Abstract
Reactive oxygen species (ROS) are involved in various cellular processes in plants. Among those, resistance to abiotic stress, defence mechanisms and cell expansion have been intensively studied during the last years. We recently demonstrated that ROS, in concert with auxin, have a role in cell cycle activation of differentiated leaf cells.1 In this addendum we provide further evidence to show that oxidative stress/ROS accelerate auxin-mediated cell cycle entry (G0-to-G1
Addendum to: Pasternak TP, Ötvös K, Domoki M, Fehér A. Linked activation of cell division and oxidative stress defense in alfalfa leaf protoplast-derived cells is dependent on exogenous auxin. Plant Growth Regul 2007; 51:109-17.
Cells of a developing multicellular organism have to exit from the cell division cycle before they can differentiate to fulfil a specialized function. However, cell cycle re-entry of differentiated cells may also occur in plants in many instances during normal development (e.g., during organ formation) or in response to environmental impacts (e.g., in response to wounding or pathogen attack). Moreover, somatic plant cells can readily be induced to re-enter the division cycle under in vitro conditions. While our knowledge considering the regulation of cell cycle progression in plants is continuously increasing, we know only little about the key molecular and cellular events governing cell cycle entry (G0-to-G1 transition) that precedes the activation of the core cell cycle machinery with cyclin-dependent kinase, CDK, complexes as central players (reviewed in refs. Citation2 and Citation3). Dissection of G0 and G1 cell cycle phases is difficult in plants but using leaf protoplasts it could be clearly shown that dedifferentiation and cell cycle activation are two separate processes marked by characteristic changes in the chromatin.Citation4,Citation5
Recently it was hypothesized that in animal cells a redox dependent signalling pathway controls the induction of cell division (G0-to-G1 transition) through the regulation of cyclinD1 expression.Citation6 This hypothesis is in concert with numerous observations supporting the view that reactive oxygen species (ROS) may serve as signalling molecules at the crossroad of proliferation, differentiation and cell death (reviewed in ref. Citation7). On the other hand, ROS may also act as toxic metabolic by-products that inhibit cell division. This dual role of ROS is mainly dependent on concentration, pulse duration and site of action. The regulatory role of the cellular redox state acting at transcriptional and post-translational levels during cell cycle progression of animal cells is well demonstrated (reviewed in ref. Citation8). The negative effect of environmental stress and the cellular redox status on plant cell division and especially on the cell cycle machinery including core components such as cyclins and cyclin-dependent kinases (CDKs) is also well documented,Citation9–Citation15 but there are only few reports on the opposite.Citation1,Citation16,Citation17 There are accumulating experimental indications, however, on the important roles of reactive nitrogen species (RNS) during the re-entry of quiescent plant cells into the cell division cycle both in vivo (in the root meristemCitation18) and in vitro (in cell culturesCitation19).
Here we provide some additional evidence that moderate oxidative stress/ROS may also accelerate cell division entry and affect the activity of the cell cycle machinery in cultured plant cells.
Moderate Oxidative Stress, Synergistically with Auxin, Accelerates and is Required for, G0-to-G1 Transition in Cultured Plant Cells
It is not so easy to define the G0 or “quiescent” state in plants where cell differentiation is much more flexible as compared to animals. For example, the cell division kinase (CDK) protein, one of the main enzymes of the cell cycle regulation machinery, is present at a relatively high level even in non-dividing differentiated plant cells, such as of young leaves, marking a specific “cell division competent” stage.Citation20 Despite of this fact, the terminology of “quiescence” or “G0” are used in plant biology as well, and they are very useful to mark plant cells actually being out of the cell cycle with differentiated functions.
