedly in the silenced plants compared with that in control plants (). There was no noticeable morphological difference between control and DS2-silenced plants (). All control leaves were infected and sporulating 6 d after inoculation with P. infestans. In contrast, sporulation was barely observed in the DS2 plant leaves (). At 4 d after inoculation with P. infestans, an approximately 15-fold reduction in growth of P. infestans was observed in DS2 plants compared with control plants ().
Figure 1. DS2 plants show enhanced disease resistance to P. infestans. (A) Characteristic symptoms in control and DS2-silenced plant leaves inoculated with P. infestans. Photograph was taken 4 d after inoculation. Similar results were obtained from 3 independent experiments. (B) Total RNA was isolated from control and DS2 plant leaves. DS2 expression levels were analyzed using qRT-PCR as described in Materials and Methods. Values are means of 3 replicate experiments ± SD. Asterisks denote values significantly different from those of control plants (*, P < 0.05, t test). (C) Morphological observation of DS2 plants. Photograph was taken 3 wk after inoculation with Agrobacterium. (D) Plant growth was determined by measuring stem length. Values are means of 10 replicate experiments ± SD (E) Disease development of late blight was evaluated as described in Materials and Methods. (F) Biomass of P. infestans in control and DS2 plant leaves 4 d after inoculation. Total DNA was isolated from each plant after inoculation. The biomass of P. infestans was determined using qRT-PCR with P. infestans-specific primers. Data are means ± SD from 3 independent experiments. Asterisks denote values significantly different from those of control plants (*, P < 0.05, t test). (G) Microscopic observation of N. benthamiana leaves 36 h after inoculation with P. infestans. Inoculated leaves were stained with trypan blue to show dead plant cells and fungal hyphal structures. Red arrows and black arrows indicate the hyphae and zoospore of P. infestans, respectively. Yellow arrows represent dead plant cells. Scale bars = 50 µm. (H) Reactive oxygen species was detected by chemiluminescence intensities after inoculation with P. infestans or water (Mock). Asterisks denote values significantly different from those of control plants (*, P < 0.05, t test). (I) Total RNA was isolated from control and DS2 plant leaves 24 h after inoculation with P. infestans. NbPR-1a expression levels were analyzed using qRT-PCR as described in Materials and Methods. Data are means of 3 replicate experiments ± SD. Asterisks denote values significantly different from those of control plants (*, P < 0.05, t test).
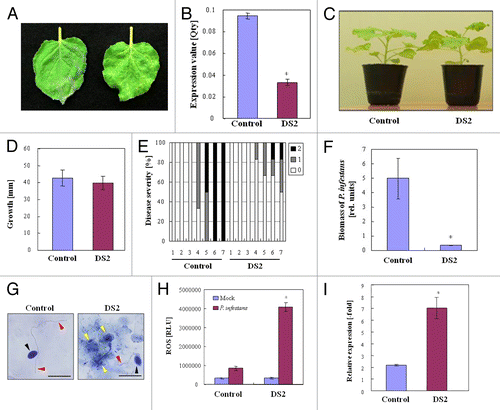
DS2 plants showed accelerated defense responses against P. infestans
In general, disease resistance to pathogens correlates to enhanced defense responses. Therefore, we first examined cell death, the most characterized defense response, in control and DS2 plants inoculated with P. infestans. Trypan blue staining showed that there were no detectable dead plant cells in control plants 36 h after inoculation, and P. infestans hyphae developed extensively in the intercellular spaces of mesophyll cells. In DS2 plants, blue-stained epidermal cells were clearly observed under and around the penetration site of P. infestans, whereas hyphal growth was barely detected (). These data suggest that hyphal growth of P. infestans was inhibited in DS2 plants with an increased number of dead cells. Because cell death responses are usually accompanied by ROS production and defense-related gene expression, we evaluated ROS accumulation and PR-gene level in control and DS2 plants in response to P. infestans infection. At 24 h after inoculation with P. infestans, a high level of ROS production was observed in DS2 plants compared with control plants (). Moreover, expression of salicylic acid (SA)-responsive PR-1a increased significantly in DS2 plant leaves 24 h after inoculation ().
DS2 encodes aminoacylase-like gene
The deduced amino acid sequence of full-length DS2 shows 97% and 86% identity with its ortholog in N. tabacum (NtACY1) and Solanum tuberosum (StACY), and 85%, 72%, 70%, and 67% identity with uncharacterized protein from Solanum lycopersicum (SlUP), putative aminoacylase 1 from Ricinus communis (RcACY1P), peptidase M20 family protein from Arabidopsis thaliana (AtM20FP), and aminoacylase 1 from Medicago truncatula (MtACY1) (), respectively. The residues required for ACY activity, His80, Asp113, Glu148, Glu175, and His373 were highly conserved in DS2 ().Citation12 These results suggest that DS2 might act as an ACY1 in N. benthamiana.
