Abstract
Calcium (Ca2+) transients have been shown to take place in response to diverse developmental and physiological cues. Also, it is involved in biotic and abiotic stress signaling. Nitric oxide (NO) is an important signaling molecule that plays a crucial role in plant growth and development, starting from germination to flowering, ripening of fruit and senescence of organs. Moreover, it plays a pivotal role in several biotic and abiotic stress signaling processes. In the present work, the ability of NO to trigger increases in cytosolic calcium concentration ([Ca2+]cyt) was investigated. For this purpose, transgenic Arabidopsis seedlings constitutively expressing the luminescent Ca2+-sensitive protein apoaequorin (35S::APOAEQUORIN) was employed. In chemiluminescence and in vivo Ca2+ imaging assays, the NO-donor sodium nitroprusside (SNP) brought about a strong, instantaneous, reproducible, and dose-dependent rise in [Ca2+]cyt. Moreover, the observed rise in [Ca2+]cyt was shown to be NO-specific and not associated with decomposition products of SNP, as the NO-scavenger 2-4-carboxyphenyl-4,4,5,5-tetramethylimidazoline-1-oxyl-3 oxide (C-PTIO) significantly blunted the observed NO-mediated spike in [Ca2+]cyt. Interestingly, preincubation of 35S::APOAEQUORIN Arabidopsis seedlings with the plasma membrane channel blocker lanthanum chloride resulted in partial concentration-dependent blocking of the NO-specific Ca2+ transient. This observation indicates that, in addition to the mobilization of [Ca2+]cyt, as an external source in response to NO treatment, an appreciable contribution of yet unidentified internal pools also exist.
Introduction
Calcium (Ca2+) is deemed to be one of the most important second messengers in many signal transduction networks in all living organisms, including animals and plants. In animal cells, Ca2+-mediated signal transduction pathways have been shown to be enormously versatile and complex in the temporal and spatial organisation of the signal, as well as in the proteins that produce and intercept it.Citation1 Equally, plants possess signal transduction networks that are highly conserved in terms of components which are also as sophisticated as that in their mammalian counterparts.Citation2 In the resting (un-stimulated) state, the cytosolic free Ca2+ concentration [Ca2+]cyt is ∼200 nM and most of the cellular calcium is sequestered in cytoplasmic organelles such as the vacuole (100 mM), endoplasmic reticulum (ER) (1 mM), or the cell wall (1 mM).Citation3 This difference of many orders of magnitude in free Ca2+ concentrations between the cytoplasm and cytosolic compartments creates a large electrochemical Ca2+ gradient (200–300 mV), across the plasma membrane (PM), ER, and tonoplast that favours the energy-independent (downhill) movement of Ca2+ into the cytosol (influx). This movement is controlled by a diverse set of specialized Ca2+ channels and pores. Conversely, the movement of Ca2+ against this electrochemical gradient, from the cytoplasm into the stores (efflux), is energy-dependent, and, requires ATP or proton motive force and is provided by specialized Ca2+-ATPase pumps. Both types of proteins, channels and pumps, are abundant in the PM and tonoplast and also occur in the ER, mitochondria and chloroplasts.Citation3,Citation4
The channels that are responsible for Ca2+ influx into the cytosol are gated by either voltage, stretch, inositol triphosphate (IP3), cADPR or G-proteins. Ca2+-mediated signaling takes place when some of these channels open, allowing Ca2+ to move down the electrochemical gradient.Citation4 A single channel can conduct 10Citation6 Ca2+ atoms per second; thus, [Ca2+]cyt can increase rapidly. On the other hand, subsequent activation of membrane-bound Ca2+-ATPases (or Ca2+/H+ exchange proteins) in the ER, vacuolar, mitochondrial, or PM ensures that most [Ca2+]cyt signals are transients.Citation3,Citation5 In plants, Ca2+ or Ca2+ fluxes function in many biochemical and processes including: red light, abscisic acid, gibberellin, salinity/drought, hypo-osmotic stress, touch, cold, heat shock, and nodulation factors.Citation6–Citation8 Moreover, Ca2+ fluxes play a pivotal role in developmental and physiological processes such as: egg cell fertilisation,Citation9 pollen tube elongation,Citation10 circadian rhythms,Citation11 oxidative stressCitation12,Citation13 and pathogen infection.Citation14
