Abstract
Arbuscular mycorrhizal symbiosis (AMS) and root nodule symbiosis (RNS) share several common symbiotic components, and many of the common symbiosis mutants block the entry of symbionts into the roots. We recently reported that CERBERUS (an E3 ubiquitin ligase) and NSP1 (a GRAS family transcription factor), required for RNS, also modulate AMS development in Lotus japonicus. The novel common symbiosis mutants, cerberus and nsp1, have low colonization of arbuscular mycorrhiza (AM) fungi, caused by a defect in internal hyphal elongation and by a decreased fungal entry into the roots, respectively. Here, we showed that CERBERUS was induced at the sites of symbiotic fungal or bacterial infection. NSP1 has been implicated in a strigolactone biosynthesis gene DWARF27 expression. Nevertheless, in nsp1, DWARF27 was induced by inoculation with AM fungi, implying the existence of a NSP1-independent regulatory mechanism of strigolactone biosynthesis during AMS establishment. These results support functional analysis of CERBERUS and NSP1, and also contribute to elucidation of common mechanisms in AMS and RNS.
Leguminous plants establish 2 major mutualistic plant–microbe interactions, arbuscular mycorrhizal symbiosis (AMS) with AM fungi and root nodule symbiosis (RNS) with rhizobia. AMS/RNS genes, referred to as “common symbiosis genes,” have been identified in the non-leguminous and leguminous plant species (SYMRK/DMI2, CASTOR and POLLUX/DMI1, NUP85, NUP133, NENA, CCaMK/DMI3 and CYCLOPS/IPD3).Citation1-Citation3 Many common symbiosis mutants have a defect in the early signaling pathway, which prevents symbiont recognition and blocks the entry of both fungal and bacterial symbionts into the host roots. Previously we reported 2 novel AMS mutants, cerberus and nsp1, by re-investigating in detail the AMS phenotypes of known Lotus japonicus symbiotic mutantsCitation4 which were thought to be RNS-specific. Our quantitative re-evaluation of AMS phenotypes showed that plants with cerberus or nsp1 had a significantly lower rate of infection by fungal symbionts than the wild-type. Phenotypes of the 2 mutants differed from each other and from any known L. japonicus AMS/RNS mutants. Phenotypic analysis revealed that CERBERUS (an E3 ubiquitin ligase) and NSP1 (a GRAS family transcription factor) are required for quantitative regulation of microbial infection through a system that may act in parallel with or later than the early symbiotic signaling pathway composed of the classical common symbiosis factors. In this study, we provide additional insights into the establishment of AMS and RNS through spatio-temporal analysis of CERBERUS expression at the early stages of both symbioses, and expression analysis of a strigolactone biosynthesis gene, regulated by NSP1 under nonsymbiotic condition,Citation5 during AMS in the nsp1 mutant.
CERBERUS (like its Medicago truncatula ortholog LIN) functions at the early steps of RNS. In cerberus/lin mutants, the development of infection threads in the root hairs is arrested.Citation6,Citation7 Additionally, the cerberus mutant has a defect in AM hyphal elongation, but apparently normal entry of AM hyphae into the host roots.Citation4 In RNS, strong expression of CERBERUS/LIN in nodule primordia and the infected zone of mature nodules has been reported.Citation6,Citation7 In the late stages of AMS, CERBERUS was constitutively expressed at low levels regardless of the presence of AM fungi.Citation4 Induction of CERBERUS was not detected during the early stages of either RNS or AMS by quantitative reverse transcription and real-time PCR (qRT-PCR) analysis in the whole root tissues.Citation4,Citation6 However, local induction of CERBERUS may be obtained in the cell periphery around the bacterial and fungal penetration sites, because CERBERUS regulates these early infection steps.Citation4,Citation6 To assess in which tissues CERBERUS is expressed, transgenic hairy roots expressing the β-glucuronidase gene (GUS) under the control of a CERBERUS promoter (CERBERUSpro:GUS) were infected with the rhizobium Mesorhizobium loti carrying DsRed or the AM fungus Rhizophagus irregularis and analyzed histochemically. In early stages of RNS, elevation of GUS activity was clearly detected in cells around the infection threads in hairy roots (). Unlike the constitutive expression of CERBERUS in whole roots at the late stages of AMS,Citation4 a weak GUS induction was often observed around the internal hyphae including penetration sites at earlier stages (). This analysis revealed that CERBERUS is locally and transiently expressed around the infection sites of both symbionts. These results are consistent with a function of CERBERUS at early stages of infection, presumably in promoting the elongation of rhizobial infection threads or internal AM fungal hyphae.