Figure 2. Sequence alignment of DS2 and aminoacylase from various plant species. Alignment of deduced amino acid sequence of DS2 (AB894320) and its ortholog in N. tabacum (NtACY:AB894399) and Solanum tuberosum (StACY1: Sotub01 g049740.1.1), uncharacterized protein from Solanum lycopersicum (SlUP:K4B489), putative aminoacylase 1 from Ricinus communis (RcACY1P:B9RKY8), peptidase M20 family protein from Arabidopsis thaliana (AtM20FP:Q8S9L3), and aminoacylase 1 from Medicago truncatula (MtACY1:G71BI0). Asterisks (*) denote amino acid residues that are identical. Bars represent gaps. Black boxes and gray boxes indicate ArgE/DapE/ACY1/CPG2/YscS conserved sites and peptidase M20 domains, respectively. Amino acid residues that are related to proton acceptor and zinc binding are shown with underlines and dashed underlines, respectively. The multiple-protein alignment was constructed using Clustal Omega. Functional sites and domains were predicted with InterPro.
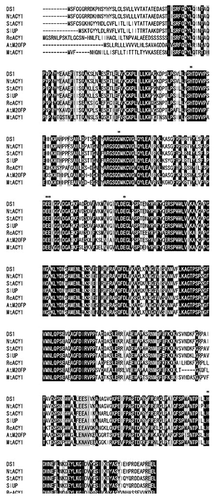
SA signaling plays crucial role in DS2 phenotype
DS2 phenotype was accompanied by enhanced expression of PR-1a during P. infestans infection. To determine whether the enhanced resistance in DS2 plants is caused by SA signaling or not, we used the N. benthamiana-expressing SA-degrading enzyme NahG that suppresses SA signaling. We created a VIGS plant based on NahG (NahG:DS2) plants without noticeable growth phenotypes (). Both NahG control and NahG:DS2 plants were infected with P. infestans (). At 4 d after inoculation with P. infestans, an increase in the biomass of P. infestans was observed in NahG:DS2 plants similar to NahG control (). Furthermore, no significant differences were observed in defense responses to P. infestans, such as cell death, ROS production, and PR-1a expression, between NahG control and NahG:DS2 plants (). These results suggest that SA signaling pathways are required for DS2 phenotype.
Figure 3. Role of SA in DS2 phenotype against P. infestans. (A) Total RNA was isolated from leaves of NahG control and NahG:DS2 plants. DS2 expression levels were analyzed using qRT-PCR as described in Materials and Methods. (B) Morphological observation of NahG and NahG:DS2 plants. Photograph was taken 3 wk after inoculation. (C) Plant growth was determined by measuring stem length as described in . (D) Characteristic symptoms in NahG control and NahG:DS2 plant leaves inoculated with P. infestans. Photograph was taken 4 d after inoculation. (E) Evaluation of disease symptom in NahG control and NahG DS2 plant leaves after inoculation as described Materials and Methods. (F) Biomass of P. infestans in NahG control and NahG:DS2 plant leaves 4 d after inoculation as described in . (G) Microscopic observation of N. benthamiana leaves 36 h after inoculation as described in . (H) Reactive oxygen species was detected using L-012 after inoculation with P. infestans or water (Mock) as described in . (I) Total RNA was isolated from NahG control and NahG:DS2 plant leaves 24 h after inoculation with P. infestans. NbPR-1a expression levels were analyzed using qRT-PCR as described in Materials and Methods.
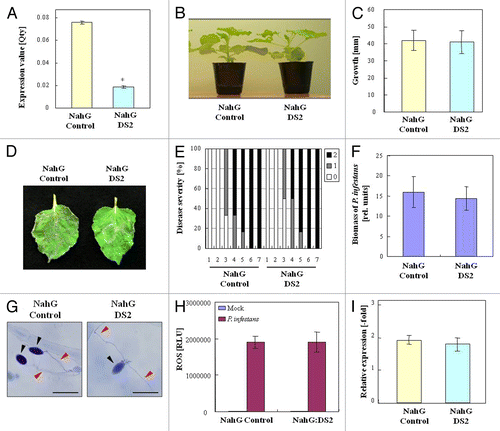
DS2 regulates PTI signaling
It has been shown that INF1 is a major secretory protein of P. infestans, and acts as a PAMP, inducing PAMP-triggered immunity (PTI) in Nicotiana spp.Citation13,Citation14 To determine whether DS2 phenotype was related to PTI, control, and DS2 plant leaves were inoculated with Agrobacterium carrying the INF1. We observed necrotic lesions 2 d after inoculation with Agrobacterium carrying INF1 in DS2-silenced plants, whereas no lesions were visible in control plants (). At 3 d after inoculation, INF1-triggered necrotic lesions also appeared in control plants (data not shown). To investigate a possible role of DS2 in PTI, we further analyzed INF1-triggered responses in control and DS2-silenced plants. Compared with control plants, DS2 plants showed accelerated cell death in response to INF1 (). Furthermore, INF1-elicited ROS production also increased in DS2 plants compared with that in control plants ().
