Abstract
Phloem injury triggers local sieve-plate occlusion including callose-mediated constriction and protein plugging of sieve pores. In intact plants, reversible sieve-plate occlusion is induced by electric potential waves (EPWs)—accompanied by Ca2+-influx—as result of distant burning. Here, we present additional results which pertain to (a) the variability of EPW-profiles in relation to forisome conformation in intact Vicia faba plants and (b) the differential occlusion reactions to burning and cutting in various plant species. A correlation between stimulus perception and mode of phloem loading is discussed.
Addendum to: Furch ACU, Hafke JB, Schulz A, van Bel AJE. Ca2+-mediated remote control of reversible sieve-tube occlusion in Vicia faba. J Exp Bot 2007; 58:2827-38.
Modes of Sieve-Plate Occlusion
Upon phloem injury, sieve elements (SEs) are occluded by combined callose-collar formation around sieve poresCitation1,Citation2 and protein pluggingCitation3–Citation6 to prevent leakage of nutrients and invasion of phytopathogens.Citation7
Given the fact that sieve pores are modified plasmodesmata (PDs),Citation8 callose synthesis around PDs and sieve pores may strongly resemble. PD constriction is reached by Ca2+-dependentCitation9 deposition of β-1,3-glucane polymers (callose) into the apoplast, caused by activation of membrane-associated callose synthase (β-1,3-glucan synthase); PD re-opening is achieved by a callose-degrading membrane-associated β-1,3-glucanase.Citation10 In complement sieve-tubes are occluded by structuralCitation7,Citation4 or, possibly, water-soluble proteinsCitation11 (). Among the structural proteins, forisomes (dispersive protein bodies, exclusively present in legume sieve tubes) were of particular importance for our studies. Forisomes disperse upon Ca2+-influx into SE-lumina and recondense spontaneously, probably after Ca2+-removal by Ca2+-pumps.Citation12,Citation13
Remote Control of Sieve-Tube Occlusion
In intact Vicia faba plants, heat stimuli induced distant SE-occlusionCitation13 following the passage of an electrical potential wave (EPWs)Citation14 during which Ca2+ flows into the SEs via activated Ca2+-channels.Citation15 EPWs are generated by mechanical and physical stimuliCitation16–Citation18 and depend on voltage-activated and possibly mechanosensitive channels along sieve tubes.Citation14,Citation19
Our recent studyCitation13 showed, that forisomes at 3–4 cm from the stimulus site dispersed 15–45 sec after burning the leaf tip. The dispersion is reversible; the forisome recondensed after 7–15 min.
In the mean time, calloseCitation1 was deposited around sieve pores and pore plasmodesma units (PPUs; one-sided branched PDs between SE and CC). Callose deposition reached a maximum ca 20 min after the heat shock and was reversible too.Citation13 Callose breakdown at sieve pores lasted for 1.5–2.0 h, whereas degradation appeared to be more rapid at PPUs (30–40 min) (). Burning experiments with Solanum lycopersicum and Ocimum basilicum showed similar, but species-specific time lapses ().
Different EPW-Profiles Correlated with the Forisome Reaction in Response to Leaf Tip Burning
The time lapse between forisome dispersion and recondensation appreciably varies (2–30 min) in Vicia faba, which suggests a variable engagement of Ca2+-channels/pumps. Optical examination in combination with electrophysiology disclosed a relationship between EPW-profile and forisome reaction ( and ). In a typical example of a long depolarisation wave (), an abrupt SE membrane depolarisation from −150 mV down to −40 mV nearly coincides with forisome dispersion, 5–8 seconds after burning. The quick repolarisation changes smoothly into a plateau phase that lasts for several minutes. The forisome remained permanently dispersed during the depolarisation plateau phase (). This may result from massive initial Ca2+-influx before or permanent Ca2+-influx during the plateau. Hence, activity of Ca2+-ATPasesCitation20 may present a physiological bottleneck for removal of Ca2+ from SEs. With a short depolarisation (), the forisome only occasionally dispersed; only a marginal swelling was observed, which suggests a minor Ca2+-influx into the SE.