Figure 1.CERBERUS promoter activation in L. japonicus infected with the symbiotic rhizobium Mesorhizobium loti or the AM fungus Rhizophagus irregularis. Transgenic hairy roots expressing CERBERUSpro:GUS were infected with M. loti carrying DsRED (A, B) for 2 wk or R. irregularis for 3 wk (C-E). Histochemical staining and microscopy were performed as in ref. Citation4. Roots were stained with 5-bromo-4-chloro-3-indolyl β-d-glucuronide (X-gluc) to visualize GUS in the regions with CERBERUS promoter activity (A, C and D). M. loti was visualized by DsRed (B). Infection thread (boxed area) is magnified in inset. Arrowheads indicate GUS staining (A) and DsRED fluorescence (B) observed in epidermal cells around infection threads. AM fungal structures were visualized with wheat germ agglutinin (WGA) conjugated with Alexa Fluor 594 (E). Arrows indicate fungal infection sites (C–E). Weak GUS staining was observed around the internal AM hyphae including penetration sites (C, D). Bars = 200 μm (A-E) and 20 μm (B, inset).
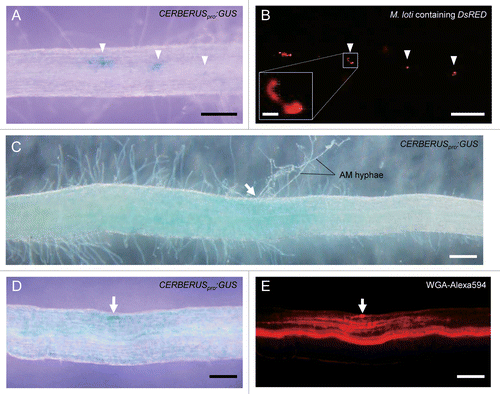
NSP1 and NSP2 belong to a family of plant-specific GRAS transcription factors, involved in root and shoot development, and in gibberellin- and light-mediated signaling. NSP1 and NSP2 are essential for RNSCitation8-Citation12 and control the expression of strigolactone biosynthesis genes, including DWARF27 required for AMS establishment.Citation4,Citation5,Citation13,Citation14 Strigolactones stimulate AM spore germination, hyphal growth, and branching through activation of AM fungal metabolism,Citation15-Citation17 and strigolactone-insensitive, strigolactone-deficient or putative strigolactone-export mutants exhibit AM-defective phenotypes.Citation18-Citation21 In Oryza sativa, DWARF27 is essential for strigolactone biosynthesis.Citation22 Earlier we reported that in uninfected roots of L. japonicus basal expression of DWARF27 was NSP1- and NSP2-dependent.Citation4 In this study, we investigated DWARF27 expression during AMS using qRT-PCR (). In the wild-type, DWARF27 expression was not affected by AM symbiont infection (). In non-inoculated roots of the nsp1 mutant, the DWARF27 transcript level was reduced to ~10% of the wild-type level (). In inoculated roots of the nsp1 mutant, the expression level of DWARF27 was still lower than that in wild-type roots (), suggesting that NSP1 affects basal expression levels of DWARF27 under both nonsymbiotic and symbiotic conditions. However, the DWARF27 transcript level in the inoculated nsp1 roots was significantly higher than that in non-inoculated nsp1 roots (3.1 fold-induction), implying existence of a NSP1-indepnedent regulatory mechanism of strigolactone biosynthesis during AMS establishment. Although strigolactones activate AM fungal growth,Citation15-Citation17 the low AM colonization of the nsp1 mutant was not only due to a decreased strigolactone biosynthesis, because AM colonization of nsp1 mutant was not rescued by treatment with exogenous strigolactoneCitation4 and the loss of NSP2, which also results in a decreased strigolactone biosynthesis,Citation4,Citation5 showed a normal AM fungal colonization.Citation4 In addition to its role in regulation of infection thread formation and nodule organogenesis in RNS,Citation8,Citation11 NSP1, but not NSP2, acts as a positive regulator of AMS in L. japonicus and should have a function in AM fungal infection other than regulation of strigolactone biosynthesis.