Figure 4. Acceleration of defense-related responses in DS2 plants elicited by INF1 and NbMEK2DD in N. benthamiana. Control and DS2 plant leaves were inoculated with A. tumefaciens carrying INF1, NbMEK2DD or GUS construct. (A, E) Appearance of necrotic lesions in control and DS2 plant leaves. Photograph was taken 2 d after inoculation. (B, F) Cell death induction in control and DS2 plant leaves 2 d after inoculation. Inoculated leaves were stained with trypan blue to show dead plant cells. Scale bars = 200 µm. (C, G) Cell death was quantified by ion leakage from leaves 2 d after inoculation. Data are means ± SD from 3 independent experiments. Asterisks denote values significantly different from those of control plants (*, P < 0.05, t test). (D, H) Reactive oxygen species was detected using L-012 after inoculation with A. tumefaciens carrying the indicated gene constructs. Chemiluminescence intensities were quantified as described in .
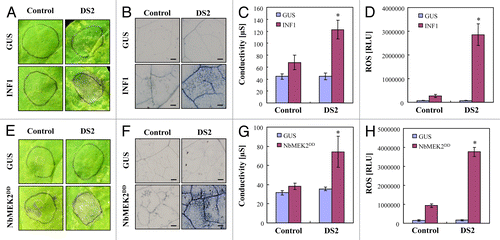
Relationship of DS2 to MAP kinase signaling
Recent studies have revealed that mitogen-activated protein kinase (MAPK) cascades have a crucial role in PTI. In N. benthamiana, NbMEK2 positively regulates production of ROS and cell death elicited by INF1 protein.Citation15 To further elucidate the role of DS2 in PAMP signaling after INF1 perception, NbMEK2DD, a constitutive active form of NbMEK2, was transiently expressed in control and DS2 plant leaves. Consistent with the enhanced responses to INF1, DS2 plants showed acceleration of NbMEK2DD-induced necrotic lesions, cell death, and ROS production (), compared with control plants.
Overexpression of DS2 exhibited INF1- or NbMEK2DD-elicited defense responses
INF1- or NbMEK2DD-elicited responses were accelerated in DS2 plants, suggesting that DS2 might act as a negative regulator of these responses. To test this hypothesis, we created DS2-overexpressing transgenic tobaccos (DS2-OX#2, OX#9) (). We observed necrotic lesions caused by INF1 or NbMEK2DD in GFP-OX plants, whereas only weak lesions were visible in both DS2-OX#2 and DS2-OX#9 plants at least 2 d after inoculation (). Both INF1 and NbMEK2DD expression induced cell death in GFP-OX leaves, whereas DS2-overexpressing plants showed markedly suppressed cell death ().
Figure 5. Reduction in defense responses in DS2-overexpressing plants elicited by INF1 or NbMEK2DD. GFP- or DS2-overexpressing tobacco leaves were inoculated with A. tumefaciens carrying INF1, NbMEK2DD or GUS construct. (A) Morphological observation of DS2-overexpressing plants. Photograph was taken 2 mo after germination. (B) Genomic DNA was prepared from GFP-transformed (GFP-OX) or DS2-transformed plants (DS2-OX#2 and DS2-OX#9). Genomic PCR was applied to detect the transformed vector using specific primers based on 35S promoter and NOS terminator. (C) Expression of DS2 in transgenic tobacco plants. Total RNA was isolated from fully expanded leaves of GFP-transformed (GFP-OX) or DS2-transformed plants (DS2-OX#2 and DS2-OX#9). DS2 expression levels were analyzed using qRT-PCR as described in . (D) Appearance of INF1- or NbMEK2DD-induced necrotic lesions in GFP-transformed (GFP-OX) and DS2-overexpressing plants (DS2-OX#2, DS2-OX#9). Photograph was taken 3 d after inoculation with A. tumefaciens carrying the indicated gene construct. (E) Cell death induction in tobacco leaves 3 d after inoculation with A. tumefaciens carrying the indicated gene construct. Inoculated leaves were stained with trypan blue. Scale bars = 400 µm. (F, G) Cell death was quantified by ion leakage from the inoculated leaves as described in .
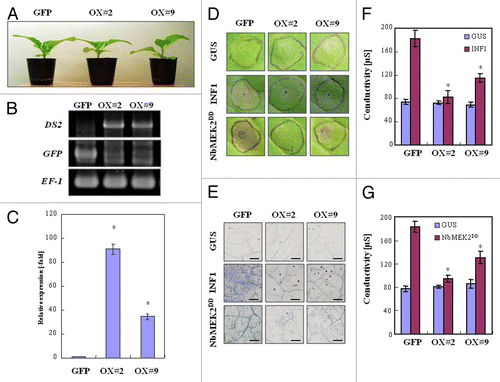
Discussion
Potato late blight’s impact on humankind is rivaled by few other plant diseases. Phytophthora infestans is the causal agent of late blight in potato and has continued to wreak havoc on potato fields throughout the world. Late blight remains the most destructive disease, resulting in annual losses of potatoes. Therefore, development of an effective method of controlling the disease is important for plant pathologists.Citation16 P. infestans is a potent pathogen of N. benthamiana.Citation11 Therefore, we searched for a P. infestans-resistant plant by high-throughput screening with VIGS, and isolated a DS2 plant. Growth of P. infestans was dramatically suppressed in DS plants. Microscopic analysis showed inhibited hyphae growth of P. infestans. We also observed cell death induction around P. infestans-penetrating areas (). These observations suggested that induction of HR-like defense in the DS2 plant against P. infestans.
