Abstract
Plants sense environmental stimuli such as light to regulate their flowering time. In Arabidopsis, phytochrome B (phyB) is the major photoreceptor that perceives red and far-red light, and destabilizes transcriptional regulator CONSTANS (CO) protein. However the mechanism that links photoreceptor and CO protein degradation is largely unknown. We recently showed that PHYTOCHROME-DEPENDENT LATE-FLOWERING (PHL) protein inhibits phyB signaling through direct protein-protein interaction. Here, we report that light exposure destabilizes PHL protein as is the case with CO. Fluorescence from PHL-YFP fusion protein expressed under the control of Cauliflower Mosaic Virus (CaMV) 35S promoter (35S::PHL-YFP) almost disappeared after four-hour treatment of white light. Furthermore, the similar results were also obtained from the analysis of PHL-GUS fusion protein expressed by PHL promoter (PHLpro::PHL-GUS phl-1). These results highlight the importance of post-transcriptional regulation in phyB-mediated flowering regulation and will give us hints how phyB regulates CO protein amount.
Since light is one of the most important environmental signals in plants, various kinds of photoreceptors have evolved.Citation1 Among them, a red/far-red light photoreceptor phyB and several blue light photoreceptors regulate flowering time through modulating CO protein stability.Citation2-Citation4 However, how phyB regulates CO protein amount has remained unclear. Our recent work demonstrated that a novel protein, PHL, interacts with phyB in vitro and in vivo.Citation5 Furthermore, two phl mutant alleles cause late-flowering phenotype under long day (LD) but not under short day (SD) conditions, suggesting that PHL regulates flowering in the photoperiod pathway. Consistent with the view, FLOWERING LOCUS T (FT) expression under LD condition was suppressed in the phl mutant. These findings suggest that PHL have significant roles in flowering regulation by modulating phyB-signaling pathway. It was also demonstrated that the PHL could bridge interaction between phyB and CO protein in a red-light-dependent manner, implying that PHL protein may also undergo light-dependent destabilization, as does CO.Citation2
Here, we report that PHL protein is destabilized in response to light exposure. We first established a transgenic line that expresses PHL fused to YFP, under the control of the CaMV 35S promoter in the wild-type background (35S::PHL-YFP). Since the 35S::PHL-YFP line produced a 2-fold elevated PHL mRNA level, the line was expected to have only slight side effects of exogenous PHL-YFP (data not shown). In consistent with the low expression level of PHL-YFP, the transgenic line showed no significant phenotype both under LD and SD conditions (). To test the hypothesis that PHL is destabilized by light exposure, we observed fluorescence of PHL-YFP in dark- and light-grown seedlings. YFP fluorescence was observed in dark-grown seedlings, whereas significant fluorescence was not observed in light-grown seedlings (). Since the CaMV 35S promoter are active both under light and dark conditions, posttranscriptional regulation of PHL by light is strongly suggested. We then performed time-course observation of the PHL-YFP fluorescence. Dark-grown plants were transferred to continuous white light condition for 24 h. The intensity of PHL-YFP fluorescence was decreased in proportion to the time under continuous white light, and no significant fluoresce was observed after four-hour exposure to light ().