The ability of a plant cell to differentiate among various Ca2+ signals to activate the correct set of cellular responses is intriguing. Each signal creates its own Ca2+ fingerprint that is unique in the lag period, frequency and amplitude of the Ca2+ wave, and its spatial distribution. The targets of Ca2+ signal transduction can be divided into two categories: primary sensors and downstream substrates. Phosphorylation cascades regulated by protein kinases and phosphatases represent the primary transduction route interpreting the [Ca2+]cyt signal. Ca2+-dependent protein kinase (CDPK), Ca2+/Calmodulin (CaM)-dependent protein kinase (CaM kinase) and a protein kinase C-type enzyme have all been shown to be present in plants with primary role in Ca2+sensing and decoding. When bound to Ca2+, CaM activates over 100 proteins, most of which have not been characterized. The activities of several enzymes including myosin V, kinesin, Ca2+-ATPase, Ca2+ channels, glutamate carboxylase, NAD kinase, and a number of protein kinases are all calmodulin dependent in plant cells.Citation15,Citation16
In animal cells, nitric oxide (NO) is a wellknown potent inducer of calcium transients. It has been demonstrated the ability of sodium nitroprusside (SNP), an NO donor, and to elicit dose-dependent Ca2+ fluxes in human neutrophiles.Citation17 Moreover, it has been indicated that exogenous NO donor SNP induced the release of Ca2+ from intracellular IP(3) receptorsensitive stores of respiratory burst neutrophils via S-nitrosylation.Citation18 To this end, NO was found to play a crucial role in plant growth and development, starting from germination to flowering, ripening of fruit and senescence of organs. Also in case of environmental stress hazard, caused by both abiotic and biotic factors, enhanced NO generation is observed in different plant species and organs.Citation19–Citation23
Taken together, an increasing body of evidence points to a role for NO in plant development, stress responses, and programmed cell death,Citation19–Citation23 although its situation within any one signal cascade is still poorly understood. Moreover, in plant systems, a comprehensive knowledge on the ability of NO to induce Ca2+ fluxes and the potential contributing cellular sources is incomplete. Therefore, this work was primarily focused on the investigation of the ability of NO to induce Ca2+ transients via the utilisation of transgenic Arabidopsis thaliana seedlings, expressing the Ca2+-sensitive reporter apoaequorin. The obtained results are discussed in the light of the specificity, dose-dependence and the possible cellular sources of the obtained NO-mediated Ca2+ transients.
Results
Ca2+ transients follow a dose-dependent fashion in response to NO.
Arabidopsis seedlings were employed to study the changes in [Ca2+]cyt levels in response to the NO-donor SNP. Elevations in luminescence responses or relative luminescence units (RLU) counts were interpreted as a faithful reporter of increases in [Ca2+]cyt levels. illustrates the results obtained from the dose-response experiments between SNP and luminescent light. An instantaneous spike, with a maximum RLU value of ∼1500000, was observed when a 0.1 mM SNP solution was injected (). Moreover, the observed RLU counts almost doubled when the reconstituted aequorin seedlings were treated with a ten-time more concentrated SNP solution (i.e., 1.0 mM) (). Furthermore, the increase in the RLU value in response to increasing SNP concentrations was maintained, albeit to a lesser extent (only ∼1/6 increase), when a solution of 10 mM SNP was administered (). The observed dose-dependency was reproducible in several independent experiments. Irrespective of NO concentration, a single instantaneous transient spike of Ca2+-dependent luminescence was observed. Moreover, the overall increase in [Ca2+]cyt was transient, lasting between 1 and 2 s, even though the seedlings remained immersed in the solution of SNP.