Previous reports showed the relationship between SA and defense responses against P. infestans. Hyphal wall elicitors of P. infestans activate a 51-kD SA-inducible protein kinase-like (SIPK-like) MAPK in potato tubers.Citation17 In addition, resistance against P. infestans required NbrbohB, which was induced by SA.Citation18 SYNTAXIN-RELATED 1 (StSYR1) silencing confirmed resistance against P. infestans with correlation with the constitutive accumulation of salicylic acid and PR-1 transcripts.Citation19 Transgenic NahG plants showed a drastic enhancement of pathogen growth in potato plants by depletion of SA, indicating SA is an important compound required for basal defense of potato against P. infestans.Citation20 In addition, virus-induced gene silencing of NbICS1, the genes for SA biosynthesis, reduced resistance of mature N. benthamiana against P. infestans indicating the requirement of SA for the resistance of mature N. benthamiana.Citation21 Present data showed that the appearance of the DS2 phenotype might be SA-dependent signaling, since DS2 plants showed overexpression of SA-dependent PR-1a against P. infestans infection, and DS2 phenotype was compromised in NahG plants (, ). Plants activate distinct defense responses depending on the lifestyle of the attacking pathogens.Citation22 In these responses, SA plays an important signaling role, and induces defense against biotrophic pathogens that feed and reproduce on live host cells. The oomycete P. infestans is a near-obligate (a potential biotroph) pathogen.Citation23 Therefore, dependency of DS2 phenotype on SA is in good agreement with the lifestyle of P. infestans.
Plants commonly use two distinct classes of immune responses, including PTI and ETI. DS2 plants showed accelerated defense response, HR and ROS, induced by INF1. Additionally, the defense responses induced by NbMEK2DD, which act as a signaling component downstream regulator of INF1 perception,Citation18 were also enhanced in DS2 plants. In addition, INF1- and NbMEK2DD-induced defense responses were prevented by overexpression of DS2. Therefore, DS2 may act in INF1 signaling downstream of NbMEK2. In contrast, no differences between control and DS2 plants were observed in the cell death caused by the interaction between Avr3a and its cognate R3a (data not shown). These results suggest that DS2 negatively regulates plant defense responses triggered by INF1, but is not related to defense triggered by Avr3a-R3a interaction. Further analysis is needed to not only clarify the role of DS2 in signaling other different PAMPs and effectors, but also different signaling mechanisms between INF1 (PAMP) and Avr3a-R3a (effector). DS2 might be a useful tool for the analysis.
We found that DS2 encoded a gene containing conserved amino acid residues responsible for ACY activity (). A homology search of DS2 indicated ACY-like genes are conserved in several dicot plant species, including potato and tomato. Therefore, DS2-like ACY might function in potato plants during natural interaction between potato and P. infestans. Aminoacylase I (EC 3.5.1.14) is a homodimeric zinc binding metalloenzyme located in the cytosol, and it catalyzes the hydrolysis of N-linked acyl groups in L-amino acids, including the N-acetylated derivatives of serine, glutamic acid, alanine, methionine, glycine, leucine, and valine.Citation24 In mammal cells, the biological functions of ACY have been well documented mainly in N-acetylated amino acid metabolisms and its relationship to cell death. ACY3 efficiently deacetylates and detoxifies 4-hydroxy-2-nonenal and acrolein, which are highly reactive neurotoxic products of lipid peroxidation.Citation25 ACY3 deacetylates halogenated mercapturic acids, which are a cytotoxic agent in mice.Citation26 In contrast, transfection of ACY1 inhibited cell proliferation and increased apoptosis in renal cell carcinoma cell lines.Citation27 The ACYs are also shown to relate to intracellular catabolism of N-acetylated proteins that are generally assumed by the ubiquitin system. This proteolytic system is involved in fundamental biological functions such as the cell cycle, apoptosis, metabolism, signal transduction, immune response, and protein quality control.Citation28 Plant immune responses, including resistance gene-dependent HR, are also related to protein degradation via the ubiquitin system.Citation29 In this experiment, we observed acceleration of cell death by inoculation with P. infestans and Agrobacterium-expressing INF1- and NbMEK2DD in DS2 plants (, ). Therefore, we believe that DS2 may negatively regulate cell death and suppression of immune responses, resulting in establishing disease susceptibility. As far as we know, this is the first report showing the possible function of ACY in plants, especially as a disease susceptible factor. Furthermore, confirmation is needed of the functional analysis of DS2 and the functional link between DS2, SA, and suppression of plant immune responses (disease susceptibility).
