Abstract
Graminaceous plants release mugineic acid family phytosiderophores to acquire iron from the soil. Recently, we reported that particular vesicles are involved in deoxymugineic acid (DMA) and nicotianamine (NA) biosynthesis and in DMA secretion from rice roots. A fusion protein of rice NA synthase 2 (OsNAS2) and synthetic green fluorescent protein (sGFP) was observed in a dot-like pattern, moving dynamically within the cell. OsNAS2 mutated in the tyrosine motif or di-leucine motif, which was reported to be involved in cellular transport, caused a disruption in vesicular movement and vesicular localization, respectively. Unlike OsNAS2, Arabidopsis NA synthases AtNAS1–4 were distributed uniformly in the cytoplasm with no localization in dot-like structures when transiently expressed in tobacco BY-2 cells. Interestingly, Fe deficiency-inducible genes were upregulated in the OsNAS2-sGFP plants, and the amounts of NA and DMA produced and DMA secreted by the OsNAS2-sGFP plants were significantly higher than in those by the non-transformants and domain-mutated lines. We propose a model for OsNAS2-localized vesicles in rice, and discuss why the introduction of OsNAS2-sGFP caused a disturbance in Fe homeostasis.
Iron (Fe) is an essential nutrient for plant growth. Fe deficiency leads to leaf chlorosis, poor yields, and decreased nutritional quality. However, excess Fe is toxic because it catalyzes the generation of free radicals. Therefore, plants have developed sophisticated and tightly regulated mechanisms to acquire Fe from soil.Citation1
Graminaceous plants utilize a chelation strategy to acquire Fe from soil that involves the secretion of mugineic acid family phytosiderophores (MAs),Citation2,Citation3 which chelate and solubilize Fe(III) in the rhizosphere from their roots through Transporter Of Mugineic acids 1 (TOM1).Citation4,Citation5 The resultant Fe(III)-MAs complexes are absorbed by root cells through a transporter protein, Yellow Stripe 1 (YS1)/YS1-Like (YSL).Citation6,Citation7
Rice produces and secretes 2′-deoxymugineic acid (DMA), which is the first MAs to be biosynthesized. DMA is synthesized from S-adenosylmethionine (SAM) through a nicotianamine (NA) intermediateCitation8-Citation10 by 3 enzymes: NA synthase (NAS),Citation11 NA aminotransferase (NAAT),Citation12 and DMA synthase.Citation13 NA is a structural analog of MAs, and is responsible for metal homeostasis through metal translocation in plants.Citation14-Citation17
We investigated the diurnal rhythm of DMA secretion and vesicular involvement in DMA biosynthesis and secretion in rice.Citation18 We discovered that DMA secretion in rice fluctuated throughout the day under Fe-deficient and -sufficient conditions. In rice root cells, particular vesicles were found just before DMA secretion, which were similar to those reported in barley; these were designated as MAs-vesicles because of the putative site of MAs biosynthesis.Citation19,Citation20 In barley roots, enzymes responsible for MAs biosynthesis such as HvNAS1, HvNAAT-A, and HvDMAS1 are localized inside or close to these rER-derived vesicles (Nagasaka et al., unpublished data).Citation21 SAM is supplied by the methionine cycle in graminaceous plants,Citation22 and produced in the cytoplasm,Citation23 suggesting that SAM is imported to the MAs-vesicles by an unknown carrier and converted into NA and MAs in the MAs-vesicles. These vesicles in rice were increased in Fe-deficient roots, as compared with Fe-sufficient roots.Citation18 OsNAS2 fused to the N-terminus of synthetic green fluorescent protein (sGFP) was localized to similar vesicles. These results suggest that DMA biosynthesis in rice roots occurs in the vesicles (). These vesicles appear to originate from the rough endoplasmic reticulum (rER) because they are surrounded by ribosomes. It is thought that rER-derived vesicles are unable to fuse directly with the cell membrane. Therefore, it was speculated that NA and/or DMA might be transported from these vesicles into the cytoplasm through a specific transporter on the vesicle membrane; DMA and NA are then secreted from the cytoplasm to the rhizosphere and apoplasm through TOM1 and efflux transporter of NA 1 (ENA1),Citation4 respectively.