Alfalfa leaf cells and freshly prepared protoplasts constitute a reliably homogenous population of mesophyll cells, most of which can be considered to be at the G0-phase of the cell cycle. Up to the moment of protoplast isolation, these cells are specialized for photosynthesis, with RUBISCO (ribulose-1,5-bisphosphate carboxylase/oxygenase) comprising more than 70% of their protein content. As a consequence, majority of nuclear genes are switched off in these cells. Therefore, mesophyll cells and their protoplasts have compact nuclei with a diameter of 3.8–4.4 µm, containing a small nucleolus (diameter around 1 µm), and are characterized by highly condensed chromatin. In the presence of appropriate growth regulators (auxin and cytokinin), these cells start to divide; that is preceded by the remodelling of the chromatin and the de novo synthesis of ribosomes, in order to allow the reorganization of the gene expression pattern and protein synthesis, respectively. These changes are reflected in the morphology and size of the nucleus and the nucleolus and in the amount of DNA/RNA-specific fluorescent dyes they can accumulate ().Citation21 Therefore, these parameters can be well used as cellular markers of G0-to-G1 transition in plantCitation21 as well as in animal cells.Citation22
On it is shown that cell activation (increased size and fluorescence of PI-stained nuclei/nucleoli) is inhibited by the application of diphenyleneiodonium (DPI), an inhibitor of plasma membrane NADPH-oxidase, one of the potential source enzymes of ROS (superoxide and hydrogen peroxide). PM NADPH oxidase has been proposed to play a significant role in the proliferation of animal cells as well (reviewed in refs. Citation23–Citation25). In contrast, at a limiting level of exogenous auxin, moderate oxidative stress (provoked e.g., by 50 µM CuSO4) promoted G0-to-G1 transition as indicated by the increased size and RNA-dependent fluorescence of nuclei/nucleoli (). Although oxidative stress alone (e.g., copper-treatment, ) is also capable to increase RNA-dependent nuclear fluorescence, it may rather be associated with cell death-related changes in transcription/chromatin organization as indicated by uneven nucleoplasm staining, and small and un-stained (no increased rRNA synthesis) nucleoli (). Oxidative stress alone (in the absence of auxin) is not capable to enhance cell cycle entry in this type of cells.Citation1
Similar treatments, low level of auxin combined with oxidative stress, have previously been shown to accelerate the entry of the cells into the S-phase of the cell cycle.Citation17,Citation19 Based on the above data, we hypothesize, however, that this acceleration is due to a shortened G0-to-G1 transition rather than to an accelerated G1 phase.
The complex interaction of auxin and reactive oxygen/nitrogen species during plant development is not well known yet but more and more data accumulate indicating a mutual interaction that may be based on auxin transport inhibition and auxin-mediated ROS generation/removal what finally may converge on chromatin remodelling.Citation16,Citation26
ROS may Enhance the Activation of a Plant CDK Complex During Cell Cycle Entry
In animal cells it is already well accepted that low level of ROS promotes cell proliferation in various cell types (reviewed in refs Citation8 and 28). It has also been proposed that G0-to-G1 transition, the only cell cycle phase transition not governed by cyclin-dependent kinases, rests on redox-dependent signal transduction pathways that finally converge on cyclinD1 expressionCitation6. D-type cyclins are also important regulators of G0/G1-to-S cell cycle phase transition in plants, and they are also hypothesized to mediate external signals towards the cell cycle machinery (reviewed in ref. Citation2). Although the redox-dependent expression of D-type cyclin genes has not been established yet in plants, the activation of CycD3;1 transcription by nitric oxide during cell cycle activation has already been described in different experimental systems.Citation18,Citation19 In the same studies, the enhanced transcription/activity of the cyclin-dependent CDKA1 protein kinase has also been demonstrated.Citation18,Citation19
Here we provide evidence that oxidative stress/ROS might also play a role in CDKA1 activation during the re-entry of cultured plant cells into the cell division cycle. Alfalfa stationary phase (11 days without subculture) suspension cultured cells were transferred to fresh media and the cell cycle re-entry was monitored in the absence and presence of 30 µM CuSO4, as an inducer of mild oxidative stress, and 1 µM DPI as NADPH oxidase inhibitor. The treatments were applied directly at subculture. On it can be observed that the stress treatment transiently enhanced the CDK activity at 24–48 hours after subculture that was prevented by parallel DPI application. DPI alone resulted only in a slightly decreased CDK activity but still inhibited cell division as indicated by the decreased growth rate of the cultures ().