Wounding Reactions and Passage of EPWs After Cutting the Main Vein of Different Plant Species
Surprisingly, distant cutting of leaf tips of intact Vicia faba and Solanum lycopersicum plants induced neither an EPW nor distant callose deposition, but yet forisome dispersion (Vicia faba). Callose deposition was restricted to a distance of about 0.5 cm from the site of cutting and results from local wound effects. In the vicinity of cutting loss of turgor pressure may cause activation of mechano-sensitive Ca2+-channelsCitation21, but reduced Ca2+-influx into the SE lumen is not sufficient to induce callose production further away from the cut. In conclusion, forisome dispersion may have a lower Ca2+ threshold than callose synthesis.Citation13
EPWs (as in ) were recorded only at distance of 0.1–0.5 cm from the site of cutting; the electrical signal steeply decreased with the distance (). While a depolarisation of 54 mV was measured close to the cut, a spike with amplitude of 6 mV was observed at 2 cm from the cut. From a distance of 3 cm from the site of cutting on, no electrical signal was recorded ().
In contrast to lacking callose deposition in Vicia faba and Solanum lycopersicum, heavy callose deposition was induced by cutting in Ocimum basilicum and Ficus pumila ().
Concluding Remarks
EPW-profiles possibly correspond with the amount of free Ca2+-released into the SE lumen. The variability may depend on the strength of the stimulus and/or the spatial coupling between the SE measured and the site of burning. Strong innate differences between SEs are less likely.
EPWs triggered by cutting are more local and less persistent than those induced by burning. In the latter case, EPWs are presumably based on the collective activities of voltage- and mechano-sensitive Ca2+-channels; only mechano-sensitive channels would be activated by the turgor release following cutting.
The higher sensitivity (callose production) to cutting of symplasmic loaders may be purely coincidental in view of the few species probed. However, it may belong to a conglomerate of structural/functional properties related to the mode of phloem loading. The initial speculationCitation22 that the differential anatomy of SE/CCs in collection phloem of symplasmic and apoplasmic loaders extends into the transport phloem zone, was substantiated by electron microscopic studies.Citation23 Inherent consequences for photoassimilate distribution are inferred from membrane-potential measurements in transport phloem, where the capability of photoassimilate retrieval by SEs is higher in apoplasmic loaders.Citation24 Furthermore, differences between symplasmic and apoplasmic loaders have been reported for stomatal regulation,Citation25 invertase activity in the leaf apoplast,Citation26 and adaptability to high-light regimes.Citation27
Abbreviations
CC | = | companion cell |
EPW | = | electrical potential wave |
PD | = | plasmodesma |
PPU | = | pore plasmodesma unit |
SE | = | sieve element |
SE/CC | = | sieve element/companion cell complex |
Figures and Tables
Figure 1 Survey of the diversity of phloem-specific proteins in SEs. (1) Parietal protein clots, (1a) Fibrillar protein nets, (2) Dispersive protein bodies (=forisomes), (3) Non-dispersive protein bodies, (4) Proteins in SE plastids, (5) Soluble proteins.
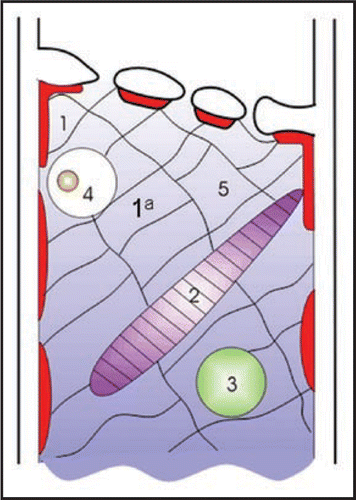
Figure 2 Long-lasting electrical response of a Vicia faba SE following burning the leaf tip (at a distance of 3 cm) and photographs taken simultaneously as an optical control of forisome reaction (arrows 1–4). The forisome dispersed during the depolarisation and recondensed after repolarisation (3↑–4↑, 13.5 minutes). The forisome is marked with an asterisk. m, microcapillary for membrane potential recording.