Figure 1. Models of the intracellular transport of MAs-vesicles in rice roots (A) and posttranscriptional mRNA degradation and/or posttranslational protein degradation of OsNAS2 (B). (A) MAs-biosynthetic enzymes, including OsNAS2, are located on/in MAs-vesicles derived from the rER, and DMA and/or NA are biosynthesized in these vesicles. The vesicles are transported via an actin-based system to the cell membrane. DMA and NA are transported from MAs-vesicles to the cytoplasm, and then secreted into the rhizosphere or apoplasm by TOM1 and ENA1, respectively. ARF was reported to be involved in the budding of vesicles; therefore, ARF may be involved in the budding of MAs-vesicles from the rER. These vesicles may be involved in DMA and/or NA transport to the vacuole for Fe sequestration, and VPS may be involved in this transport. (B) Under Fe-deficient conditions, many Fe deficiency-inducible genes, including OsNAS2, are upregulated at both the mRNA and protein levels. Under Fe-sufficient conditions, the mRNA or protein level must be kept low. Therefore, there may be a specific degradation system for these mRNAs and/or proteins. In OsNAS2-sGFP plants, this degradation may be disturbed, resulting in high-level NA production. NA may chelate Fe and induce Fe deficiency signaling.
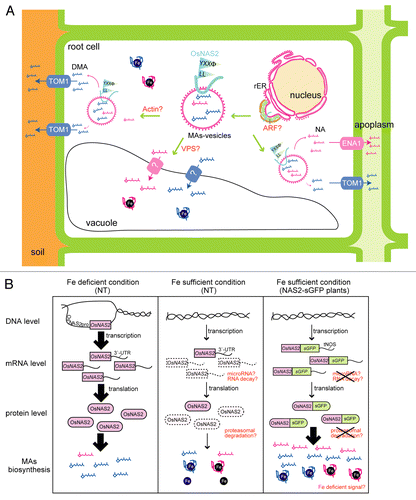
The OsNAS2-sGFP vesicles moved dynamically in the cells. OsNAS2 contains tyrosine (YXXφ) and di-leucine (LL) motifs, which are reported to be involved in cellular transport.Citation24,Citation25 OsNAS2 mutated in the YXXφ motif (m6-sGFP) was localized to the vesicles; however, these vesicles stuck together and were immobile.Citation18 On the other hand, OsNAS2 mutated in the LL motif (m7-sGFP) did not localize to these vesicles. In vitro, both OsNAS2-sGFP and m6-sGFP showed enzymatic activity; however, m7-sGFP did not. These results suggest that the tyrosine motif is involved in vesicle movement, whereas the di-leucine motif is involved in vesicle localization and OsNAS2 activity, which are crucial for the proper function of OsNAS2. NAS is predicted to contain 2 transmembrane domains, implying that it is integrated into the membrane of these vesicles. The YXXφ and LL motifs exist within the N-tail of OsNAS2, which might be located in the cytoplasm. An oligo-microarray analysis revealed that the expression of actin-binding proteins, vacuolar protein-sorting protein (VPS) 45 homolog, and ADP-ribosylation factor (ARF) differed between OsNAS2-sGFP and m6-sGFP plants. These factors may interact with the YXXφ motif and be involved in the movement of OsNAS2-sGFP vesicles ().
Interestingly, we found that Fe homeostasis was disturbed in the OsNAS2-sGFP plants, and that these plants received Fe-deficiency signals even under Fe-sufficient conditions.Citation18 Our oligo-microarray analysis showed that many genes whose expression levels were changed by the Fe nutrient status in non-transformants (NTs) were constitutively expressed in the roots of OsNAS2-sGFP plants, regardless of the availability of Fe. Consistent with these results, the amount of DMA secreted from the OsNAS2-sGFP plants was extremely high under Fe-sufficient conditions. In addition, there was dramatically more NA and DMA in the OsNAS2-sGFP plants than in the NT, m6-sGFP, and m7-sGFP plants. Our results suggest that NA accumulation caused by the introduction of OsNAS2-sGFP enabled the de-repression of genes induced by Fe deficiency, and further increased the NA and DMA levels in the OsNAS2-sGFP plants.