Conclusions
The redox control of cell division is well established in animal cells but has hardly been investigated in plants. It is plausible to hypothesize that similarly as in animal cells reactive oxygen and nitrogen species have dual role in regulating cell division; depending on their concentration, pulse duration and site of action they may promote or inhibit cell proliferation (). Cell cycle entry (G0-to-G1) transition may represent a regulatory node where the cellular redox status has a central role. Whether ROS/RNS-mediated CDK activation is exerted through the activation of Cyclin D expression in plants, similarly as in animals, is still a question to be answered.
Figures and Tables
Figure 1 Oxidative stress/ROS enhance and are required for G0-to-G1 transition in leaf protoplast-derived alfalfa cells as indicated by nuclear parameters. (A) Diphenylene iodonium (DPI; 1 µM) applied to leaf protoplasts prevents cell activation in the presence of auxin (1 µM 2,4-dichlorophenoxyacetic acid, 2,4-D) and cytokinin (1 µM zeatin) as indicated by flow cytometric analysis (Part a) and microscopic investigation (Part b) of PI-stained (4 µg/ml; staining DNA as well as RNA) nuclei at four days of culture. (Part a) The histograms show the numbers of nuclei falling into fluorescence intensity groups (as defined by detection “channels”) depending on the amount and accessibility of nuclear DNA and RNA for staining. The fluorescence channels representing nuclei in G0 and G1 phases are indicated. (Part b) The sizes of nuclei and nucleoli in DPI-treated and control cells, as above. Increased sizes indicate active transcription and rRNA synthesis. DPI-treatment kept the nuclei as they could be observed in leaf cells (data not shown). DPI-DPI + auxin, A-auxin. (B) Copper stress (50 µM CuSO4) enhanced cell activation in the presence of 1 µM 2,4-D (and 1 µM zeatin). Alfalfa leaf protoplasts were cultured without auxin and copper (P), with copper stress (Cu), or copper stress and auxin (A + Cu). Nuclei were subjected to flow cytometric analysis following propidium iodide (PI, DNA and RNA) staining without (Part a) or after RNase-treatment (Part b) as above. RNase treatment diminished the difference of nuclear fluorescence among the control and treated cells indicating the role of nuclear RNA accumulation in the fluorescence intensity differences. Microscopic observations (Part c) of stained (DAPI staining DNA, left; PI staining DNA and RNA, right) highlighted the differences among cells treated by copper in the presence or absence of auxin, especially in the size and RNA content (arrow) of the nucleoli and homogeneity of nucleoplasm staining.
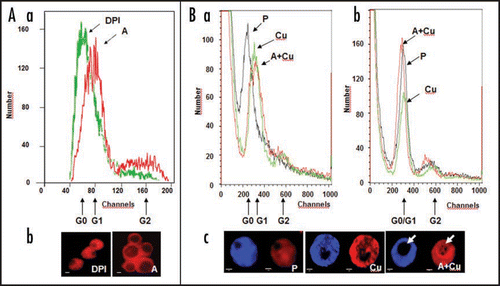
Figure 2 Effect of copper stress and DPI application on the cell cycle re-entry of stationary phase cultured plant cells. (A) The amount and activity of the cell cycle-regulatory CDKA/BMs kinase in 11-day old suspension-cultured alfalfa cells following subculture into fresh medium supplemented with copper (30 µM CuSO4, Cu), DPI (1 µM, DPI), their combination (Cu + DPI), or nothing (Contr.). The upper histogram shows the densitometry values of the CDK activity (as in vitro histone H1 phosphorylation activity of immunoprecipitated CDKA/BMs protein complexes also shown in the upper lane as an autoradiogram). The CDKA/BMs protein amount is determined by Western analysis (lower lane). (B) Growth of the control and treated cells as shown by fresh weight four days after subculture. The initial cell density is shown as a dashed line.