Material and Methods
Plant growth conditions and pathogen inoculation
Nicotiana benthamiana, transgenic N. benthamiana expressing NahG, and N. tabacum were grown as previously described.Citation30Phytophthora infestans race 1.2.3.4 was maintained on susceptible potato (Solanum tuberosum) tubers.Citation31 Immature leaves of N. benthamiana are susceptible, whereas mature leaves of N. benthamiana are weakly susceptible and show basal resistance to P. infestans race 1.2.3.4.Citation31 A suspension of the zoospores was prepared as described by Yoshioka et al. Nicotiana benthamiana leaves were inoculated with a 0.3 ml drop of P. infestans zoospores (2 x 105 zoospores/ml) and covered with lens paper to disperse the zoospores. The inoculated leaves were kept at high humidity at 25 °C for 1 d after inoculation, and were then moved to a plant growth condition as described above. Disease development of late blight was evaluated daily using the following scoring index of disease symptoms: 0, no symptoms; 1, growing necrosis; 2, sporulation. At least 8 inoculated leaves from each plant were scored.Citation12
RNA and DNA isolation, and cDNA synthesis
Total RNA and DNA were isolated from N. benthamiana leaves with a NucleoSpin RNA plant kit in combination with an RNA/DNA Buffer Set (Macherey-Nagel, Düren, Germany) according to the manufacturer’s manual. Reverse transcription was performed with 1 µg total RNA using a PrimeScript RT reagent Kit (Takara Shuzo, Shiga, Japan).
Isolation of Full-Length cDNA
PCR amplification was performed with the primers DS2S (CATATGAGTT TTTGCGGCGG TAG) and DS2A (GGATCCTCAA TGATGATGAT GATGATGCAA TTCTTCCCTC GG). Cycling parameters were as follows: 30 cycles of 94 °C for 1 min, 55 °C for 1 min, and 72 °C for 1 min. The full-length cDNA was cloned into pGEMT-Easy (Promega), creating pGEM-DS2.
Sequencing
Sequencing analysis was performed using M4 (GTTTTCCCAG TCACGAC) and RV (CAGGAAACAG ATATGAC) primers with the reagents for the Big Dye Terminator Cycle Sequencing Kit (Applied Biosystems) and Applied Biosystems 3100 Avant Automated Sequencer (Applied Biosystems) according to the manufacturer’s instructions. The sequence analysis was performed using DNASIS (version 3.6; Hitachi) and the BLAST network service from the National Center for Biotechnology Information.
Quantitative real-time PCR
Expression levels of defense-related genes and the growth of P. infestans were determined by quantitative real-time PCR as described by Maimbo et al.Citation32 and Asai et al.,Citation33 respectively. Quantitative PCR was performed in a 20 µl reaction mixture containing 1 µl cDNA or DNA, and 10 pM respective primers using SYBR GreenER qPCR Reagent System (Invitrogen) on an Applied Biosystems 7300 real-time PCR instrument. The cycling parameters were the same for all primers: initial 50 °C for 2 min and 95 °C for 10 min, followed by 40 cycles of 95 °C for 10 s and 60 °C for 1 min. Primers used in this experiment were as follows: DS2rtpF (CCTTTCTCGG CCCACTTAGA) and DS2rtpR (GAGAACCCCG GGCGTAGA) for NbAC, PR1rtpF (GGTCAACACG GCGAAAACC) and PR1rtpR (GCCTTAGCAG CCGTCATGA) for PR-1, FboxrtpF (GGCACTCACA AACGTCTATT TC and FboxrtpR (ACCTGGGAGG CATCCTGCTT AT) for F-Box, L23rtpF (AAGGATGCCG TGAAGAAGAT GT) and L23rtpR (GCATCGTAGT CAGGAGTCAA CC) for L23, EF1rtpF (CCTCAAGAAG GTTGGATACA AC) and EF1rtpR (TCTTGGGCTC ATTAATCTGG TC) for NbEF-1α gene, and PirtpF (TGCACTGTGG AGGGCAAGT) and PirtpR (GCCCGAGGCC AAATTCA) for biomass of P. infestans. Melting curve runs were performed at the end of each PCR reaction to verify the specificity of primers by the presence of a single product. The relative amount of P. infestans genes was calculated using NbEF-1α as standard.Citation29 The normalization factor of plant gene expression was calculated as a geometric mean of F-box and L23 for each sample as shown previously.Citation34 Data are based on 3 independent biological replicates.
Virus-induced gene silencing
A 264 bp cDNA fragment of DS2 was amplified with primers DS2-PVX-S (AAAATAGCGT GCATCTGTCG ATGCAGGAAA) and DS2-PVX-A (AAAATAGCGT GCATCTGTCG ATGCAGGAAA). This cDNA fragment was subcloned into the TA cloning site of pGEM-T-Easy, creating pGEM-PVX::DS2. The pGEM-PVX::DS2 plasmid was digested with PstI and SalI, and ligated into the PVX vector pPVX201 digested with Sse8387I and SalI.Citation35 The construct containing this insert in the antisense orientation was designated as pPVX::DS2. The plasmids, pPVX201 (empty control) and pPPVX::DS2, were transformed into A. tumefaciens strain GV3101, and inoculated into N. benthamiana leaves as described previously,Citation13 and we used approximately 30-d-old silencing plants, which showed basal resistance (weakly susceptible) to P. infestans.Citation18
Trypan blue staining
Trypan blue staining was performed as described previouslyCitation18 with minor modifications. Inoculated leaves were boiled for 2 min in trypan blue staining solution (60 ml ethanol, 10 ml lactic acid, 10 ml glycerol, 10 ml phenol, 10 ml distilled water, 67 mg trypan blue). The leaves were decolorized in 2.5 g ml−1 chloral hydrate for at least 1 h. Stained leaves were observed using a fluorescence microscope (FSX100; Olympus).
