Abstract
In our recent paper in Plant Physiology, we have reported the identification and functional characterization of a unique regulator, SHW1, a serine-arginine-aspartate rich protein in Arabidopsis seedling development.1 Genetic and molecular analyses have revealed that SHW1 functions in an independent and interdependent manner with COP1, and differentially regulates photomorphogenic growth and light regulated gene expression. Here, we show the involvement of photoreceptors in the function of SHW1. Our results have further revealed that SHW1 is a common regulator of light and ABA signaling pathways. These results along with some data described in Plant Physiology paper have been discussed here in a broader perspective.
Addendum to: Bhatia S, Gangappa SN, Kushwaha R, Kundu S, Chattopadhyay S. Short hypocotyl in white light (SHW1), a serine-arginine-aspartate rich protein in Arabidopsis, acts as a negative regulator of photomorphogenic growth. Plant Physiol, In Press.
Plants are exposed to various intensities and wavelengths of light with a specific wavelength of light being predominant at a particular daytime. For example, plants are exposed to varied intensities of light in the morning and noon, or far-red light being predominant in the twilight. However, plants have also evolved to respond to and subsequently tackle such variations in light quality or quantity by multiple modes of actions. One such mode of action might be to employ multiple negative regulatory proteins that function as filtering units to light intensity. These negative regulators could be operative in a specific wavelength of light or in a broad spectrum of lightCitation2. Identification and functional characterization of several negative regulators, including SHW1, of photomorphogenic growth support such notion. SHW1 does not seem to have a homologue in animal system or in lower eukaryotes, and thereby has evolved as a plant specific gene. When seedlings are exposed to light after reaching the soil surface, it is important to protect the emerging cotyledons from high intensity light that otherwise might get bleached and subsequently die. SHW1 is expressed in germinating seeds to flowering plants, and it is predominantly expressed in the photosynthetically active tissues.Citation1 Therefore, SHW1 might function as a filtering unit not only in the case of emerging seedlings in the soil but also in the adult plants during dark to light transition.
Involvement of Photoreceptors in the Function of SHW1
The most striking feature of shw1 mutants, which are partly photomorphogenic in the dark, is that the light mediated enhanced inhibition of hypocoytyl elongation is restricted to WL without any visible effect in RL, FR or BL.Citation1 To examine the possible involvement of photoreceptorsCitation2 in the function of SHW1, we generated double mutants such as shw1 phyA, shw1 phyB and shw1 cry1 and carried out epistasis analyses. The shw1 phyB double mutants displayed significantly reduced hypocotyl length as compared to phyB single mutants in WL, suggesting that shw1 could partly suppress the phyB mutant phenotype in WL ( and B). On the other hand, shw1 phyA and shw1 cry1 double mutants showed similar hypocotyl lengths as phyA and cry1 single mutants, respectively ( and D). These results suggest that the increased sensitivity to WL caused by shw1 mutation requires light perception by phyA and cry1. Taken together, the epistatic analyses indicate that SHW1 mediated inhibition of hypocotyl elongation in WL may play an important role in negative feed back control of phyB signaling, whereas the functions of phyA and cry1 are likely to be independent of SHW1.