Figure 1. Flowering phenotype and protein stabilization of 35S::PHL-YFP. (A, B) Plants were grown under 16h light/8h dark long day and 8h light/16h dark short day conditions at 22 °C. Mean ± SD (n ≥ 12). (C) 35S::PHL-YFP were grown under continuous white light (cW), continuous dark (cD) for 7 d. Seedlings grown under cD were then exposed to white light for 1 to 24 h (cD + cW). YFP fluorescence was observed under a laser scanning confocal microscope. Bar = 50 µm
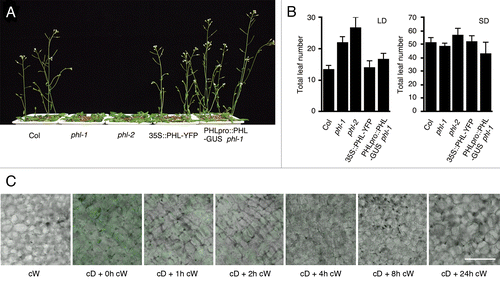
To confirm these observations, we also employed the PHLpro:PHL-GUS phl-1, which was used in our previous study.Citation5 PHL protein amount in seedlings was examined by staining for GUS (). In consistent with the observation from PHL-YFP, enough amount of PHL-GUS was detected in the dark grown seedlings, whereas PHL-GUS accumulation was not detected in the light grown seedlings (). Kinetics of PHL-GUS protein degradation was also comparable to that of PHL-YFP ( and ). Furthermore, accumulation of PHL was observed only in cotyledons even though the PHL mRNA expression has been detected in all organs tested ().Citation5
Figure 2. PHL-GUS stabilization under light and dark conditions. Ten-day-old PHLpro::PHL-GUS phl-1 plants grown under continuous white light (cW) and continuous dark (cD) (A) and 1 to 24h exposure of white light to the cD grown seedlings (B). Bar=1 mm
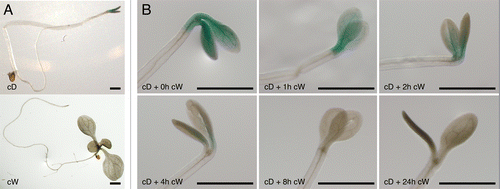
Through the time-course observation of PHL protein expressed as fusion proteins, we showed that PHL protein is destabilized by light exposure. Since phyB and PHL interact directly in a red-light-dependent manner, it is likely that PHL is degraded in response to red light. In support of this hypothesis, PHYTOCHROME ITNERACTING FACTORs (PIFs) and CO are also destabilized by red-light exposure, suggesting that these proteins are destabilized in a similar mechanism.Citation2,Citation6-Citation9 Previous studies demonstrated that an E3 ubiquitin ligase, CONSTITUTIVE PHOTOMORPHOGENIC 1 (COP1), is involved in the destabilization process of CO and PIF1.Citation7,Citation10,Citation11 Therefore, future study should involve the analysis of protein interaction between COP1 and PHL.
Interestingly, not only the PHLpro::PHL-GUS line but also the 35S::PHL-YFP line showed leaf-specific expression of PHL in the dark-grown seedlings, indicating the existence of an active destabilization mechanism of PHL presumably operating independently of phyB, although the biological meanings of the organ-specific degradation is unclear. Previous studies also demonstrated that phyB and CO regulate flowering by acting in leaves, supporting the existence of functional phyB-PHL-CO tripartite complex in leaves.Citation5,Citation12,Citation13
In conclusion, our study provides a new insight into the phyB-mediated and phyB-independent protein degradation system(s). Together with our recent findings, it is suggested that destabilization of PHL is an important step to modulate phyB signaling in the photoperiod pathway. Therefore, elucidation of molecular mechanism of PHL protein destabilization will help to understand how phyB regulates flowering by modulating CO protein amount.
Abbreviations: | ||
Cauliflower Mosaic Virus | = | CaMV |
CONSTANS | = | CO |
CONSTITUTIVE PHOTOMORPHOGENIC 1 | = | COP1 |
FLOWERING LOCUS T | = | FT |
long day | = | LD |
Phytochrome B | = | phyB |
PHYTOCHROME-DEPENDENT LATE-FLOWERING | = | PHL |
short day | = | SD |
Disclosure of Potential Conflicts of Interest
No potential conflicts of interest were disclosed.
Acknowledgments
This work was in part supported by a JSPS KAKENHI grant 22770036 (to M.E.), Grants-in-Aid for Scientific Research on Priority Areas 19060012 and 19060016 (to T.A.), and a Grant-in-Aid for Scientific Research on Innovative Areas 25113005 (to T.A.).