Cell death quantification
Cell death was quantified by ion conductivity as described previously.Citation10 For ion leakage measurements, 6 leaf discs (8 mm in diameter) were collected from each plant and floated on 1 ml distilled water for 4 h at room temperature with gentle shaking. Conductivity of the water was measured using a twin conductivity meter (Twin Cond D-173; Horiba).
ROS measurement
ROS measurements were conducted as described by Kobayashi et al.Citation36 The relative intensity of ROS generation was determined by counting photons from L-012-mediated chemiluminescence. The L-012 probe (0.5 mM in 10 mM MOPS-KOH, pH 7.4) was infiltrated into N. benthamiana leaves using a needleless syringe. Chemiluminescence was monitored continuously using a photon image processor equipped with a sensitive CCD camera (LAS-4000 mini), and quantified with Multi Gauge ver. 3.0 software (Fujifilm, Tokyo, Japan).
Agrobacterium tumefaciens-mediated transient expression (agroinfiltration)
Agrobacterium tumefaciens strain GV3101 carrying GUS, INF1, R3a, AVR3a, NbMEK2DD were prepared as described previously.Citation37-Citation40 These constructs were transiently expressed in Nicotiana plants as described previously.Citation13
Creation of transgenic tobacco plants
The pGEM-DS2 was digested with BamHI and SacI (Takara Bio), and ligated into the pRI101 vector (Clontech, Tokyo, Japan) digested with the same enzymes. The construct containing the insert was designated as pRI::DS2. Tobacco plants (N. tabacum cv Samsun NN) aseptically grown from seed for approximately 1 mo were transformed with pRI::DS2 via the A. tumefaciens-mediated leaf disc procedureCitation41 and selected using 5 µg ml−1 kanamycin as the selection reagent. Genomic PCR was applied to confirm successful transformation with pRI::DS2 using primers based on CaMV35S promoter (35S-S) and NOS terminator (NOS-A) sequences and tobacco NbEF-1α primers as described previously.Citation10
Disclosure of Potential Conflicts of Interest
No potential conflicts of interest were disclosed.
Acknowledgments
The authors thank D Baulcombe for the pPVX201 vector, S Kamoun for AVR3a/R3a and INF1expression construct, and J Jones for NahG transgenic construct. This work was supported by Grants in Aid for Scientific Research to AK (16780031 and 18780029) and to YH (15028214 and 16380037) from the Ministry of Education, Culture, Sports, Science, and Technology, Japan and Grant-in-Aid from the Japan Society for the Promotion of Science Fellows to MN (25–7248). AK is also grateful for financial support from Asahi Glass foundation and Agricultural Chemical Foundation.
References
- Jones JD, Dangl JL. The plant immune system. Nature 2006; 444:323 - 9; http://dx.doi.org/10.1038/nature05286; PMID: 17108957
- Göhre V, Robatzek S. Breaking the barriers: microbial effector molecules subvert plant immunity. Annu Rev Phytopathol 2008; 46:189 - 215; http://dx.doi.org/10.1146/annurev.phyto.46.120407.110050; PMID: 18422429
- Zipfel C. Pattern-recognition receptors in plant innate immunity. Curr Opin Immunol 2008; 20:10 - 6; http://dx.doi.org/10.1016/j.coi.2007.11.003; PMID: 18206360
- Baker CJ, Orlandi EW. Active oxygen in plant pathogenesis. Annu Rev Phytopathol 1995; 33:299 - 321; http://dx.doi.org/10.1146/annurev.py.33.090195.001503; PMID: 18999963
- Levine A, Tenhaken R, Dixon R, Lamb C. H2O2 from the oxidative burst orchestrates the plant hypersensitive disease resistance response. Cell 1994; 79:583 - 93; http://dx.doi.org/10.1016/0092-8674(94)90544-4; PMID: 7954825
- Phytoalexins KJ. Annu Rev Phytopathol 1972; 10:207 - 32; http://dx.doi.org/10.1146/annurev.py.10.090172.001231
- Mauch F, Staehelin LA. Functional implications of the subcellular localization of ethylene-induced chitinase and β-1,3 glucanase in bean leaves. Plant Cell 1989; 1:447 - 57; PMID: 12359894
- Rushton PJ, Somssich IE. Transcriptional control of plant genes responsive to pathogens. Curr Opin Plant Biol 1998; 1:311 - 5; http://dx.doi.org/10.1016/1369-5266(88)80052-9; PMID: 10066598
- Yang Y, Shah J, Klessig DF. Signal perception and transduction in plant defense responses. Genes Dev 1997; 11:1621 - 39; http://dx.doi.org/10.1101/gad.11.13.1621; PMID: 9224713