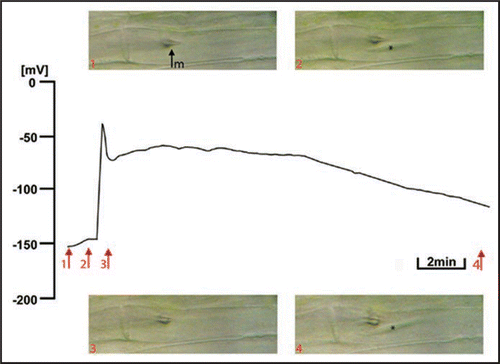
Figure 3 Short-lasting electrical response of a Vicia faba SE following burning the leaf tip (at a distance of 3 cm) and photographs taken simultaneously as an optical control of forisome reaction (arrows 1–4). The forisome remained in the condensed state during the short plateau phase (2↑-3↑, 3 min). The forisome is marked with an asterisk. m, microcapillary for membrane potential recording.
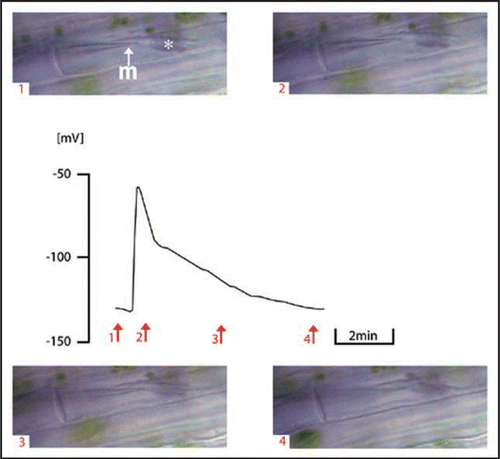
Figure 4 Signal conduction in the form of EPW in Vicia faba SEs in dependence of the distance from the cutting site after subsequent cutting (2) with an earthed razor blade. The schematic drawing on the right side shows the experimental design. 1↑-2 cm from the cutting site; 2↑-1 cm from the cutting site; m, microcapillary for membrane potential recording; rb, razor blade.
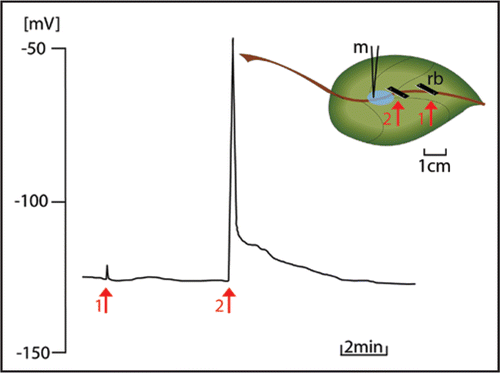
Table 1 Time-course of sieve-tube occlusion
Table 2 Callose deposition and mode of phloem loading
Acknowledgements
This work was supported by the Deutsche Forschungsgemeinschaft (BE 1925/8-2) in the frame of Schwerpunktprogramm 1108.