To examine whether the observed Ca2+ spike is due to a direct reaction between aequorin and SNP, apoaequorin was in vitro reconstituted and its bioluminescence counts were integrated over 10 s upon treatment with 10 mM SNP. Typically, the reconstituted aequorin gave a luminescence value of ∼150000 when treated with 25 mM CaCl2, whereas a background luminescence value averaging around 30 was obtained when the reconstituted aequorin was treated with sterile water at room temperature (data not shown). Moreover, when the reconstituted aequorin was treated with a 10 mM solution of SNP, luminescence values around 100 were consistently obtained. Furthermore, the 10 mM SNP solution in the absence of aequorin gave similar values to that obtained in the presence of aequorin (i.e., 100) (data not shown).
SNP-induced [Ca2+]cyt increase is partially contributed by PM channels.
Lanthanum chloride (La3+) is a well known plasma membrane channel blocker.Citation26 To investigate the contribution of PM channels towards the observed elevation in [Ca2+]cyt, in response to SNP challenge 35S::AEQUORIN seedlings were pretreated with increasing concentrations of La3+. represents luminescence traces obtained upon La3+-pretreated seedlings that were subsequently challenged with a 1.0 mM SNP solution. A one order-of-magnitude reduction was observed when seedlings were pretreated with a 10 µM La3+ (). Moreover, a two order-of-magnitude inhibition was obtained when seedlings were treated with a ten-time more concentrated La3+ solution (100 µM) (). No significant inhibitions in [Ca2+]cyt spike's magnitude were observed when more concentrated La3+ solutions were tested (data not shown).
[Ca2+]cyt increase induced by SNP is NO-specific.
To investigate whether the observed increase in [Ca2+]cyt is associated with NO and not with SNP, we tested the impact of the SNP non-functional analogue potassium ferrocyanide {K4Fe(CN)6}, on the induction of Ca2+ spikes. Treatment with a 1.0 mM solution of {K4Fe(CN)6} induced only a weak spike that was three order-of-magnitudes weaker than that induced by 1.0 mM SNP ( and B). Moreover, we tested the effect of the NO scavenger 2-4-carboxyphenyl-4,4,5,5-tetramethylimidazoline-1-oxyl-3-oxide (C-PTIO) on the observed SNP-induced Ca2+ spike. A significant two-order-reduction in the magnitude of the 1.0 mM SNP-induced Ca2+ spike was obtained when Arabidopsis seedlings were pretreated with 150 µM C-PTIO ( and D). Approximately, a three order-of-magnitudes reduction was observed when a two-time more concentrated C-PTIO solution (300 µM) was used (data not shown). Moreover, in vivo Ca2+ imaging experiments indicated that infiltrating leaves of adult Arabidopsis plants with 1 mM SNP strongly induced a bioluminescence response. The observed bioluminescence was strongly blunted when leaves were preinfiltrated with 150 µM C-PTIO ( and F). Leaves infiltrated with sterile water exhibited an undetectable bioluminescent response (image not shown).
Discussion
The use of transgenic plants that have been transformed with the soluble, calcium-sensitive, luminescent protein aequorin has significantly contributed to the advancement of our knowledge of the signals that trigger increase in [Ca2+]cyt levels.Citation27 Aequorin is a Ca2+-activated photoprotein normally produced by the marine jellyfish Aequoria victoria. The protein consists of a single polypeptide chain, apoaequorin. Coelenterazine, a hydrophobic luminophore, converts apoaequorin into an active calcium-sensitive aequorin. When Ca2+ ions bind to this protein, the lumniphore is discharged (oxidized) and a finite amount of blue light is emitted in a dose-dependent manner.Citation26 In this work, apoaequorin-expressing Arabidopsis plants were employed to examine the involvement of Ca2+ in NO signal transduction. Several chemical compounds have previously been reported to act as donor for NO. These include S-nitrosothiols (e.g., SNAP) and nitroprussides (e.g., SNP). The SNP anion [Fe(CN)5(NO)]2−, with an NO+ nitrosyl group, is an effective nitrosating agent to S and N nucleophiles at neutral pH values. The product may then decompose to release NO and oxidized thiol.Citation28 Thus, SNP has been previously used as a potential NO donor to study NO-mediated signaling in mammalian and plant systems.Citation17,Citation29,Citation30 Therefore, SNP was utilized as the NO-donor in all experiments. Previously, luminescence response has been successfully used as a satisfactory parameter for qualitative analysis of changes in [Ca2+]cyt in response to oxidative signals.Citation12 Therefore, in