The reason for the disturbance in Fe homeostasis in the OsNAS2-sGFP plants might be related to the overproduction of DMA and NA, which increases the chelating capacity of Fe and disrupts an unknown Fe-sensing mechanism (). Recently, a network of transcription factors that regulate the Fe-deficiency response have begun to be clarified; these appear to be only partially conserved among non-graminaceous and graminaceous plants.Citation26 In rice, cis-acting Fe deficiency-responsive element (IDE)-binding factor 1 (IDEF1) was proposed as a Fe sensor.Citation27,Citation28 IDEF1 protein directly binds to Fe and other divalent metals, raising the possibility that IDEF1 itself might sense Fe status in plant cells. Overproduction of NA and/or DMA might interfere the binding of Fe to IDEF1 or other unidentified Fe sensors.
NA has long been considered a candidate long-distance Fe signaling molecule in both graminaceous plants and dicots,Citation29 but this has not been proven. In rice, NAS overexpression positively modulate Fe homeostasis-related genes.Citation30 NA accumulation in Osnaat1 mutants also triggers a constitutive Fe-deficiency response.Citation31 In Arabidopsis, it was reported that NA in excess paradoxically leads to Fe deficiency, suggesting that an increase of the NA apoplastic pool sequestered Fe and extracellular NA in the apoplast could control plant Fe homeostasis.Citation32 Overexpression of ZINC-INDUCED FACILITATOR1 (ZIF1) increase the amount of NA both in the roots and shoots and leads to Fe deficiency.Citation33 ZIF1 is a vacuolar membrane localized putative transporter required for Zn tolerance, and hypothesized to transport NA from cytoplasm into vacuoles. It was suggested that perturbing the subcellular distribution of the NA has profound effects on Fe with respect to their subcellular distribution and interorgan partitioning. Regulation of the subcellular distribution of Fe is important for maintaining Fe homeostasis.Citation26 The mobilization of vacuolar Fe pool by Natural Resistance-Associated Macrophage Protein 3, 4 (AtNRAMP3, 4) is necessary for seed germination under Fe deficient condition in Arabidopsis.Citation34 The membrane-spanning protein PIC1 (for permease in chloroplasts 1) is tightly linked to Fe storage and Fe homeostasis through regulating the Fe level in chloroplasts.Citation35 Fe transport into mitochondria by Mitochondrial Fe transporter (MIT) is essential for plant growth and development.Citation36 In our results, Fe homeostasis was disturbed in the OsNAS2-sGFP plants, but not in the NT, m6-sGFP, and m7-sGFP plants.Citation18 These results may suggest that NA and DMA need to be synthesized and stored in vesicles and transported to adequate compartment in the cell to prevent interferences with Fe deficiency signaling. Indeed, in pea and tomato, it was immunochemically investigated that NA level in vacuole and Fe concentration paralleled, indicating the possible role of NA in the vacuolar sequestration for the detoxification of excess Fe.Citation37 Recently, high resolution secondary ion mass spectrometry (NanoSIMS) technology clearly revealed the subcellular localization of micronutrients including Fe.Citation38 It was hypothesized by analysis of the OsNAS2-overexpression rice grain with NanoSIMS that Fe in rice grain could be bound not only to phytic acid but also to NA and/or DMA.Citation39 Further analysis of the cellular localization of NA in OsNAS2-sGFP rice by immunohistochemistry with an antibody against NA and NanoSIMS could prove our hypothesis that NA and/or DMA in the vesicles are involved in Fe homeostasis.
Dicot plants produce NA but not MAs; NA is a final product in dicot. On the other hand, in graminaceous plants, NA works not only as a chelator for internal transport of metals but also as the intermediate of MAs. Therefore, physiological functions of AtNASs and OsNAS2 are not identical. Previously, we confirmed the enzyme activities of AtNAS1–3 in vitro.Citation40 To gain further insight into physiological functions of NASs, we analyzed subcellular localization of AtNAS1–4 by transient expression of sGFP fusions in tobacco BY-2 cells (). Unlike OsNAS2, all of AtNAS1–4 were distributed uniformly in the cytoplasm with no localization in dot-like structures. We also analyzed subcellular localization of maize NASs (ZmNAS1–3) previouosly.Citation42 ZmNAS1 and 2 fused to sGFP localized to the dot-like strictures but ZmNAS3-sGFP distributed uniformly in the cytoplasm similarly to AtNAS1–4. These differences in subcellular localization of NASs may reflect their physiological roles.