Figure 2.DWARF27 expression in wild-type (WT) and nsp1 roots during AM fungal infection. The DWARF27 transcript levels were analyzed 4 wk after inoculation with R. irregularis by qRT-PCR and are shown relative to that in uninfected WT plants. Error bars, SD (n = 3 or 4 biologically independent experiments). Asterisk indicates significant statistical difference (t test, P < 0.05).
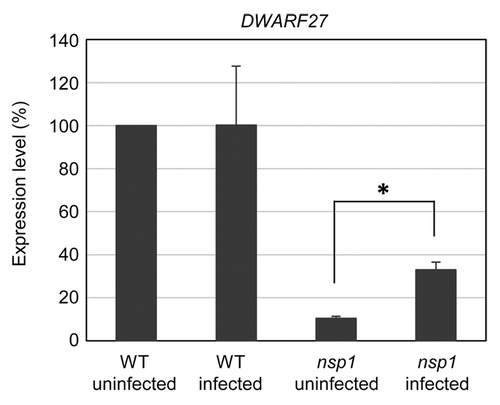
CERBERUS and NSP1 play a critical role in the early steps of RNS development, but they also regulate the establishment of AMS. This study, together with the recent identification of common symbiosis genes in M. truncatula,Citation13,Citation14,Citation23,Citation24 prompts a re-evaluation of classical models of the common symbiosis pathway and extension of the concept of commonality between RNS and AMS.
Abbreviations: | ||
AMS | = | arbuscular mycorrhizal symbiosis |
RNS | = | root nodule symbiosis |
AM | = | arbuscular mycorrhiza |
qRT-PCR | = | quantitative reverse transcription and real-time PCR |
GUS | = | β-glucuronidase |
Disclosure of Potential Conflicts of Interest
No potential conflicts of interest were disclosed.
Acknowledgments
This work is supported by the Japan Society of the Promotion of Science (No. 24770050) and Science and Technology Research Promotion Program for agriculture, forestry, fisheries, and food industry (No. 250023A).
