Abstract
Phosphoinositides (PIs) control various cellular functions of eukaryotic cells. PIs are derived from phosphatidylinositol (PtdIns) by phosphorylation of the inositol-ring in the lipid-head group; the action of specific lipid kinases gives rise to a family of structurally-related PIs representing PtdIns-mono-, bis-, and -trisphosphates. Specific PIs, such as phosphatidylinositol-4,5-bisphosphate (PtdIns(4,5)P2), can influence more than one physiological process, raising the question as to how interactions with alternative protein partners are coordinated. Previous studies have proposed that PIs are organized by spatiotemporal compartmentation into distinct functional pools, however, mechanisms for the generation and maintenance of such pools has not been presented. Several recent studies now indicate that not only the distinctive inositolpolyphosphate head groups may be relevant for PI function but also the associated fatty acyl-moieties, which may be involved in sorting of lipid precursors into distinct pools. This mini-review aims at highlighting recent evidence that PI acyl-groups exert relevant effects on signaling.
The Plant Phosphoinositide System
A plethora of information is available on the PI systems of mammalian cells and yeast, however, knowledge on PI functions in plants is still rather limited. Extensive research on PIs in eukaryotic organisms indicates that more physiological functions of these enigmatic lipids remain to be discovered even in the better-characterized model systems. By acting as specific lipid ligands to various alternative protein partners PIs can regulate localization or activity of specific target proteins, and ensuing effects on physiology in various eukaryotic organisms have been extensively reviewed.Citation1–Citation4 Depending on the nature of the target, physiological processes controlled, for instance, by PtdIns(4,5)P2 alone include the control of ion-channel and ATPase activity,Citation5,Citation6 the regulation of cytoskeletal dynamicsCitation7–Citation10 and hormonal and stress signalingCitation1–Citation4,Citation11 in different eukaryotic models, including plants. In addition to functions as intact lipids, PIs also serve as substrates for phospholipase C (PLC), which cleaves the lipids to release the respective soluble inositolphosphate head groups and diacylglycerol (DAG).Citation11 It is obvious that the action of multifunctional effectors, such as PIs, must be tightly regulated.
The subcellular distribution of PIs in a eukaryotic cell is a key factor in determining the regulatory effects that will be exerted by the lipids.Citation9 PIs and the activities of their biosynthetic enzymes have been found associated with different subcellular structures of plant cells,Citation4,Citation11,Citation12 suggesting that different regulatory functions of PIs are exerted in different compartments. Previous work performed on plants provides a body of circumstantial and direct evidence that PIs are organized in distinct lipid poolsCitation13–Citation17 that may be independently regulated. So far, however, it is unresolved how independent pools of inositol-containing lipids can be established and maintained.
Different Pools of Phosphoinositides
Research on PIs up to now has mainly focused on the distinction of lipids categorized according to their specific headgroups, and the glycerolipid fatty acyl-backbone has been largely neglected. The development of analytical techniques to resolve both head group identity and fatty acid composition of PIs allowed to detect the lipids in biological materialCitation18 and has aided the investigation of PI pools also in plants. A number of proposals of independent PI pools in plants or algae were based on indirect evidence and reflect attempts at explaining sometimes counterintuitive results from biochemical studies.Citation13,Citation14,Citation19 While the interpretation of previous models was somewhat speculative and subject to debate, direct evidence for the presence of independent PI-pools came recently from the observation that the fatty acid composition of PIs in Arabidopsis leaves transiently increasing with hyperosmotic stress differed from those of PIs that were constitutively present in that tissue. So far, the exact physiological roles of the increased PI levels induced by the stress are not clear, however, it can be assumed that the transiently formed lipids have functions in stress adaptation. By tracing the appearance of unsaturated diacylglycerol backbones in various possible lipid products downstream of PtdIns(4,5)P2-formation, it was proposed that part of the unsaturated PIs are involved in the generation of inositol-1,4,5-trisphosphate (Ins(1,4,5)P3)-signals.Citation15 Other stress-induced PI-species may perform regulatory functions as intact lipids.Citation15 In a subsequent study on wound-induced lipid changes in Arabidopsis,Citation20 increased formation of unsaturated PI-species was also observed, supporting the earlier findings. The differences in associated fatty acids provide a clear indication that constitutive and stress-induced PIs originate from different pools of PtdIns.