this work we solely relied on the use of relative luminescence units (RLU) counts. Results indicated that NO is capable of triggering a strong monophasic Ca2+ pulse in a dose-dependent manner (). Interestingly, the average amplitude of the bioluminescent signal exceeded that produced in response to both cold shock and hydrogen peroxide (H2O2) treatments. Precisely, the average amplitude of the Ca2+ spike obtained upon treatment with 10 mM SNP was approximately 500 and 60 times greater than that observed upon either H2O2 or cold shock treatments, respectively.Citation12 However, despite being different in amplitude, the patterns of the induced [Ca2+]cyt, in response to H2O2, cold shock and NO, are similar in that they are all monophasic. Conversely, it has previously been reported that hypo-osmotic shock induces a distinctively different elevation in [Ca2+]cyt, which is biphasic.Citation31 Moreover, the observed [Ca2+]cyt spike in response to NO challenge was similar to that obtained upon cold shock treatment, in the fact that it was instantaneous, with no apparent lag period (). The reported [Ca2+]cyt spike obtained upon H2O2 treatment has been shown to follow a definite lag period of 20–40 s lasting between 1–2 min.Citation12 Furthermore, the decay time in the NO-induced luminescent signal was comparable with a shorter transient signal lasting only between 3–5 s. Taken together, it is envisaged that these apparent qualitative and quantitative differences in [Ca2+]cyt dynamics, between different stimuli, underline the uniqueness of each [Ca2+]cyt transient in transducing a distinct signal. This observation conforms to the current knowledge that the lag period length before the transient starts, the rise time to the transient peak and the decay time back to the resting level are all unique parameters to each Ca2+ signal.Citation16
It was conceivable that the observed luminescence emission was not brought about by NO but rather via a direct chemical modification of aequorin by SNP or one of its byproducts. To evaluate this possibility, a combination of a SNP non-functional analogue and NO scavenger was employed. Any direct interaction between SNP and aequorin was ruled out by demonstrating that SNP was not capable of inducing any significant light emissions when added to in vitro reconstituted aequorin. Moreover, the SNP non-functional analogue {K4Fe(CN)6} was shown to induce negligible luminescence in chemiluminometer assay (). Furthermore, the NO scavenger C-PTIO significantly blunted the SNP-induced [Ca2+]cyt spike in both chemiluminometer and in vivo Ca2+ imaging assays (). Taken together, these results suggest that the observed SNP-mediated spike in [Ca2+]cyt is specific to NO. Thus, based on the obtained experimental evidence ( and ), the NO capacity of inducing [Ca2+]cyt spikes in plants was clearly indicated. These results are in agreement with previously reported studies providing strong evidence that NO regulates cytosolic Ca2+ homeostasis in plant cells.Citation22
Previously, pharmacological studies have been successfully employed to identify the sources of observed Ca2+ transients induced in response to several stimuli.Citation12,Citation21,Citation31 In this work, the use of a Ca2+ channel inhibitor proved to be useful. The PM-channel blocker La3+ was utilized, which acts as a potent Ca2+ antagonist, to assess the contribution of external Ca2+ pools towards the observed NO-induced [Ca2+]cyt spike. La3+ treatment caused a concentration-dependent inhibition in [Ca2+]cyt spike. However, complete abolition of the luminescent signal was not evident (). Moreover, La3+ concentrations above 100 µM resulted in no further significant inhibition. Thus, it can be concluded that the NO-mediated elevation in [Ca2+]cyt is only partially contributed by an external Ca2+ pool or source. Taken together, it can be concluded that the observed elevation in [Ca2+]cyt can partially be accounted for by a contribution from external Ca2+ pool and might possibly be from PM channels. Moreover, the observation that the inhibition in spike's magnitude is only partial suggests that there are, as yet unidentified, other sources of Ca2+ giving rise to the observed SNP-induced increases in [Ca2+]cyt. In contrast, employing a combination of La3+ and ruthenium red (a mitochondrial channel blocker), it has been shown that H2O2 is capable of mobilizing Ca2+ from both external and internal pools, with a major contribution from the internal pools.Citation12 Conversely, cold shock has been reported to mobilize Ca2+ primarily from external