shw1 Mutants are Less Sensitive to ABA Responsiveness
The cross talk between light and various other signaling cascades have been reported.Citation3–Citation6 Phytochrome signaling controls the expression of TOP2, one of the components of DNA replication and cell cycle machinery.Citation7 HY5 and HYH transcription factors of light signaling have been shown to act as a point of cross-talk among light, auxin and cytokinin signaling pathways.Citation8,Citation9 MYC2/ZBF1 transcription factor has been shown to act as a point of cross talk among light, abscisic acid (ABA), jasmonic acid (JA) and ethylene-jasmonate signaling pathways.Citation10–Citation14
To determine whether shw1 mutants have altered ABA responses, we monitored the effect of ABA on shw1 mutant plants. Seeds of wild type and mutant plants were plated on MS plates in the absence or presence of various concentrations of ABA. As shown in and B, whereas 1mM ABA severely reduced the rate of germination of wild type seeds, the effect was significantly suppressed in shw1 mutants either in constant dark or WL condition. Quantification of these results revealed that after 6 days, whereas the rate of seed germination was found to be about 2 percent in wild type, about 20 percent seeds of shw1 mutants were germinated in the dark. This dramatic elevated level of seed germination in shw1 mutants was maintained after twelve days of incubation in the darkness (). Similarly, significantly increased rate of seed germination was observed in shw1 mutants compared to wild type in WL (). Collectively, these results suggest that shw1 mutants are less sensitive to ABA mediated inhibition of seed germination.
The light and ABA effects are potentially antagonistic. For example, the loss of function mutants of MYC2/ZBF1 display enhanced inhibition of hypocotyl elongation in BL, and less sensitive to ABA-mediated inhibition of seed germination.Citation14 Examination of ABA responsiveness of shw1 mutants in this study reveals that shw1 mutants are less sensitive to ABA-mediated inhibition of seed germination either in dark or WL conditions. Thus, SHW1, the negative regulator of photomorphogenic growth, acts as a positive regulator of ABA mediated inhibition of seed germination. Although the exact mechanism of differential regulation of light and ABA signaling pathways by SHW1 is not known, demonstration of SHW1 as a common regulator for light and ABA will help in deciphering the mechanism of integration of these signaling pathways in future. A simple way to explain the differential regulation is to consider that SHW1 could function either as an activator or repressor, depending on the available light conditions and ABA levels in the seedlings. Alternatively, it could be envisioned that SHW1 modulates independent regulatory proteins of light and ABA signaling pathways which in turn play opposite regulatory roles in signaling cascades.
Figures and Tables
Figure 1 SHW1 and phyB Function antagonistically to Regulate Hypocotyl Length in WL. (A) Visible phenotype of 6-day-old constant WL (15 µmol m−2 s−1) grown segregated wild type, shw1-1, phyB and shw1 phyB seedlings shown from left to right, respectively. (B–D) Hypocotyl lengths of 6-day-old constant WL (15 µmol m−2 s−1) grown segregated wild type (WT) and various mutant seedlings.
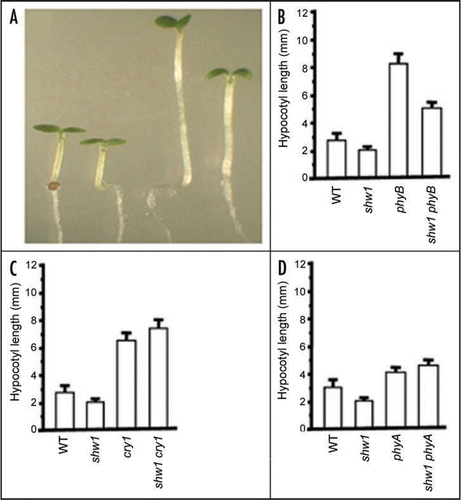
Figure 2 The shw1 Mutants are Less Responsive to ABA. (A) Six-day-old constant dark grown seedlings in the presence of 1 µM ABA. (B) Six-day-old constant WL (30 µmol m−2 s−1) grown seedlings in the presence of 1 µM ABA. (C) Quantification of rate of seed germination in dark grown wild type (Col) and shw1-1 mutant seedlings in the presence of 1 µM ABA at various days. (D) Quantification of rate of seed germination in constant WL (30 µmol m−2 s−1) grown wild type (Col) and shw1-1 mutant seedlings in the presence of 1 µM ABA at various days.