Lipid Heads and Tails
When contemplating the possibility that the acyl-moiety of PIs contributes to subcellular sorting of PIs and, thus, the definition of their physiological functions, the biogenesis of the acyl-lipid precursors must be considered. PtdIns, the parent lipid of all PIs, is synthesized in the endoplasmic reticulum (ER) from cytidinediphospho-diacylglycerol (CDP-DAG) and D-myo-inositol by PtdIns-synthase (PI-synthase)Citation21,Citation22 and is distributed by an unknown mechanism to various target locations in the cell. PtdIns can then be sequentially phosphorylated by PtdIns-kinases (PI-kinases) and PtdIns-monophosphate kinases (PIP-kinases), which generate the known PI isomers.Citation4
PI-synthase Isoforms Create PI-precursors with Different Diacylglycerol Backbones
Based on the lipid patterns observed with stress-treatmentsCitation15,Citation20 the presence of at least two pools of PtdIns was postulated that consist of lipid species differing in fatty acid composition. The presence of two pools of PtdIns that were possibly giving rise to PIs with different functions prompted the investigation of the responsible PI-synthase isoforms. The Arabidopsis genome contains two sequences with similarity to PI-synthases. The cDNAs encoding both PIS-isoenzymes (PIS1 and PIS2) were cloned and the recombinant enzymes demonstrated to possess similar catalytic activity in vitro.Citation21–Citation23 Tests with different CDP-DAG species indicated differences in substrate preference with PIS1 and PIS2 preferring saturated or more unsaturated CDP-DAG substrates, respectively.Citation23
Another factor governing the distinction of PtdIns-populations generated by PIS1 or PIS2 is the subcellular localization of the enzymes, which may in part depend on specific substrate binding. Fluorescently labelled PIS1 and PIS2 localized predominantly to the ER, as was previously shown for PI-synthases from other organisms. Occasionally punctate structures were observed, in which both PIS1 and PIS2 were present in clearly distinct patterns.Citation23 The data so far indicated that PIS1 and PIS2 differ in both substrate specificity and subcellular localization.
PtdIns Produced by PIS1 or PIS2 Enters Alternative Routes of Conversion
An important functional insight into the role of PI-synthases in the generation of distinct pools of PtdIns comes from the biochemical effects of overexpression in Arabidopsis. When PIS1 or PIS2 were ectopically expressed in transgenic Arabidopsis plants, the transgenic plant lines were retarded in growth compared to wild type controls grown in parallel. The transgenic lines contained increased amounts of PtdIns, as expected, and had also increased levels of phosphatidic acid, a possible breakdown product of PtdIns. While PtdIns levels were increased in both cases, overexpression of PIS1 or PIS2 resulted in differential effects on other phospholipids. While the formation of PtdIns4P and PtdIns(4,5)P2 was increased in plants overexpressing PIS2, plants overexpressing PIS1 had increased levels of phosphatidylethanolamine (PtdEtn) and no increased content of polyphosphoinositides.Citation23 The data suggest that PtdIns originating from PIS1 activity entered a different route of further conversion than PtdIns formed by PIS2. The fatty acid composition of phospholipid products in plants overexpressing PIS1 indicated an increased proportion of saturated fatty acids, whereas in plants overexpressing PIS2 product lipids contained more unsaturated fatty acids.Citation23 Importantly, these results mirrored the data from the in vitro characterization of PIS1 and PIS2 and indicate physiological relevance for the observed differences in substrate specificities. The data suggest that PIS1 and PIS2 may act in different metabolic contexts, resulting in PtdIns channeling towards different physiological pools. illustrates a hypothetical model how different species of PtdIns may give rise to partially independent PI-pools.