References
- Parniske M. Arbuscular mycorrhiza: the mother of plant root endosymbioses. Nat Rev Microbiol 2008; 6:763 - 75; http://dx.doi.org/10.1038/nrmicro1987; PMID: 18794914
- Oldroyd GE, Harrison MJ, Paszkowski U. Reprogramming plant cells for endosymbiosis. Science 2009; 324:753 - 4; http://dx.doi.org/10.1126/science.1171644; PMID: 19423817
- Groth M, Takeda N, Perry J, Uchida H, Dräxl S, Brachmann A, Sato S, Tabata S, Kawaguchi M, Wang TL, et al. NENA, a Lotus japonicus homolog of Sec13, is required for rhizodermal infection by arbuscular mycorrhiza fungi and rhizobia but dispensable for cortical endosymbiotic development. Plant Cell 2010; 22:2509 - 26; http://dx.doi.org/10.1105/tpc.109.069807; PMID: 20675572
- Takeda N, Tsuzuki S, Suzaki T, Parniske M, Kawaguchi M. CERBERUS and NSP1 of Lotus japonicus are common symbiosis genes that modulate arbuscular mycorrhiza development. Plant Cell Physiol 2013; 54:1711 - 23; http://dx.doi.org/10.1093/pcp/pct114; PMID: 23926062
- Liu W, Kohlen W, Lillo A, Op den Camp R, Ivanov S, Hartog M, Limpens E, Jamil M, Smaczniak C, Kaufmann K, et al. Strigolactone biosynthesis in Medicago truncatula and rice requires the symbiotic GRAS-type transcription factors NSP1 and NSP2. Plant Cell 2011; 23:3853 - 65; http://dx.doi.org/10.1105/tpc.111.089771; PMID: 22039214
- Yano K, Shibata S, Chen WL, Sato S, Kaneko T, Jurkiewicz A, Sandal N, Banba M, Imaizumi-Anraku H, Kojima T, et al. CERBERUS, a novel U-box protein containing WD-40 repeats, is required for formation of the infection thread and nodule development in the legume-Rhizobium symbiosis. Plant J 2009; 60:168 - 80; http://dx.doi.org/10.1111/j.1365-313X.2009.03943.x; PMID: 19508425
- Kiss E, Oláh B, Kaló P, Morales M, Heckmann AB, Borbola A, Lózsa A, Kontár K, Middleton P, Downie JA, et al. LIN, a novel type of U-box/WD40 protein, controls early infection by rhizobia in legumes. Plant Physiol 2009; 151:1239 - 49; http://dx.doi.org/10.1104/pp.109.143933; PMID: 19776163
- Catoira R, Galera C, de Billy F, Penmetsa RV, Journet EP, Maillet F, Rosenberg C, Cook D, Gough C, Dénarié J. Four genes of Medicago truncatula controlling components of a nod factor transduction pathway. Plant Cell 2000; 12:1647 - 66; PMID: 11006338
- Smit P, Raedts J, Portyanko V, Debellé F, Gough C, Bisseling T, Geurts R. NSP1 of the GRAS protein family is essential for rhizobial Nod factor-induced transcription. Science 2005; 308:1789 - 91; http://dx.doi.org/10.1126/science.1111025; PMID: 15961669
- Kaló P, Gleason C, Edwards A, Marsh J, Mitra RM, Hirsch S, Jakab J, Sims S, Long SR, Rogers J, et al. Nodulation signaling in legumes requires NSP2, a member of the GRAS family of transcriptional regulators. Science 2005; 308:1786 - 9; http://dx.doi.org/10.1126/science.1110951; PMID: 15961668
- Heckmann AB, Lombardo F, Miwa H, Perry JA, Bunnewell S, Parniske M, Wang TL, Downie JA. Lotus japonicus nodulation requires two GRAS domain regulators, one of which is functionally conserved in a non-legume. Plant Physiol 2006; 142:1739 - 50; http://dx.doi.org/10.1104/pp.106.089508; PMID: 17071642
- Murakami Y, Miwa H, Imaizumi-Anraku H, Kouchi H, Downie JA, Kawaguchi M, Kawasaki S. Positional cloning identifies Lotus japonicus NSP2, a putative transcription factor of the GRAS family, required for NIN and ENOD40 gene expression in nodule initiation. DNA Res 2006; 13:255 - 65; http://dx.doi.org/10.1093/dnares/dsl017; PMID: 17244637