Addendum to:
References
- King RW, Zeevaart JAD. Enhancement of phloem exudation from cut petioles by chelating agents. Plant Physiology 1974; 53:96 - 103
- Zabotin AI, Barysheva TS, Trofimova OI, Lozovaya VV, Widholm J. Regulation of callose metabolism in higher plant cells in vitro. Russ J Plant Physiol 2002; 49:792 - 798
- Evert RF. Sieve-tube structure in relation to function. Bioscience 1982; 32:789 - 795
- Alosi MC, Melroy DL, Park RB. The regulation of gelation of phloem exudates from Cucurbita fruit by dilution, glutathione, and glutathione reductase. Plant Physiol 1988; 86:1089 - 1094
- Knoblauch M, van Bel AJE. Sieve tubes in action. Plant Cell 1998; 10:35 - 50
- van Bel AJE. Baluska F, Volkmann D, Barlow PW. Cell-Cell Channels 2006; 113 - 118 (www.Eurekah.com)
- van Bel AJE. Transport phloem: Low profile, high impact. Plant Physiol 2003; 131:1509 - 1510
- Evert RF. Behnke HD, Sjolund RD. Dicotyledons. Sieve Elements, Comparative Structure, Induction and Development 1990; Springer New York 103 - 137
- Colombani A, Djerbi S, Bessueille L, Blomqvist K, Ohlsson A, Berglund T, Teeri TT, Bulone V. In vitro synthesis of (1→3)-β-D-glucan (callose) and cellulose by detergent extracts of membranes from cell suspension cultures of hybrid aspen. Cellulose 2004; 11:313 - 327
- Levy A, Erlanger M, Rosenthal M, Epel BL. A plasmodesmata-associated b-1,3-glucanase in Arabidopsis. Plant J 2007; 49:669 - 682
- Will T, van Bel AJE. Physical and chemical interactions between aphids and plants. J Exp Bot 2006; 57:729 - 737
- Knoblauch M, Peters WS, Ehlers K, van Bel AJE. Reversible calcium-regulated stopcocks in legume sieve tubes. Plant Cell 2001; 13:1221 - 1230
- Furch ACU, Hafke JB, Schulz A, van Bel AJE. Ca2+-mediated remote control of reversible sieve tube occlusion in Vicia faba. J Exp Bot 2007; 58:2827 - 2838
- Davies E. New functions for electrical signals in plants. New Phytologist 2004; 161:607 - 610
- Volk GM, Franceschi VR. Localization of a calcium channel-like protein in the sieve element plasma membrane. Austr J Plant Physiol 2000; 27:779 - 786
- Rhodes JD, Thain JF, Wilson DC. The pathway for systemic electrical signal conduction in the wounded tomato plant. Planta 1996; 200:50 - 57
- Stahlberg R, Cosgrove DJ. The propagation of slow wave potentials in pea epicotyls. Plant Physiol 1997; 113:209 - 217
- Fromm J, Lautner S. Electrical signals and their physiological significance in plants. Plant Cell Environm 2007; 30:249 - 257
- Brenner ED, Stahlberg R, Mancuso S, Vivanco J, Baluska F, van Volkenburgh E. Plant neurobiology: an integrated view of plant signalling. Trends Plant Sci 2006; 11:413 - 419
- Hetherington AM, Brownlee C. The generation of Ca2+ signals in plants. Ann Rev Plant Biol 2004; 55:401 - 427
- Hafke JB, Furch ACU, Reitz MU, van Bel AJE. Functional sieve element protoplasts. Plant Physiol 2007; 145:703 - 711
- van Bel AJE. Interaction between sieve element and companion cell and the consequences for photoassimilate distribution. Two structural hardware frames with associated physiological software packages in dicotyledons?. J Exp Bot 1996; 47:1129 - 1140
- Kempers R, Ammerlaan A, van Bel AJE. Symplasmic constriction and ultrastructural features of the sieve element companion cell complex in the transport phloem of apoplasmically and symplasmically phloem-loading species. Plant Physiol 1998; 116:271 - 278
- Hafke JB, van Amerongen JK, Kelling F, Furch ACU, Gaupels F, van Bel AJE. Thermodynamic battle for photosynthate acquisition between sieve tubes and adjoining parenchyma in transport phloem. Plant Physiol 2005; 138:1527 - 1537
- Kang Y, Outlaw WH Jr, Fiore GB, Riddle KA. Guard cell apoplastic photosynthate accumulation corresponds to a phloem-loading mechanism. J Exp Bot 2007; 58:4061 - 4070
- Kingston Smith AH, Pollock CJ. Tissue level localization of acid invertase in leaves: an hypothesis for the regulation of carbon export. New Phytol 1996; 134:423 - 432
- Amiard V, Mueh KE, Demmig Adams B, Ebbert V, Turgeon R, Adams WW III. Anatomical and photosynthetic acclimation to the light environment in species with differing mechanisms of phloem loading. Proc Natl Acad Sci USA 2005; 102:12968 - 12973