Abstract
Arabidopsis plants transformed with a chimeric repressor for 6 transcription factors (TFs), including ADA2b, Msantd, DDF1, DREB26, AtGeBP, and ATHB23, that were converted by Chimeric REpressor gene Silencing Technology (CRES-T), show elevated salt and osmotic stress tolerance compared with wild type (WT) plants. However, the roles of TFs in salt and osmotic signaling remain largely unknown. Their hyper-osmotic stress tolerance was evaluated using 3 criteria: germination rate, root length, and rate of seedlings with visible cotyledons at the germination stage. All CRES-T lines tested exhibited better performance than WT, at least for one criterion under stress conditions. Under 600 mM mannitol stress, 3-week-old CRES-T lines accumulated proline, which is a major compatible solute involved in osmoregulation, at higher levels than WT. Expression levels of the delta 1-pyrroline-5-carboxylate synthase gene in CRES-T lines were similar to or lower than those in WT. In contrast, expression of the proline dehydrogenase (PDH) gene in DREB26-SRDX was significantly downregulated and that in ADA2b-SRDX and AtGeBP-SRDX was also rather downregulated compared with that in WT. Although plants at different stages were used for stress tolerance test and proline measurement in this study, we previously reported that 4 out of the 6 CRES-T lines showed better growth than WT after 4 weeks of incubation under 400 mM mannitol. These results suggest that proline accumulation caused by PDH gene suppression may be involved in enhanced osmotic stress tolerance in the CRES-T lines, and that these TFs may be involved in regulating proline metabolism in Arabidopsis.
To evaluate osmotic stress tolerance in the 6 CRES-T lines, T3 seeds from CRES-T lines and wild type (WT) plants were sown on 1/2 MS medium supplemented with 1% sucrose, 0.8% agar, and 500 or 600 mM mannitol, and the rate of germinating seeds was analyzed (). A seed was regarded as germinated when the radical protruded through the seed coat. In a previous study, all 6 CRES-T lines germinated earlier [by 3 d after sowing (DAS)] than WT (at 4 DAS) under a 400 mM mannitol stress, and the germination rates of both WT and all 6 CRES-T lines reached almost 100% by 5 DAS.Citation8 Under 500 mM mannitol, DDF1-SRDX, DREB26-SRDX, and ATHB23-SRDX showed significantly higher germination rates than WT at 5 DAS (). Under 600 mM mannitol, ADA2b-SRDX, Msanted-SRDX, DDF1-SRDX, and DREB26-SRDX showed significantly higher germination rates than WT at 10 DAS and later but AtGeBP-SRDX and ATHB23-SRDX did not (). Statistical significance was determined using unpaired the Student t-test at 0.05 probability level.
Figure 1. Osmotic stress tolerance in Chimeric REpressor gene Silencing Technology (CRES-T) lines. Germination rates (A, B, n = 10 × 3 sets), root length (C, D, n = 10), rate of seedlings with visible cotyledons (E, n = 10 × 3 sets), and appearance of seedlings (F) of wild-type (WT) and CRES-T lines (ADA2b-SRDX, Msantd-SRDX, DDF1-SRDX, DREB26-SRDX, AtGeBP-SRDX, and ATHB23-SRDX) were monitored on medium supplemented with 500 mM (A, C, E, F) and 600 mM (B, D, E) mannitol. A seed was regarded as germinated when the radical protruded through the seed coat. Cotyledons were regarded as visible when they appeared from the seed coat. Photographs were taken at 13 d after sowing. Only a representative photo is shown. Error bars represent standard errors. Scale = 1 cm. * and ** denote significant difference from WT at P < 0.05 and 0.01, respectively. ND, not detected.
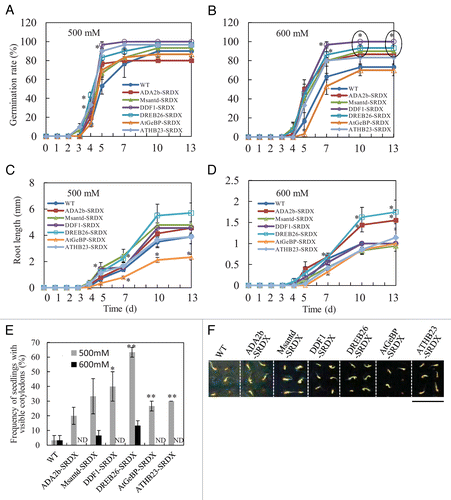
Root length of CRES-T lines and WT was measured during germination under osmotic stress conditions (). Petri dishes were vertically placed, and root length was measured using a ruler and a loupe. Under 500mM mannitol, DDF1-SRDX and DREB26-SRDX showed significantly better root growth than WT at 5 and 7 DAS, respectively, and AtGeBP-SRDX showed significantly shorter root length than WT (). Under 600 mM mannitol, ADA2b-SRDX and DREB26-SRDX showed significantly better root growth than WT at 7 DAS and later, and the other CRES-T lines showed comparable root growth to WT ().