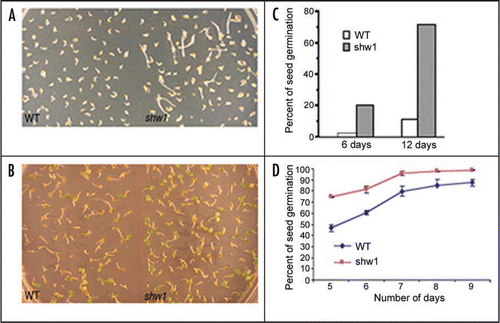
Addendum to:
References
- Bhatia S, Gangappa SN, Kushwaha R, Kundu S, Chattopadhyay S. Short hypocotyl in white light (SHW1), a serine-arginine-aspartate rich protein in Arabidopsis, acts as a negative regulator of photomorphogenic growth. Plant Physiol 2008; 147:169 - 178
- Jiao Y, Lau OS, Deng XW. Light-regulated transcriptional networks in higher plants. Nat Rev Genet 2007; 8:217 - 230
- Chory J, Reinecke D, Sim S, Washburn T, Brenner M. A role for cytokinins in de-etiolation in Arabidopsis (det mutants have an altered response to cytokinins). Plant Physiol 1994; 104:339 - 347
- Weatherwax SC, Ong MS, Degenhardt J, Bray EA, Tobin EM. The interaction of light and abscisic acid in the regulation of plant gene expression. Plant Physiol 1996; 111:363 - 370
- Genoud T, Buchala AJ, Chua NH, Metraux JP. Phytochrome signalling modulates the SA perceptive pathway in Arabidopsis. Plant J 2002; 31:87 - 95
- Moon J, Zhao Y, Dai X, Zhang W, Gray WM, Huq E, Estelle M. A new CULLIN 1 mutant has altered responses to hormones and light in Arabidopsis. Plant Physiol 2007; 143:684 - 696
- Hettiarachchi GHCM, Yadav V, Reddy MK, Chattopadhyay S, Sopory SK. Light mediated regulation defines a minimal promoter region of TOP2. Nucl Acids Res 2003; 31:5256 - 5265
- Sibout R, Sukumar P, Hettiarachchi C, Holm M, Hardtke CS. Opposite root growth phenotypes of hy5 versus hy5 hyH mutants correlate with increased constitutive auxin signaling. PLOS Genet 2006; 2:202
- Vandenbussche F, Habricot Y, Condiff AS, Maldiney R, Straeten DVD, Ahmad M. HY5 is a point of convergence between cryptochrome and cytokinin signaling pathways in Arabidopsis thaliana. Plant J 2007; 49:428 - 441
- Abe H, Urao T, Ito T, Seki M, Shinozaki K, Yamaguchi-Shinozaki K. Arabidopsis AtMYC2 (bHLH) and AtMYB2 (MYB) function as transcriptional activators in abscisic acid signaling. Plant Cell 2003; 15:63 - 78
- Anderson JP, Badruzsaufari E, Schenk PM, Manners JM, Desmond OJ, Ehlert C, Maclean DJ, Ebert PR, Kazan K. Antagonistic interaction between abscisic acid and jasmonate-ethylene signaling pathways modulates defense gene expression and disease resistance in Arabidopsis. Plant Cell 2004; 16:3460 - 3479
- Boter M, Ruiz Rivero O, Abdeen A, Prat S. Conserved MYC transcription factors play a key role in jasmonate signaling both in tomato and Arabidopsis. Genes Dev 2004; 18:1577 - 1591
- Lorenzo O, Chico JM, Sanchez Serrano JJ, and Solano R. JASMONATE INSENSITIVE1 encodes a MYC transcription factor essential to discriminate between different jasmonateregulated defense responses in Arabidopsis. Plant Cell 2004; 16:1938 - 1950
- Yadav V, Mallappa C, Gangappa SN, Bhatia S, Chattopadhyay S. A basic Helix-loop-helix transcription factor in Arabidopsis, MYC2, acts as a repressor of blue light-mediated photomorphogenic growth. Plant Cell 2005; 17:1953 - 1966