Figure 2. Subcellular localization of AtNAS-sGFP fusion proteins in tobacco BY-2 cells. Shown are tobacco BY-2 cells, which transiently express sGFP (A), AtNAS1-sGFP (B), AtNAS2-sGFP (C), AtNAS3-sGFP (D) and AtNAS4-sGFP (E)fusion proteins under the control of the CaMV 35S promoter, observed by confocal fluorescence microscopy. Transient expression in tobacco BY-2 cells was performed as described previously.Citation41 Scale bar represents 10 μm.
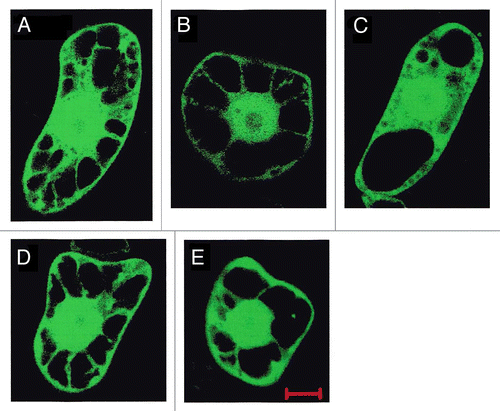
The OsNAS2-sGFP plants grew more slowly than the NT, m6-sGFP, and m7-sGFP plants. The Fe concentration in the shoots of the OsNAS2-sGFP plants was higher than that in the NT, m6-sGFP, and m7-sGFP plants. Since the endogenous amount of NA in roots of the OsNAS2-sGFP plants was high even under Fe-sufficient condition, we speculated that repression or degradation of OsNAS2 under Fe-sufficient conditions was disturbed in these plants (). It is possible that posttranscriptional mRNA degradation and/or posttranslational protein degradation repressed OsNAS2 accumulation under Fe-sufficient conditions in the NTs. To maintain Fe homeostasis, RNA degradation by mRNA decay or microRNAs is reportedly involved in regulating the mRNA expression of Fe homeostasis-related genes.Citation43,Citation44 Recently, Oryza sativa Haemerythrin motif-containing Really Interesting New Gene (RING)-and Zinc-finger proteins (OsHRZs) were reported to be Fe-binding ubiquitin ligases that negatively regulate Fe acquisition under Fe-sufficient conditions.Citation45 It is possible that ubiquitin-mediated degradation by OsHRZs is involved in OsNAS2 repression under Fe-sufficient conditions. Additional studies of OsNAS2 degradation will provide further insight into the regulation of Fe homeostasis.
Disclosure of Potential Conflicts of Interest
No potential conflicts of interest were disclosed.
References
- Marschner H. Mineral nutrition of higher plants. London: Academic Press, 1995.
- Takagi S. Naturally occurring iron-chelating compounds in oat-and rice-root washings: I. Activity measurement and preliminary characterization. Soil Sci Plant Nutr 1976; 22:423 - 33; http://dx.doi.org/10.1080/00380768.1976.10433004
- Takagi SI, Nomoto K, Takemoto T. Physiological aspect of mugineic acid, a possible phytosiderophore of graminaceous plants. J Plant Nutr 1984; 7:469 - 77; http://dx.doi.org/10.1080/01904168409363213
- Nozoye T, Nagasaka S, Kobayashi T, Takahashi M, Sato Y, Sato Y, Uozumi N, Nakanishi H, Nishizawa NK. Phytosiderophore efflux transporters are crucial for iron acquisition in graminaceous plants. J Biol Chem 2011; 286:5446 - 54; http://dx.doi.org/10.1074/jbc.M110.180026; PMID: 21156806
- Nozoye T, Inoue H, Takahashi M, Ishimaru Y, Nakanishi H, Mori S, Nishizawa NK. The expression of iron homeostasis-related genes during rice germination. Plant Mol Biol 2007; 64:35 - 47; http://dx.doi.org/10.1007/s11103-007-9132-4; PMID: 17333504
- Curie C, Panaviene Z, Loulergue C, Dellaporta SL, Briat J-F, Walker EL. Maize yellow stripe1 encodes a membrane protein directly involved in Fe(III) uptake. Nature 2001; 409:346 - 9; http://dx.doi.org/10.1038/35053080; PMID: 11201743
- Inoue H, Kobayashi T, Nozoye T, Takahashi M, Kakei Y, Suzuki K, Nakazono M, Nakanishi H, Mori S, Nishizawa NK. Rice OsYSL15 is an iron-regulated iron(III)-deoxymugineic acid transporter expressed in the roots and is essential for iron uptake in early growth of the seedlings. J Biol Chem 2009; 284:3470 - 9; http://dx.doi.org/10.1074/jbc.M806042200; PMID: 19049971