Distribution of PtdIns-species and Further Conversion to Polyphosphoinositides
In principle, PtdIns can be delivered to the sites of further conversion by association with secretory vesicles or by action of PI-specific lipid transfer proteins,Citation24,Citation25 or by a combination of both mechanisms. While the two PI-synthase isoforms, PIS1 and PIS2, may provide two pools of PtdIns, further conversion into different PIs is catalyzed by phosphoinositide-kinases that—with the exception of the single PI 3-kinase—represent enzyme families.Citation11 The Arabidopsis genome encodes at least four different isoforms of PI 4-kinase, eleven of PI4P 5-kinase, and four of PI3P 5-kinase.Citation11 The multitude of isoforms may be an indication for specialized functionality in the context of pool-specific PI-formation according to the cell's physiological requirements. Information on possible routes of PtdIns delivery comes from cell biological studies on the role of PIs in the control of polar tip expansion of root hairs in Arabidopsis. The consistent results from several studies demonstrate that isoforms of PI 4-kinasesCitation26 and of PIP-kinasesCitation27,Citation28 reside in subcellular locations that suggest association with exocytotic vesicles. At the same time, the elimination of a root hair-specific PI-specific lipid transfer protein was also demonstrated to affect polar tip growth of root hairs in Arabidopsis.Citation25 Thus, while it is clear that independent pools of inositol-lipids are present in plant cellsCitation13,Citation14,Citation29 and that these pools may differ in fatty acid composition,Citation15 so far the mode of PtdIns distribution into different pools is unresolved.
Besides controlling the phosphorylation state of head groups in different PI-pools, plant PI-kinases and PIP-kinases likely also discriminate for certain PI substrate-species in a similar fashion as PI-synthases. PI-modifying enzymes have been shown to exhibit selective preferences for lipid substrates with certain fatty acid compositions, including invertebrate PIP-kinases and PLC,Citation30 and mammalian PI phosphatases.Citation31 Although no biochemical characterizations of substrate specificities with regard to fatty acid species has been reported for plant enzymes of PI metabolism other than PI-synthases, the available data suggest that different functional pools of PIs may be generated by enzymes that have preferences for precursor lipids based on the respective fatty acid patterns.
Consequences of Differences in Fatty Acid Composition on PI Function
The fatty acid make-up of lipids exerts profound influence on the lipid's biophysical properties and lateral mobility within a membrane,Citation32–Citation35 a concept also applicable to PIs.Citation36,Citation37 The simplified kinetic models describing the catalytic behavior of soluble enzymes do not apply for lipid-modification for a number of reasons, most importantly because the accessibility of a lipid substrate for an enzyme is restricted in space by its membrane-association. As a consequence, parameters defining the behavior of the substrate-membrane will have more impact on catalytic activity of lipid-modifying enzymes than, e.g., substrate concentration alone, as is commonly assumed for soluble enzymes. For instance, it has been demonstrated that the hydrolyzing activity of PLC against PtdIns(4,5)P2 depends to a much higher degree on the curvature of the substrate vesicles than on the concentration of PtdIns(4,5)P2 in the vesicles.Citation38 Because membrane curvature will depend largely on the lipid composition of the vesicles,Citation39,Citation40 an important component of future investigations into the functionality of lipid-modifying enzymes, will have to consider the composition of target-membranes for enzyme-action regarding non-substrate lipids. In this fashion, it may be possible to better link in vitro and in vivo data and move towards a functional understanding of the PI network in plants and other eukaryotes.
Outlook
The comprehensive analysis of regulatory PIs and particular effects of certain molecular species is still in its infancy. The understanding how the physiology of eukaryotic cells is regulated by PIs will progress with the advancement of ever-better analytical tools for PI analysis and the elucidation of lipid molecular species associating with different PI-pools. Because PI signaling is conserved in evolution across organismic kingdoms and affects a great multitude of cellular processes, knowledge about the organization and function of the PI-system is relevant for a broad range of biological questions. A number of recent studies has identified particular enzymes of PI metabolism that are involved in the control of key aspects of plant function and development.Citation25–Citation28,Citation41,Citation42 It is clear that PISs, in addition to other enzymes involved in the interconversion of polyphosphoinositides, will have to be given attention, as a distribution of PtdIns species into functional pools may occur already at the level of PtdIns biosynthesis. Future experiments will combine genetic tools and cell-biological methods with biochemical analysis to elucidate the molecular mechanisms underlying the function of the PI-network in plants.