Germination of CRES-T lines and WT was also evaluated by the rate of seedlings with visible cotyledons under stress conditions (). Cotyledons were regarded as visible when they appeared from the seed coat. Under 500 mM mannitol, appearance of visible cotyledons was observed significantly earlier in DDF1-SRDX, DREB26-SRDX, AtGeBP-SRDX, and ATHB23-SRDX than in WT. The other CERS-T lines also tended to show higher rates of seedlings, with visible cotyledons appearing at 13 DAS, though the results were not significant. Under 600 mM mannitol, Msantd-SRDX and DREB26-SRDX showed a higher rate of seedlings with visible cotyledons than WT. On medium without mannitol, no obvious difference was observed among the rates of WT and CRES-T lines.Citation8
Thus, the 6 CRES-T lines screened for salt tolerance conferred elevated osmotic stress tolerance compared with WT, and the DREB26-SRDX line showed the highest performance for all 3 criteria: germination rate, root length, and rate of seedlings with visible cotyledons at the germination stage under osmotic stress conditions.
To reveal the factors responsible for the enhanced osmotic tolerance, we determined their proline content, which is associated with osmoregulation.Citation9,Citation10 Three-week-old CRES-T lines and WT plants grown with vermiculite without stress were treated with a 600 mM mannitol solution, and the shoots were sampled before and after 4 d of treatment. The shoots were homogenized in a homogenizer and proline content was determined according to Bates et al. (1973).Citation11 ATHB23-SRDX accumulated proline at a significantly higher level than WT, and the other CRES-T lines, except for DDF1-SRDX, also tended to show higher proline content than WT, although the differences were not significant (). To determine the expression level of genes involved in proline metabolism, total RNAs were extracted from the mannitol-treated shoots, and the expression level of genes for delta 1-pyrroline-5-carboxylate synthase (P5CS) and proline dehydrogenase (PDH), which control the rate-limiting step of glutamate-derived biosynthesis and degradation of proline, respectively, were determined by quantitative reverse transcription-polymerase chain reaction (RT-PCR). The RT-PCR procedure and primer sequences for the P5CS gene were according to Kazama et al. (2013).Citation8 Primer sequences for the PDH gene were 5′-TGCTGACAAA GACCGACCAAT C-3′ and 5′-CACCAACTTG AACCCCATAG GAAC-3′. Expression levels of the P5CS gene in CRES-T lines were similar to those in WT, except for Msantd-SRDX, in which expression level was significantly lower (). In contrast, the PDH gene expression level in DREB26-SRDX was significantly downregulated and that in ADA2b-SRDX and AtGeBP-SRDX was also rather downregulated compared with that in WT (). Antisense suppression of PDH results in proline accumulation and improved tolerance to freezing and salinity in Arabidopsis.Citation12 Because plants at different stages were used for stress tolerance test and proline measurement in this study, it is not rational to unify enhanced stress tolerance and elevated proline accumulation in the CRES-T lines; however, we reported that ADA2b-SRDX, Msantd-SRDX, DDF1-SRDX, and DREB26-SRDX lines showed better growth than WT after 4 wk of incubation under 400 mM mannitol.Citation8 These results suggest that proline accumulation caused by PDH gene suppression may be involved, at least partially, in enhanced osmotic stress tolerance in the CRES-T lines DREB26-SRDX and ADA2b-SRDX and that these TFs may be involved in the regulation of proline metabolism. The reason why proline content in ATHB23-SRDX was higher than that in WT and why PDH gene expression in the line was higher than that in WT is unknown. However, post-translational regulation of the related enzymes or upregulation of other proline biosynthetic pathways may be responsible.