- Mori S, Nishizawa N. Methionine as a dominant precursor of phytosiderophores in graminaceae plants. Plant Cell Physiol 1987; 28:1081 - 92
- Shojima S, Nishizawa NK, Mori S. Establishment of a cell-free system for the biosynthesis of nicotianamine. Plant Cell Physiol 1989; 30:673 - 7
- Shojima S, Nishizawa NK, Fushiya S, Nozoe S, Irifune T, Mori S. Biosynthesis of phytosiderophores: In vitro biosynthesis of 2'-deoxymugineic acid from L-methionine and nicotianamine. Plant Physiol 1990; 93:1497 - 503; http://dx.doi.org/10.1104/pp.93.4.1497; PMID: 16667646
- Higuchi K, Suzuki K, Nakanishi H, Yamaguchi H, Nishizawa NK, Mori S. Cloning of nicotianamine synthase genes, novel genes involved in the biosynthesis of phytosiderophores. Plant Physiol 1999; 119:471 - 80; http://dx.doi.org/10.1104/pp.119.2.471; PMID: 9952442
- Takahashi M, Yamaguchi H, Nakanishi H, Shioiri T, Nishizawa NK, Mori S. Cloning two genes for nicotianamine aminotransferase, a critical enzyme in iron acquisition (Strategy II) in graminaceous plants. Plant Physiol 1999; 121:947 - 56; http://dx.doi.org/10.1104/pp.121.3.947; PMID: 10557244
- Bashir K, Inoue H, Nagasaka S, Takahashi M, Nakanishi H, Mori S, Nishizawa NK. Cloning and characterization of deoxymugineic acid synthase genes from graminaceous plants. J Biol Chem 2006; 281:32395 - 402; http://dx.doi.org/10.1074/jbc.M604133200; PMID: 16926158
- Beneš I, Schreiber K, Ripperger H, Kircheiss A. Metal complex formation by nicotianamine, a possible phytosiderophore. Cell Mol Life Sci 1983; 39:261 - 2; http://dx.doi.org/10.1007/BF01955293
- Hell R, Stephan UW. Iron uptake, trafficking and homeostasis in plants. Planta 2003; 216:541 - 51; PMID: 12569395
- Takahashi M, Terada Y, Nakai I, Nakanishi H, Yoshimura E, Mori S, Nishizawa NK. Role of nicotianamine in the intracellular delivery of metals and plant reproductive development. Plant Cell 2003; 15:1263 - 80; http://dx.doi.org/10.1105/tpc.010256; PMID: 12782722
- Schuler M, Rellán-Álvarez R, Fink-Straube C, Abadía J, Bauer P. Nicotianamine functions in the Phloem-based transport of iron to sink organs, in pollen development and pollen tube growth in Arabidopsis. Plant Cell 2012; 24:2380 - 400; http://dx.doi.org/10.1105/tpc.112.099077; PMID: 22706286
- Nozoye T, Nagasaka S, Bashir K, Takahashi M, Kobayashi T, Nakanishi H, Nishizawa NK. Nicotianamine synthase 2 localizes to the vesicles of iron-deficient rice roots, and its mutation in the YXXφ or LL motif causes the disruption of vesicle formation or movement in rice. Plant J 2014; 77:246 - 60; http://dx.doi.org/10.1111/tpj.12383
- Nishizawa N, Mori S. The particular vesicle appearing in barley root cells and its relation to mugineic acid secretion. J Plant Nutr 1987; 10:1013 - 20; http://dx.doi.org/10.1080/01904168709363629
- Negishi T, Nakanishi H, Yazaki J, Kishimoto N, Fujii F, Shimbo K, Yamamoto K, Sakata K, Sasaki T, Kikuchi S, et al. cDNA microarray analysis of gene expression during Fe-deficiency stress in barley suggests that polar transport of vesicles is implicated in phytosiderophore secretion in Fe-deficient barley roots. Plant J 2002; 30:83 - 94; http://dx.doi.org/10.1046/j.1365-313X.2002.01270.x; PMID: 11967095
- Nagasaka S, Takahashi M, Nakanishi H, Mori S, Nishizawa NK. Morphological and immunocytochemical analysis on synthesis and secretion of Mas in Hordeum vulgare. In Abstracts, XII International Symposium on Iron Nutrition and Interactions in Plants, Tokyo, Japan, p. 238.