pools.Citation12 Interestingly, hypo-osmotic shock has been reported to induce a biphasic [Ca2+]cyt influx. The first influx has been shown to derive from external Ca2+ stores, whereas the second influx derives from internal ones [31]. These results suggest that NO, H2O2, cold shock and hypo-osmotic shock mobilize different Ca2+ pools. Elegant studies employing Nicotiana plumbaginifolia cells expressing the Ca2+ reporter apoaequorin subjected to hyperosmotic stress, showed that NO was able to activate both PM and intracellular Ca2+-permeable channels via signaling cascades; involving PM depolarization, cADPR, and protein kinases.Citation21 Recently, Lamattina and co-workers, via employing guard cell systems of Arabidopsis and cucumber, have made significant contributions that deepened our understanding of the involvement of NO in Ca2+ signaling. ABA has been shown to trigger a complex sequence of signaling events that lead to concerted modulation of ion channels at the plasma membrane of guard cells and solute efflux to drive stomatal closure in plant leaves. In this context, recent work has indicated that (NO) and its synthesis are a prerequisite for ABA signal transduction and stomatal closure in Arabidopsis and Vicia faba guard cells.Citation32 Latest results in Vicia faba guard cells have revealed that both Ca2+- and NO-mediated signaling pathways are implicated in the observed ABA inhibition of light-induced stomatal opening.Citation33 Moreover, in Vicia faba guard cells it has been shown that NO selectively regulates Ca2+-sensitive ion channels by promoting release from intracellular stores to raise [Ca2+]cyt. via a cADPR-dependent endomembrane Ca2+ channels, mediated through the action of a cGMP-dependent cascade.Citation34 Interestingly, protein kinase has been implicated as an essential component for intracellular Ca2+ release and ion channel control by NO and ABA in guard cells. In this context, it has been demonstrated that NO-sent signals can be modulated through protein phosphorylation, located upstream of intracellular Ca2+ release.Citation35 Similarly, it has been reported that Ca2+ and Ca2+-dependent protein kinases are involved in NO- and auxin-induced adventitious root formation in cucumber.Citation36
In the future, it will be interesting to investigate the contribution of internal Ca2+ pools towards the observed NO-induced [Ca2+]cyt spike.
Materials and Methods
Aequorin chemiluminescence measurement.
Transgenic Col-0 Arabidopsis plants genetically transformed with a chimeric gene in which the cauliflower mosaic virus 35S promoter was fused to the apoaequorin coding region were used. Seeds of this transgenic reporter line were a kind gift of Prof. Tony Trewavas (University of Edinburgh). Two-week old homozygous 35S::APOAEQUORIN seedlings were aequorin-reconstituted by floating into a cuvette containing 0.5 ml of 10 µM coelenterazine solution (prepared in sterile water) at room temperature in the dark overnight.Citation24 Four seedlings per cuvette were systematically used for each treatment. Then, reconstituted seedlings, floating in the cuvette, were treated with the NO donor sodium nitroprusside (SNP) at different concentrations. Increasing concentrations of SNP, between 0.1–10 mM, prepared in water, were injected via a syringe, fitted with a special needle, into the cuvette through a designated rubber-wrapped luminometer port. Chemicals such as lanthanum chloride (LaCl3) (a general plasma membrane channel blocker), potassium ferrocyanide {K4Fe(CN)6}, and 2-4-carboxyphenyl-4,4,5,5-tetramethylimidazoline-1-oxyl-3 oxide (C-PTIO) (Calbiochem@), were added to the coelenterazine-reconstituted seedlings 4 h before the treatment with SNP. To check whether SNP interacts with apoaequorin producing inappropriate luminescence, apoaequorin was reconstituted in vitro. Apoaequorin was in vitro reconstituted and extracted from 35S::APOAEQUORIN Arabidopsis seedlings in a buffer containing 0.5 M NaCl, 5 mM b-mercaptoethanol, 5 mM EDTA, 0.1% gelatine (w/v), 10 mM Tris-HCl pH 7.4 and 10 µM coelenterazine in the darkness for 4 h. Luminescence measurements were made using a digital chemiluminometer with a photomultiplier, model 9757 AM (THORN EMI Electron Tubes Limited, Ruislip, Middlesex, UK) at 1 kV with a discriminator.Citation25 In order to minimize background counts, temperature of the cuvette chamber was continuously kept at approximately −30°C by means of an installed cooler. The cuvette temperature was kept at room temperature. Luminescence counts were measured every 1 s for the duration of the experiment (∼2 min).