- Nakano M, Nishihara M, Yoshioka H, Takahashi H, Sawasaki T, Ohnishi K, Hikichi Y, Kiba A. Suppression of DS1 phosphatidic acid phosphatase confirms resistance to Ralstonia solanacearum in Nicotiana benthamiana.. PLoS One 2013; 8:e75124; http://dx.doi.org/10.1371/journal.pone.0075124; PMID: 24073238
- Kamoun S, Vleeshouwers VG, Govers F, de Groot KE, Govers F, van West P, de Groot KE. Resistance of nicotiana benthamiana to phytophthora infestans is mediated by the recognition of the elicitor protein INF1. Plant Cell 1998; 10:1413 - 26; PMID: 9724689
- Lindner HA, Lunin VV, Alary A, Hecker R, Cygler M, Ménard R. Essential roles of zinc ligation and enzyme dimerization for catalysis in the aminoacylase-1/M20 family. J Biol Chem 2003; 278:44496 - 504; http://dx.doi.org/10.1074/jbc.M304233200; PMID: 12933810
- Kamoun S, Hamada W, Huitema E. Agrosuppression: a bioassay for the hypersensitive response suited to high-throughput screening. Mol Plant Microbe Interact 2003; 16:7 - 13; http://dx.doi.org/10.1094/MPMI.2003.16.1.7; PMID: 12580277
- Chaparro-Garcia A, Wilkinson RC, Gimenez-Ibanez S, Findlay K, Coffey MD, Zipfel C, Rathjen JP, Kamoun S, Schornack S. . The receptor-like kinase SERK3/BAK1 is required for basal resistance against the Late blight pathogen Phytophthora infestans in Nicotiana benthamiana. 2011; 6:e16608
- Asai S, Ohta K, Yoshioka H. MAPK signaling regulates nitric oxide and NADPH oxidase-dependent oxidative bursts in Nicotiana benthamiana.. Plant Cell 2008; 20:1390 - 406; http://dx.doi.org/10.1105/tpc.107.055855; PMID: 18515503
- Yoshida K, Schuenemann VJ, Cano LM, Pais M, Mishra B, Sharma R, Lanz C, Martin FN, Kamoun S, Krause J, et al. The rise and fall of the Phytophthora infestans lineage that triggered the Irish potato famine.. Elife 2013; 2:e00731; http://dx.doi.org/10.7554/eLife.00731; PMID: 23741619
- Katou S, Senda K, Yoshioka H, Doke N, Kawakita K. A 51 kDa protein kinase of potato activated with hyphal wall components from Phytophthora infestans.. Plant Cell Physiol 1999; 40:825 - 31; http://dx.doi.org/10.1093/oxfordjournals.pcp.a029611
- Yoshioka H, Numata N, Nakajima K, Katou S, Kawakita K, Rowland O, Jones JDG, Doke N. Nicotiana benthamiana gp91phox homologs NbrbohA and NbrbohB participate in H2O2 accumulation and resistance to Phytophthora infestans. Plant Cell 2003; 15:706 - 18; http://dx.doi.org/10.1105/tpc.008680; PMID: 12615943
- Eschen-Lippold L, Landgraf R, Smolka U, Schulze S, Heilmann M, Heilmann I, Hause G, Rosahl S. Activation of defense against Phytophthora infestans in potato by down-regulation of syntaxin gene expression. New Phytol 2012; 193:985 - 96; http://dx.doi.org/10.1111/j.1469-8137.2011.04024.x; PMID: 22243492
- Halim VA, Eschen-Lippold L, Altmann S, Birschwilks M, Scheel D, Rosahl S. Salicylic acid is important for basal defense of Solanum tuberosum against Phytophthora infestans.. Mol Plant Microbe Interact 2007; 20:1346 - 52; http://dx.doi.org/10.1094/MPMI-20-11-1346; PMID: 17977146
- Shibata Y, Kawakita K, Takemoto D. Age-related resistance of Nicotiana benthamiana against hemibiotrophic pathogen Phytophthora infestans requires both ethylene- and salicylic acid-mediated signaling pathways. Mol Plant Microbe Interact 2010; 23:1130 - 42; http://dx.doi.org/10.1094/MPMI-23-9-1130; PMID: 20687803
- Spoel SH, Johnson JS, Dong X. Regulation of tradeoffs between plant defenses against pathogens with different lifestyles. Proc Natl Acad Sci U S A 2007; 104:18842 - 7; http://dx.doi.org/10.1073/pnas.0708139104; PMID: 17998535
- Fry W. Phytophthora infestans: the plant (and R gene) destroyer. Mol Plant Pathol 2008; 9:385 - 402; http://dx.doi.org/10.1111/j.1364-3703.2007.00465.x; PMID: 18705878
- Giardina T, Biagini A, Dalle Ore F, Ferre E, Reynier M, Puigserver A. The hog intestinal mucosa acylase I: subcellular localization, isolation, kinetic studies and biological function. Biochimie 1997; 79:265 - 73; http://dx.doi.org/10.1016/S0300-9084(97)83514-6; PMID: 9258435