References
- Kami C, Lorrain S, Hornitschek P, Fankhauser C. Light-regulated plant growth and development. Curr Top Dev Biol 2010; 91:29 - 66; http://dx.doi.org/10.1016/S0070-2153(10)91002-8; PMID: 20705178
- Valverde F, Mouradov A, Soppe W, Ravenscroft D, Samach A, Coupland G. Photoreceptor regulation of CONSTANS protein in photoperiodic flowering. Science 2004; 303:1003 - 6; http://dx.doi.org/10.1126/science.1091761; PMID: 14963328
- Zuo Z, Liu H, Liu B, Liu X, Lin C. Blue light-dependent interaction of CRY2 with SPA1 regulates COP1 activity and floral initiation in Arabidopsis. Curr Biol 2011; 21:841 - 7; http://dx.doi.org/10.1016/j.cub.2011.03.048; PMID: 21514160
- Song YH, Smith RW, To BJ, Millar AJ, Imaizumi T. FKF1 conveys timing information for CONSTANS stabilization in photoperiodic flowering. Science 2012; 336:1045 - 9; http://dx.doi.org/10.1126/science.1219644; PMID: 22628657
- Endo M, Tanigawa Y, Murakami T, Araki T, Nagatani A. PHYTOCHROME-DEPENDENT LATE-FLOWERING accelerates flowering through physical interactions with phytochrome B and CONSTANS. Proc Natl Acad Sci U S A 2013; 110:18017 - 22; http://dx.doi.org/10.1073/pnas.1310631110; PMID: 24127609
- Park E, Kim J, Lee Y, Shin J, Oh E, Chung WI, Liu JR, Choi G. Degradation of phytochrome interacting factor 3 in phytochrome-mediated light signaling. Plant Cell Physiol 2004; 45:968 - 75; http://dx.doi.org/10.1093/pcp/pch125; PMID: 15356322
- Shen H, Moon J, Huq E. PIF1 is regulated by light-mediated degradation through the ubiquitin-26S proteasome pathway to optimize photomorphogenesis of seedlings in Arabidopsis.. Plant J 2005; 44:1023 - 35; http://dx.doi.org/10.1111/j.1365-313X.2005.02606.x; PMID: 16359394
- Oh E, Yamaguchi S, Kamiya Y, Bae G, Chung WI, Choi G. Light activates the degradation of PIL5 protein to promote seed germination through gibberellin in Arabidopsis.. Plant J 2006; 47:124 - 39; http://dx.doi.org/10.1111/j.1365-313X.2006.02773.x; PMID: 16740147
- Nozue K, Covington MF, Duek PD, Lorrain S, Fankhauser C, Harmer SL, Maloof JN. Rhythmic growth explained by coincidence between internal and external cues. Nature 2007; 448:358 - 61; http://dx.doi.org/10.1038/nature05946; PMID: 17589502
- Jang S, Marchal V, Panigrahi KC, Wenkel S, Soppe W, Deng XW, Valverde F, Coupland G. Arabidopsis COP1 shapes the temporal pattern of CO accumulation conferring a photoperiodic flowering response. EMBO J 2008; 27:1277 - 88; http://dx.doi.org/10.1038/emboj.2008.68; PMID: 18388858
- Laubinger S, Marchal V, Le Gourrierec J, Wenkel S, Adrian J, Jang S, Kulajta C, Braun H, Coupland G, Hoecker U. Arabidopsis SPA proteins regulate photoperiodic flowering and interact with the floral inducer CONSTANS to regulate its stability. Development 2006; 133:3213 - 22; http://dx.doi.org/10.1242/dev.02481; PMID: 16854975
- Zeevaart JAD. Physiology of flower formation. Annu Rev Plant Physiol 1976; 27:321 - 48; http://dx.doi.org/10.1146/annurev.pp.27.060176.001541
- Endo M, Nakamura S, Araki T, Mochizuki N, Nagatani A. Phytochrome B in the mesophyll delays flowering by suppressing FLOWERING LOCUS T expression in Arabidopsis vascular bundles. Plant Cell 2005; 17:1941 - 52; http://dx.doi.org/10.1105/tpc.105.032342; PMID: 15965119