Figure 2. Proline content and delta 1-pyrroline-5-carboxylate synthase (P5CS) and proline dehydrogenase (PDH) gene expression in Chimeric REpressor gene Silencing Technology (CRES-T) lines. Proline contents (A) and expression of P5CS (B) and PDH (C) genes in wild type (WT) and CRES-T lines under 600 mM mannitol stress were determined. Error bars represent standard errors. *Significant difference from WT at p < 0.05.
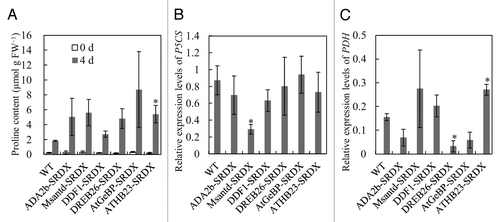
Disclosure of Potential Conflicts of Interest
No potential conflicts of interest were disclosed.
References
- Kasuga M, Liu Q, Miura S, Yamaguchi-Shinozaki K, Shinozaki K. Improving plant drought, salt, and freezing tolerance by gene transfer of a single stress-inducible transcription factor. Nat Biotechnol 1999; 17:287 - 91; http://dx.doi.org/10.1038/7036; PMID: 10096298
- Hu H, Dai M, Yao J, Xiao B, Li X, Zhang Q, Xiong L. Overexpressing a NAM, ATAF, and CUC (NAC) transcription factor enhances drought resistance and salt tolerance in rice. Proc Natl Acad Sci U S A 2006; 103:12987 - 92; http://dx.doi.org/10.1073/pnas.0604882103; PMID: 16924117
- Hu H, You J, Fang Y, Zhu X, Qi Z, Xiong L. Characterization of transcription factor gene SNAC2 conferring cold and salt tolerance in rice. Plant Mol Biol 2008; 67:169 - 81; http://dx.doi.org/10.1007/s11103-008-9309-5; PMID: 18273684
- Jung C, Seo JS, Han SW, Koo YJ, Kim CH, Song SI, Nahm BH, Choi YD, Cheong JJ. Overexpression of AtMYB44 enhances stomatal closure to confer abiotic stress tolerance in transgenic Arabidopsis. Plant Physiol 2008; 146:623 - 35; http://dx.doi.org/10.1104/pp.107.110981; PMID: 18162593
- Hiratsu K, Matsui K, Koyama T, Ohme-Takagi M. Dominant repression of target genes by chimeric repressors that include the EAR motif, a repression domain, in Arabidopsis. Plant J 2003; 34:733 - 9; http://dx.doi.org/10.1046/j.1365-313X.2003.01759.x; PMID: 12787253
- Hiratsu K, Ohta M, Matsui K, Ohme-Takagi M. The SUPERMAN protein is an active repressor whose carboxy-terminal repression domain is required for the development of normal flowers. FEBS Lett 2002; 514:351 - 4; http://dx.doi.org/10.1016/S0014-5793(02)02435-3; PMID: 11943180
- Mito T, Seki M, Shinozaki K, Ohme-Takagi M, Matsui K. Generation of chimeric repressors that confer salt tolerance in Arabidopsis and rice. Plant Biotechnol J 2011; 9:736 - 46; http://dx.doi.org/10.1111/j.1467-7652.2010.00578.x; PMID: 21114612
- Kazama D, Itakura M, Kurusu T, Mitsuda N, Ohme-Takagi M, Tada Y. Identification of chimeric repressors that confer salt and osmotic stress tolerance in Arabidopsis. Plants 2013; 2:769 - 85; http://dx.doi.org/10.3390/plants2040769
- Delauney A, Verma DPS. Proline biosynthesis and osmoregulation in plants. Plant J 1993; 4:215 - 23; http://dx.doi.org/10.1046/j.1365-313X.1993.04020215.x
- Hare PD, Cress WA, van Staden J. Proline synthesis and degradation: a model system for elucidating stress-related signal transduction. J Exp Bot 1999; 50:413 - 34
- Bates L, Waldren R, Teare I. Rapid determination of free proline for water-stress studies. Plant Soil 1973; 39:205 - 7; http://dx.doi.org/10.1007/BF00018060
- Nanjo T, Kobayashi M, Yoshiba Y, Kakubari Y, Yamaguchi-Shinozaki K, Shinozaki K. Antisense suppression of proline degradation improves tolerance to freezing and salinity in Arabidopsis thaliana.. FEBS Lett 1999; 461:205 - 10; http://dx.doi.org/10.1016/S0014-5793(99)01451-9; PMID: 10567698