Abbreviations
CDP-DAG | = | cytidinediphospho-diacylglycerol |
DAG | = | diacylglycerol |
ER | = | endoplasmic reticulum |
Ins(1,4,5)P3 | = | inositol-1,4,5-trisphosphate |
PI | = | polyphosphoinositide |
PI-kinase | = | PtdIns-kinase |
PIP-kinase | = | PtdIns-monophosphate kinase |
PI-LTP | = | phosphoinositide-specific lipid transfer protein |
PIS | = | PtdIns-synthase |
PLC | = | phospholipase C |
PM | = | plasma membrane |
PtdEtn | = | phosphatidylethanolamine |
PtdIns | = | phosphatidylinositol |
PtdIns3P | = | PtdIns-3-phosphate |
PtdIns4P | = | PtdIns-4-phosphate |
PtdIns(3,5)P2 | = | PtdIns-3,5-bisphosphate |
PtdIns(4,5)P2 | = | PtdIns-4,5-bisphosphate |
PtdOH | = | phosphatidic acid |
Figures and Tables
Figure 1 A model of functional phosphoinositide-pools in plant cells. All phosphoinositides originate from phosphatidylinositol (PI) formed by PI-synthases (PIS). The Arabidopsis isoenzymes, PIS1 and PIS2, differ in substrate specificity and localization, giving rise to PI-species with more saturated (bottom left) or more unsaturated fatty acids (bottom right). Localization of PIS1 and PIS2 with cis-Golgi and trans-Golgi sites is hypothetical. PI may be delivered to subcellular destinations by vesicle trafficking or by lipid transfer proteins. Left, Based on lipid patterns observed in Arabidopsis plants overexpressing PIS1,Citation23 PI formed by PIS1 may not enter the secretory pathway and instead support ER-associated PI-pools that may also be continuous with the nuclear envelope. In the absence of sufficient lipid transfer capacity, PI formed by overexpressed PIS1 may accumulate in the ER, followed by degradation to PA and increased PE production.Citation23 A physiological destination of PI-LTPs may be the plasma membrane, where intact saturated PI may be involved in the regulation of ion channels. Right, As PI-kinases and PIPkinases act on secretory vesicles and PIS2 overexpression resulted in increased formation of polyphosphoinositides,Citation23 PI formed by PIS2 may associate with trans-Golgi vesicles and experience partial conversion to PI4P and PIP2. PI delivered to the plasma membrane by vesicle-flow along F-actin strands may be converted by stress-activated PI-kinases and PIP-kinases; PI4P and PIP2 formed may serve as substrates for PLC and support IP3-production.Citation15 PI-LTPs may play a role in the delivery of unsaturated PI to subcellular destinations. For better clarity, short-hand abbreviations for the lipids were used for the model. ER, endoplasmic reticulum; F-A, filamentous actin; Nu, nucleus; PA, phosphatidic acid; PE, phosphatidylethanolamine; PI, phosphatidylinositol; PI4P, phosphatidylinositol-4-phosphate; PI-LTP, phosphoinositide-specific lipid transfer protein; PIP2, phosphatidylinositol-4,5-bisphosphate; PLC, phospholipase C; PM, plasma membrane; V, vesicle.
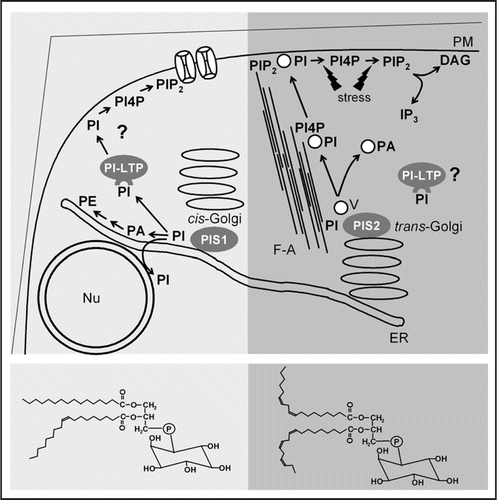
Acknowledgements
The author would like to thank Prof. Dr. Ivo Feussner for helpful discussion and acknowledges generous financial support through an Emmy Noether grant from the German Research Foundation (DFG) and the Göttingen Graduate School for Neurosciences and Molecular Biosciences (GGNB).