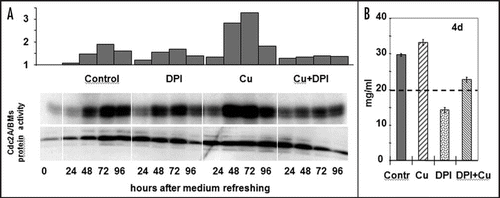
Figure 3 The scheme of potential interactions determining cellular response to oxidative stress. ROS/RNS are formed in the cells as the part of normal metabolism as well as in response to environmental stresses. ROS/RNS themselves as well as growth regulators regulate scavenging pathways to keep ROS/RNS levels low (for simplicity, only auxin is shown as beeing the main growth regulator implicated in cell cycle activation). In case net ROS/RNS production exceeds a limit due to increased production or decreased removal, differentiated cells will die. In contrast, a transient and moderate increase in ROS/RNS level may enhance cell activation in concert with auxin action.
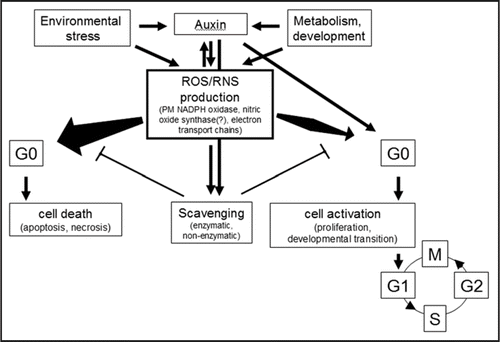
Addendum to:
References
- Pasternak TP, Ötvös K, Domoki M, Fehér A. Linked activation of cell division and oxidative stress defense in alfalfa leaf protoplast-derived cells is dependent on exogenous auxin. Plant Growth Regul 2007; 51:109 - 117
- Francis D. The plant cell cycleó15 years on. New Phytologist 2007; 174:261 - 278
- De Veylder L, Beeckman T, Inze D. The ins and outs of the plant cell cycle. Nat Rev Mol Cell Biol 2007; 8:655 - 665
- Grafi G, Avivi Y. Stem cells: a lesson from dedifferentiation. Trends Biotech 2004; 22:388 - 389
- Zhao J, Morozova N, Williams L, Libs L, Avivi Y, Grafi G. Two phases of chromatin decondensation during dedifferentiation of plant cells. Distinction between competence for cell fate switch and a commitment for S phase. J Biol Chem 2001; 276:22772 - 22778
- Burch PM, Heintz NH. Redox regulation of cell cycle re-entry: cyclin D1 as a primary target for the mitogenic effects of reactive oxygen and nitrogen species. Antioxid Redox Sign 2005; 7:741 - 751
- Sauer H, Wartenberg M, Hescheler J. Reactive oxygen species as intracellular messengers during cell growth and differentiation. Cell Physiol Biochem 2001; 11:173 - 186
- Menon SG, Goswami PC. A redox cycle within the cell cycle: ring in the old with the new. Oncogene 2006; 26:1101 - 1109
- Schuppler U, He P, John PC , Munns R. Effect of water stress on cell division and cdc2-like cell cycle kinase activity in wheat leaves. Plant Physiol 1998; 117:667 - 678
- Reichheld J, Vernoux T, Lardon F, Van Montagu M, Inze D. Specific checkpoints regulate plant cell cycle progression in response to oxidative stress. Plant J 1999; 17:647 - 656
- Potters G, Horemans N, Caubergs RJ, Asard H. Ascorbate and dehydroascorbate influence cell cycle progression in a tobacco cell suspension. Plant Physiol 2000; 124:17 - 20
- Potters G, Horemans N, Bellone S, Caubergs RJ, Trost P, Guisez Y, Asard H. Dehydroascorbate influences the plant cell cycle through a glutathione-independent reduction mechanism. Plant Physiol 2004; 134:1479 - 1487
- Potters G, De Gara L, Asard H, Horemans N. Ascorbate and glutathione: guardians of the cell cycle, partners in crime?. Plant Physiol Biochem 2002; 40:537 - 548