- Tsirulnikov K, Abuladze N, Bragin A, Faull K, Cascio D, Damoiseaux R, Schibler MJ, Pushkin A. Inhibition of aminoacylase 3 protects rat brain cortex neuronal cells from the toxicity of 4-hydroxy-2-nonenal mercapturate and 4-hydroxy-2-nonenal. Toxicol Appl Pharmacol 2012; 263:303 - 14; http://dx.doi.org/10.1016/j.taap.2012.07.002; PMID: 22819785
- Newman D, Abuladze N, Scholz K, Dekant W, Tsuprun V, Ryazantsev S, Bondar G, Sassani P, Kurtz I, Pushkin A. Specificity of aminoacylase III-mediated deacetylation of mercapturic acids. Drug Metab Dispos 2007; 35:43 - 50; http://dx.doi.org/10.1124/dmd.106.012062; PMID: 17012540
- Miller YE, Minna JD, Gazdar AF. Lack of expression of aminoacylase-1 in small cell lung cancer. Evidence for inactivation of genes encoded by chromosome 3p. J Clin Invest 1989; 83:2120 - 4; http://dx.doi.org/10.1172/JCI114125; PMID: 2542383
- Perrier J, Durand A, Giardina T, Puigserver A. Catabolism of intracellular N-terminal acetylated proteins: involvement of acylpeptide hydrolase and acylase. Biochimie 2005; 87:673 - 85; http://dx.doi.org/10.1016/j.biochi.2005.04.002; PMID: 15927344
- Marino D, Peeters N, Rivas S. Ubiquitination during plant immune signaling. Plant Physiol 2012; 160:15 - 27; http://dx.doi.org/10.1104/pp.112.199281; PMID: 22689893
- Kiba A, Tomiyama H, Takahashi H, Hamada H, Ohnishi K, Okuno T, Hikichi Y. Induction of resistance and expression of defense-related genes in tobacco leaves infiltrated with Ralstonia solanacearum.. Plant Cell Physiol 2003; 44:287 - 95; http://dx.doi.org/10.1093/pcp/pcg037; PMID: 12668775
- Yoshioka H, Miyabe M, Hayakawa Y, Doke N. Expression of genes for phenylalanine ammonia-lyase and 3-hydroxy-3-methylglutaryl CoA reductase in aged potato tubers infected with Phytophthora infestans.. Plant Cell Physiol 1996; 37:81 - 90; http://dx.doi.org/10.1093/oxfordjournals.pcp.a028917
- Maimbo M, Ohnishi K, Hikichi Y, Yoshioka H, Kiba A. Induction of a small heat shock protein and its functional roles in Nicotiana plants in the defense response against Ralstonia solanacearum.. Plant Physiol 2007; 145:1588 - 99; http://dx.doi.org/10.1104/pp.107.105353; PMID: 17965181
- Asai S, Ohta K, Yoshioka H. MAPK signaling regulates nitric oxide and NADPH oxidase-dependent oxidative bursts in Nicotiana benthamiana.. Plant Cell 2008; 20:1390 - 406; http://dx.doi.org/10.1105/tpc.107.055855; PMID: 18515503
- Liu D, Shi L, Han C, Yu J, Li D, Zhang Y. Validation of reference genes for gene expression studies in virus-infected Nicotiana benthamiana using quantitative real-time PCR. PLoS One 2012; 7:e46451; http://dx.doi.org/10.1371/journal.pone.0046451; PMID: 23029521
- Baulcombe DC, Chapman S, Santa Cruz S. Jellyfish green fluorescent protein as a reporter for virus infections. Plant J 1995; 7:1045 - 53; http://dx.doi.org/10.1046/j.1365-313X.1995.07061045.x; PMID: 7599646
- Kobayashi M, Ohura I, Kawakita K, Yokota N, Fujiwara M, Shimamoto K, Doke N, Yoshioka H. Calcium-dependent protein kinases regulate the production of reactive oxygen species by potato NADPH oxidase. Plant Cell 2007; 19:1065 - 80; http://dx.doi.org/10.1105/tpc.106.048884; PMID: 17400895
- Katou S, Yamamoto A, Yoshioka H, Kawakita K, Doke N. Functional analysis of potato mitogen-activated protein kinase kinase, StMEK1. J Gen Plant Pathol 2003; 69:161 - 8
- Kamoun S, Hamada W, Huitema E. Agrosuppression: a bioassay for the hypersensitive response suited to high-throughput screening. Mol Plant Microbe Interact 2003; 16:7 - 13; http://dx.doi.org/10.1094/MPMI.2003.16.1.7; PMID: 12580277
- Asai S, Mase K, Yoshioka H. A key enzyme for flavin synthesis is required for nitric oxide and reactive oxygen species production in disease resistance. Plant J 2010; 62:911 - 24; PMID: 20230506
- Yaeno T, Li H, Chaparro-Garcia A, Schornack S, Koshiba S, Watanabe S, Kigawa T, Kamoun S, Shirasu K. Phosphatidylinositol monophosphate-binding interface in the oomycete RXLR effector AVR3a is required for its stability in host cells to modulate plant immunity. Proc Natl Acad Sci U S A 2011; 108:14682 - 7; http://dx.doi.org/10.1073/pnas.1106002108; PMID: 21821794
- Horsch RB, Fry JE, Hoffman NL, Eichholtz D, Rogers SG, Fraley RT. A simple and general method for transferring genes into plants. Science 1985; 227:1229 - 31; http://dx.doi.org/10.1126/science.227.4691.1229; PMID: 17757866