- Maillet F, Poinsot V, André O, Puech-Pagès V, Haouy A, Gueunier M, Cromer L, Giraudet D, Formey D, Niebel A, et al. Fungal lipochitooligosaccharide symbiotic signals in arbuscular mycorrhiza. Nature 2011; 469:58 - 63; http://dx.doi.org/10.1038/nature09622; PMID: 21209659
- Delaux PM, Bécard G, Combier JP. NSP1 is a component of the Myc signaling pathway. New Phytol 2013; 199:59 - 65; http://dx.doi.org/10.1111/nph.12340; PMID: 23663036
- Akiyama K, Matsuzaki K, Hayashi H. Plant sesquiterpenes induce hyphal branching in arbuscular mycorrhizal fungi. Nature 2005; 435:824 - 7; http://dx.doi.org/10.1038/nature03608; PMID: 15944706
- Besserer A, Puech-Pagès V, Kiefer P, Gomez-Roldan V, Jauneau A, Roy S, Portais JC, Roux C, Bécard G, Séjalon-Delmas N. Strigolactones stimulate arbuscular mycorrhizal fungi by activating mitochondria. PLoS Biol 2006; 4:e226; http://dx.doi.org/10.1371/journal.pbio.0040226; PMID: 16787107
- Besserer A, Bécard G, Jauneau A, Roux C, Séjalon-Delmas N. GR24, a synthetic analog of strigolactones, stimulates the mitosis and growth of the arbuscular mycorrhizal fungus Gigaspora rosea by boosting its energy metabolism. Plant Physiol 2008; 148:402 - 13; http://dx.doi.org/10.1104/pp.108.121400; PMID: 18614712
- Yoshida S, Kameoka H, Tempo M, Akiyama K, Umehara M, Yamaguchi S, Hayashi H, Kyozuka J, Shirasu K. The D3 F-box protein is a key component in host strigolactone responses essential for arbuscular mycorrhizal symbiosis. New Phytol 2012; 196:1208 - 16; http://dx.doi.org/10.1111/j.1469-8137.2012.04339.x; PMID: 23025475
- Gomez-Roldan V, Fermas S, Brewer PB, Puech-Pagès V, Dun EA, Pillot JP, Letisse F, Matusova R, Danoun S, Portais JC, et al. Strigolactone inhibition of shoot branching. Nature 2008; 455:189 - 94; http://dx.doi.org/10.1038/nature07271; PMID: 18690209
- Koltai H, LekKala SP, Bhattacharya C, Mayzlish-Gati E, Resnick N, Wininger S, Dor E, Yoneyama K, Yoneyama K, Hershenhorn J, et al. A tomato strigolactone-impaired mutant displays aberrant shoot morphology and plant interactions. J Exp Bot 2010; 61:1739 - 49; http://dx.doi.org/10.1093/jxb/erq041; PMID: 20194924
- Kretzschmar T, Kohlen W, Sasse J, Borghi L, Schlegel M, Bachelier JB, Reinhardt D, Bours R, Bouwmeester HJ, Martinoia E. A petunia ABC protein controls strigolactone-dependent symbiotic signalling and branching. Nature 2012; 483:341 - 4; http://dx.doi.org/10.1038/nature10873; PMID: 22398443
- Lin H, Wang R, Qian Q, Yan M, Meng X, Fu Z, Yan C, Jiang B, Su Z, Li J, et al. DWARF27, an iron-containing protein required for the biosynthesis of strigolactones, regulates rice tiller bud outgrowth. Plant Cell 2009; 21:1512 - 25; http://dx.doi.org/10.1105/tpc.109.065987; PMID: 19470589
- Pumplin N, Mondo SJ, Topp S, Starker CG, Gantt JS, Harrison MJ. Medicago truncatula Vapyrin is a novel protein required for arbuscular mycorrhizal symbiosis. Plant J 2010; 61:482 - 94; http://dx.doi.org/10.1111/j.1365-313X.2009.04072.x; PMID: 19912567
- Murray JD, Muni RR, Torres-Jerez I, Tang Y, Allen S, Andriankaja M, Li G, Laxmi A, Cheng X, Wen J, et al. Vapyrin, a gene essential for intracellular progression of arbuscular mycorrhizal symbiosis, is also essential for infection by rhizobia in the nodule symbiosis of Medicago truncatula.. Plant J 2011; 65:244 - 52; http://dx.doi.org/10.1111/j.1365-313X.2010.04415.x; PMID: 21223389