- Granier C, Inze D, Tardieu F. Spatial distribution of cell division rate can be deduced from that of p34cdc2 kinase activity in maize leaves grown at contrasting temperatures and soil water conditions. Plant Physiol 2000; 124:1393 - 1402
- Burssens S, Himanen K, van de Cotte B, Beeckman T, Van Montagu M, Inze D, Verbruggen N. Expression of cell cycle regulatory genes and morphological alterations in response to salt stress in Arabidopsis thaliana. Planta 2000; 211:632 - 640
- Pasternak T, Potters G, Caubergs R, Jansen MAK. Complementary interactions between oxidative stress and auxins control plant growth responses at plant, organ, and cellular level. J Exp Bot 2005; 56:1991 - 2001
- Pasternak TP, Prinsen E, Ayaydin F, Miskolczi P, Potters G, Asard H, Van Onckelen HA, Dudits D, Fehér A. The role of auxin, Ph, and stress in the activation of embryogenic cell division in leaf protoplast-derived cells of alfalfa. Plant Physiol 2002; 129:1807 - 1819
- Correa Aragunde N, Graziano M, Chevalier C, Lamattina L. Nitric oxide modulates the expression of cell cycle regulatory genes during lateral root formation in tomato. J Exp Bot 2006; 57:581 - 588
- Ötvös K, Pasternak TP, Miskolczi P, Domoki M, Dorjgotov D, Szúcs A, Bottka S, Dudits D, Fehér A. Nitric oxide is required for, and promotes auxin-mediated activation of, cell division and embryogenic cell formation but does not influence cell cycle progression in alfalfa cell cultures. Plant J 2005; 43:849 - 860
- Hemerly AS, Ferreira P, de Almeida Engler J, Van Montagu M, Engler G, Inze D. Cdc2a expression in arabidopsis is linked with competence for cell division. Plant Cell 1993; 5:1711 - 1723
- Pasternak T, Miskolczi P, Ayaydin F, Mészáros T, Dudits D, Fehér A. Exogenous auxin and cytokinin dependent activation of cdks and cell division in leaf protoplast-derived cells of alfalfa. Plant Growth Regul 2000; 32:129 - 141
- Bottiroli G, Croce AC, Bottone MG, Vaccino S, Pellicciari C. G0-G1 cell cycle phase transition as revealed by fluorescence resonance energy transfer: Analysis of human fibroblast chromatin. Eur J Histochem 2004; 48:37 - 48
- Ammendola R, Ruocchio MR, Chirico G, Russo L, De Felice C, Esposito F, Russo T, Cimino F. Inhibition of NADPH oxidase affects signal transduction by growth factor receptors in normal fibroblasts. Archives Biochem Biophys 2002; 397:253 - 257
- Mofarrahi M, Brandes RP, Gorlach A, Hanze J, Terada LS, Quinn MT, Mayaki D, Petrof B, Hussain SNA. Regulation of proliferation of skeletal muscle precursor cells by NADPH oxidase. Antioxid Redox Sign 2008; 10:559 - 574
- Griendling KK. NADPH oxidases: new regulators of old functions. Antioxid Redox Sign 2006; 8:1443 - 1445
- Beveridge CA, Mathesius U, Rose RJ, Gresshoff PM. Common regulatory themes in meristem development and whole-plant homeostasis. Curr Opin Plant Biol 2007; 10:44 - 51
- Rothstein EC, Lucchesi PA. Redox control of the cell cycle: a radical encounter. Antioxid Redox Sign 2005; 7:701 - 703