References
- Balla T. Phosphoinositide-derived messengers in endocrine signaling. J Endocrinol 2006; 188:135 - 153
- Meijer HJ, Munnik T. Phospholipid-based signaling in plants. Annu Rev Plant Biol 2003; 54:265 - 306
- Santarius M, Lee CH, Anderson RA. Supervised membrane swimming: small G-protein lifeguards regulate PIPK signalling and monitor intracellular PtdIns(4,5)P2 pools. Biochem J 2006; 398:1 - 13
- Stevenson JM, Perera IY, Heilmann I, Persson S, Boss WF. Inositol signaling and plant growth. Trends Plant Sci 2000; 5:252 - 258
- Suh BC, Hille B. Regulation of ion channels by phosphatidylinositol 4,5-bisphosphate. Curr Opin Neurobiol 2005; 15:370 - 378
- Cote GG, Yueh YG, Crain RC. Phosphoinositide turnover and its role in plant signal transduction. Subcell Biochem 1996; 26:317 - 343
- Wenk MR, De Camilli P. Protein-lipid interactions and phosphoinositide metabolism in membrane traffic: insights from vesicle recycling in nerve terminals. Proc Natl Acad Sci USA 2004; 101:8262 - 8269
- Wasteneys GO, Galway ME. Remodeling the cytoskeleton for growth and form: an overview with some new views. Annu Rev Plant Biol 2003; 54:691 - 722
- Doughman RL, Firestone AJ, Anderson RA. Phosphatidylinositol phosphate kinases put PI4,5P(2) in its place. J Membr Biol 2003; 194:77 - 89
- Lemmon MA, Ferguson KM, Abrams CS. Pleckstrin homology domains and the cytoskeleton. FEBS Lett 2002; 513:71 - 76
- Mueller-Roeber B, Pical C. Inositol phospholipid metabolism in Arabidopsis. Characterized and putative isoforms of inositol phospholipid kinase and phosphoinositide-specific phospholipase C. Plant Physiol 2002; 130:22 - 46
- Bunney TD, Watkins PA, Beven AF, Shaw PJ, Hernandez LE, Lomonossoff GP, Shanks M, Peart J, Drobak BK. Association of phosphatidylinositol 3-kinase with nuclear transcription sites in higher plants. Plant Cell 2000; 12:1679 - 1688
- Heilmann I, Perera IY, Gross W, Boss WF. Changes in phosphoinositide metabolism with days in culture affect signal transduction pathways in Galdieria sulphuraria. Plant Physiol 1999; 119:1331 - 1339
- Heilmann I, Perera IY, Gross W, Boss WF. Plasma membrane phosphatidylinositol 4,5-bisphosphate levels decrease with time in culture. Plant Physiol 2001; 126:1507 - 1518
- König S, Mosblech A, Heilmann I. Stress-inducible and constitutive phosphoinositide pools have distinct fatty acid patterns in Arabidopsis thaliana. FASEB J 2007; 21:1958 - 1967
- King CE, Stephens LR, Hawkins PT, Guy GR, Michell RH. Multiple metabolic pools of phosphoinositides and phosphatidate in human erythrocytes incubated in a medium that permits rapid transmembrane exchange of phosphate. Biochem J 1987; 244:209 - 217
- Augert G, Blackmore PF, Exton JH. Changes in the concentration and fatty acid composition of phosphoinositides induced by hormones in hepatocytes. J Biol Chem 1989; 264:2574 - 2580
- König S, Hoffmann M, Mosblech A, Heilmann I. Determination of content and fatty acid composition of unlabeled phosphoinositide species by thin layer chromatography and gas chromatography. Anal Biochem 2008; 197 - 201
- Perera IY, Heilmann I, Chang SC, Boss WF, Kaufman PB. A role for inositol 1,4,5-trisphosphate in gravitropic signaling and the retention of cold-perceived gravistimulation of oat shoot pulvini. Plant Physiol 2001; 125:1499 - 1507
- Mosblech A, König S, Stenzel I, Grzeganek P, Feussner I, Heilmann I. Phosphoinositide and inositolpolyphosphate-signaling in defense responses of Arabidopsis thaliana challenged by mechanical wounding. Mol Plant 2008; 1:249 - 261
- Collin S, Justin AM, Cantrel C, Arondel V, Kader JC. Identification of AtPIS, a phosphatidylinositol synthase from Arabidopsis. Eur J Biochem 1999; 262:652 - 658
- Justin AM, Kader JC, Collin S. Phosphatidylinositol synthesis and exchange of the inositol head are catalysed by the single phosphatidylinositol synthase 1 from Arabidopsis. Eur J Biochem 2002; 269:2347 - 2352