- Suzuki M, Takahashi M, Tsukamoto T, Watanabe S, Matsuhashi S, Yazaki J, Kishimoto N, Kikuchi S, Nakanishi H, Mori S, et al. Biosynthesis and secretion of mugineic acid family phytosiderophores in zinc-deficient barley. Plant J 2006; 48:85 - 97; http://dx.doi.org/10.1111/j.1365-313X.2006.02853.x; PMID: 16972867
- Ravanel S, Block MA, Rippert P, Jabrin S, Curien G, Rébeillé F, Douce R. Methionine metabolism in plants: chloroplasts are autonomous for de novo methionine synthesis and can import S-adenosylmethionine from the cytosol. J Biol Chem 2004; 279:22548 - 57; http://dx.doi.org/10.1074/jbc.M313250200; PMID: 15024005
- Zuo J, Niu Q-W, Nishizawa N, Wu Y, Kost B, Chua N-H. KORRIGAN, an Arabidopsis endo-1,4-β-glucanase, localizes to the cell plate by polarized targeting and is essential for cytokinesis. Plant Cell 2000; 12:1137 - 52; PMID: 10899980
- Robert S, Bichet A, Grandjean O, Kierzkowski D, Satiat-Jeunemaître B, Pelletier S, Hauser M-T, Höfte H, Vernhettes S. An Arabidopsis endo-1,4-β-D-glucanase involved in cellulose synthesis undergoes regulated intracellular cycling. Plant Cell 2005; 17:3378 - 89; http://dx.doi.org/10.1105/tpc.105.036228; PMID: 16284310
- Kobayashi T, Nishizawa NK. Iron uptake, translocation, and regulation in higher plants. Annu Rev Plant Biol 2012; 63:131 - 52; http://dx.doi.org/10.1146/annurev-arplant-042811-105522; PMID: 22404471
- Kobayashi T, Ogo Y, Itai RN, Nakanishi H, Takahashi M, Mori S, Nishizawa NK. The transcription factor IDEF1 regulates the response to and tolerance of iron deficiency in plants. Proc Natl Acad Sci U S A 2007; 104:19150 - 5; http://dx.doi.org/10.1073/pnas.0707010104; PMID: 18025467
- Kobayashi T, Itai RN, Aung MS, Senoura T, Nakanishi H, Nishizawa NK. The rice transcription factor IDEF1 directly binds to iron and other divalent metals for sensing cellular iron status. Plant J 2012; 69:81 - 91; http://dx.doi.org/10.1111/j.1365-313X.2011.04772.x; PMID: 21880076
- Curie C, Briat JF. Iron transport and signaling in plants. Annu Rev Plant Biol 2003; 54:183 - 206; http://dx.doi.org/10.1146/annurev.arplant.54.031902.135018; PMID: 14509968
- Wang M, Gruissem W, Bhullar NK. Nicotianamine synthase overexpression positively modulates iron homeostasis-related genes in high iron rice. Front Plant Sci 2013; 4:156; http://dx.doi.org/10.3389/fpls.2013.00156; PMID: 23755054
- Cheng L, Wang F, Shou H, Huang F, Zheng L, He F, Li J, Zhao FJ, Ueno D, Ma JF, et al. Mutation in nicotianamine aminotransferase stimulated the Fe(II) acquisition system and led to iron accumulation in rice. Plant Physiol 2007; 145:1647 - 57; http://dx.doi.org/10.1104/pp.107.107912; PMID: 17951455
- Cassin G, Mari S, Curie C, Briat JF, Czernic P. Increased sensitivity to iron deficiency in Arabidopsis thaliana overaccumulating nicotianamine. J Exp Bot 2009; 60:1249 - 59; http://dx.doi.org/10.1093/jxb/erp007; PMID: 19188276
- Haydon MJ, Kawachi M, Wirtz M, Hillmer S, Hell R, Krämer U. Vacuolar nicotianamine has critical and distinct roles under iron deficiency and for zinc sequestration in Arabidopsis. Plant Cell 2012; 24:724 - 37; http://dx.doi.org/10.1105/tpc.111.095042; PMID: 22374397
- Lanquar V, Ramos MS, Lelièvre F, Barbier-Brygoo H, Krieger-Liszkay A, Krämer U, Thomine S. Export of vacuolar manganese by AtNRAMP3 and AtNRAMP4 is required for optimal photosynthesis and growth under manganese deficiency. Plant Physiol 2010; 152:1986 - 99; http://dx.doi.org/10.1104/pp.109.150946; PMID: 20181755
- Duy D, Stübe R, Wanner G, Philippar K. The chloroplast permease PIC1 regulates plant growth and development by directing homeostasis and transport of iron. Plant Physiol 2011; 155:1709 - 22; http://dx.doi.org/10.1104/pp.110.170233; PMID: 21343424
- Bashir K, Ishimaru Y, Shimo H, Nagasaka S, Fujimoto M, Takanashi H, Tsutsumi N, An G, Nakanishi H, Nishizawa NK. The rice mitochondrial iron transporter is essential for plant growth. Nat Commun 2011; 2:322; http://dx.doi.org/10.1038/ncomms1326; PMID: 21610725
- Pich A, Manteuffel R, Hillmer S, Scholz G, Schmidt W. Fe homeostasis in plant cells: does nicotianamine play multiple roles in the regulation of cytoplasmic Fe concentration?. Planta 2001; 213:967 - 76; http://dx.doi.org/10.1007/s004250100573; PMID: 11722133
- Zhao FJ, Moore KL, Lombi E, Zhu YG. Imaging element distribution and speciation in plant cells. Trends Plant Sci 2014; 19:183 - 92; http://dx.doi.org/10.1016/j.tplants.2013.12.001; PMID: 24394523
- Kyriacou B, Moore KL, Paterson D, Jonge MD, Howard DL, Stangoulis J, Tester M, Lombi E, Johnson AAT. J Cereal Sci 2014; 59:173 - 80; http://dx.doi.org/10.1016/j.jcs.2013.12.006
- Suzuki K, Higuchi K, Nakanishi H, Nishizawa NK, Mori S. Cloning of nicotianamine synthase genes from Arabidopsis thaliana. Soil Sci Plant Nutr 1999; 45:993 - 1002; http://dx.doi.org/10.1080/00380768.1999.10414350
- Yamaguchi H, Nishizawa NK, Nakanishi H, Mori S. IDI7, a new iron-regulated ABC transporter from barley roots, localizes to the tonoplast. J Exp Bot 2002; 53:727 - 35; http://dx.doi.org/10.1093/jexbot/53.369.727; PMID: 11886893
- Mizuno D, Higuchi K, Sakamoto T, Nakanishi H, Mori S, Nishizawa NK. Three nicotianamine synthase genes isolated from maize are differentially regulated by iron nutritional status. Plant Physiol 2003; 132:1989 - 97; http://dx.doi.org/10.1104/pp.102.019869; PMID: 12913155
- Ravet K, Reyt G, Arnaud N, Krouk G, Djouani B, Boucherez J, Briat J-F, Gaymard F. Iron and ROS control of the DownSTream mRNA decay pathway is essential for plant fitness. EMBO J 2012; 31:175 - 86; http://dx.doi.org/10.1038/emboj.2011.341; PMID: 21946558
- Waters BM, McInturf SA, Stein RJ. Rosette iron deficiency transcript and microRNA profiling reveals links between copper and iron homeostasis in Arabidopsis thaliana.. J Exp Bot 2012; 63:5903 - 18; http://dx.doi.org/10.1093/jxb/ers239; PMID: 22962679
- Kobayashi T, Nagasaka S, Senoura T, Itai RN, Nakanishi H, Nishizawa NK. Iron-binding Haemerythrin RING ubiquitin ligases regulate plant iron responses and accumulation. Nat Commun 2013; 4:2792; http://dx.doi.org/10.1038/ncomms3792; PMID: 24253678