- Löfke C, Ischebeck T, Konig S, Freitag S, Heilmann I. Alternative metabolic fates of phosphatidylinositol produced by PI-synthase isoforms in Arabidopsis thaliana. Biochem J 2008; 413:115 - 124
- Phillips SE, Vincent P, Rizzieri KE, Schaaf G, Bankaitis VA, Gaucher EA. The diverse biological functions of phosphatidylinositol transfer proteins in eukaryotes. Crit Rev Biochem Mol Biol 2006; 41:21 - 49
- Vincent P, Chua M, Nogue F, Fairbrother A, Mekeel H, Xu Y, Allen N, Bibikova TN, Gilroy S, Bankaitis VA. A Sec14p-nodulin domain phosphatidylinositol transfer protein polarizes membrane growth of Arabidopsis thaliana root hairs. J Cell Biol 2005; 168:801 - 812
- Preuss ML, Schmitz AJ, Thole JM, Bonner HK, Otegui MS, Nielsen E. A role for the RabA4b effector protein PI-4Kbeta1 in polarized expansion of root hair cells in Arabidopsis thaliana. J Cell Biol 2006; 172:991 - 998
- Stenzel I, Ischebeck T, König S, Holubowska A, Sporysz M, Hause B, Heilmann I. The type B phosphatidylinositol-4-phosphate 5-kinase 3 is essential for root hair formation in Arabidopsis thaliana. Plant Cell 2008; 20:124 - 141
- Kusano H, Testerink C, Vermeer JEM, Tsuge T, Shimada H, Oka A, Munnik T, Aoyama T. The Arabidopsis phosphatidylinositol phosphate 5-kinase PIP5K3 is a key regulator of root hair tip growth. Plant Cell 2008; 20:367 - 380
- Kost B, Lemichez E, Spielhofer P, Hong Y, Tolias K, Carpenter C, Chua NH. Rac homologues and compartmentalized phosphatidylinositol 4, 5-bisphosphate act in a common pathway to regulate polar pollen tube growth. J Cell Biol 1999; 145:317 - 330
- Carricaburu V, Fournier B. Phosphoinositide fatty acids regulate phosphatidylinositol 5-kinase, phospholipase C and protein kinase C activities. Eur J Biochem 2001; 268:1238 - 1249
- Schmid AC, Wise HM, Mitchell CA, Nussbaum R, Woscholski R. Type II phosphoinositide 5-phosphatases have unique sensitivities towards fatty acid composition and head group phosphorylation. FEBS Lett 2004; 576:9 - 13
- Janmey PA, Kinnunen PK. Biophysical properties of lipids and dynamic membranes. Trends Cell Biol 2006; 16:538 - 546
- Mukherjee S, Maxfield FR. Role of membrane organization and membrane domains in endocytic lipid trafficking. Traffic 2000; 1:203 - 211
- Kooijman EE, Chupin V, de Kruijff B, Burger KN. Modulation of membrane curvature by phosphatidic acid and lysophosphatidic acid. Traffic 2003; 4:162 - 174
- Mukherjee S, Soe TT, Maxfield FR. Endocytic sorting of lipid analogues differing solely in the chemistry of their hydrophobic tails. J Cell Biol 1999; 144:1271 - 1284
- Cho H, Kim YA, Ho WK. Phosphate number and acyl chain length determine the subcellular location and lateral mobility of phosphoinositides. Mol Cells 2006; 22:97 - 103
- Schneiter R, Brugger B, Amann CM, Prestwich GD, Epand RF, Zellnig G, Wieland FT, Epand RM. Identification and biophysical characterization of a very-long-chain-fatty-acidsubstituted phosphatidylinositol in yeast subcellular membranes. Biochem J 2004; 381:941 - 949
- Ahyayauch H, Villar AV, Alonso A, Goni FM. Modulation of PI-specific phospholipase C by membrane curvature and molecular order. Biochemistry 2005; 44:11592 - 11600
- Cooke IR, Deserno M. Coupling between lipid shape and membrane curvature. Biophys J 2006; 91:487 - 495
- Farsad K, De Camilli P. Mechanisms of membrane deformation. Curr Opin Cell Biol 2003; 15:372 - 381
- Lee Y, Kim YW, Jeon BW, Park KY, Suh SJ, Seo J, Kwak JM, Martinoia E, Hwang I. Phosphatidylinositol 4,5-bisphosphate is important for stomatal opening. Plant J 2007; 52:803 - 816
- Leshem Y, Seri L, Levine A. Induction of phosphatidylinositol 3-kinase-mediated endocytosis by salt stress leads to intracellular production of reactive oxygen species and salt tolerance. Plant J 2007; 51:185 - 197