In vivo Ca2+ imaging.
Four-week old Arabidopsis 35S::APOAEQOURIN plants were reconstituted by infiltrating the abaxial side of the leaf with 10 µM coelenterazine solution and incubation in the dark overnight. The next day, reconstituted leaves were infiltrated with SNP. Immediately after infiltration, leaves were detached and placed in the dark box for imaging. Leaves were imaged within 1 min after infiltration and placement in the camera darkbox. Imaging was performed using an ultra low light imaging camera system, EG&G Luminograph 980, (Berthold, London, UK). Images were collected over a three-minute accumulation period and integrated for 1.5 min. Images were processed using Confocal Assistant and PaintShop Pro software.
Figures and Tables
Figure 1 Dose dependency of the Ca2+ transient induced by the NO donor SNP. SNP 0.1 mM (A), 1 mM (B) and 10 mM (C). Traces shown best represent the average response for each concentration Treatments were repeated at least three times with similar results.
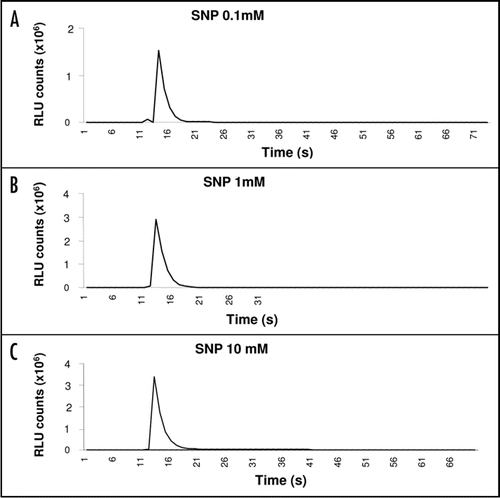
Figure 2 La3+ significantly diminishes the SNP-induced Ca2+ transient. Leaves were incubated with La3+ 10 µM (B) and 100 µM (C) for 4 hrs before SNP injection. (D) The superimposition of (A and B).
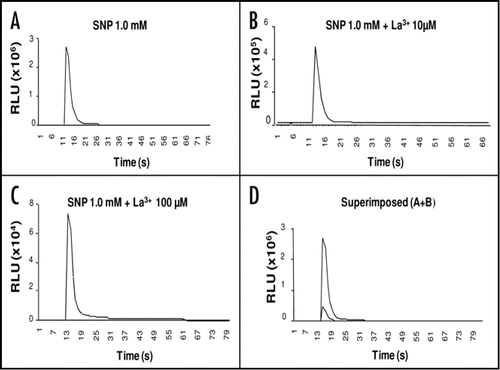
Figure 3 The effect of NO scavengers non-functional analogues on SNP-mediated Ca2+ transient. Treatment with 1.0 mM {K4Fe(CN)6} results in weak Ca2+ transient (B) when compared with the strong SNP-mediated Ca2+ transient (A). Seedlings preincubated with 150 µM C-PTIO (C). In vivo Ca2+ imaging, with an ultra low light camera, of a leaf treated with 1.0 mM SNP (E) and SNP treatment of a leaf that was pretreated with 150 µM C-PTIO (F).
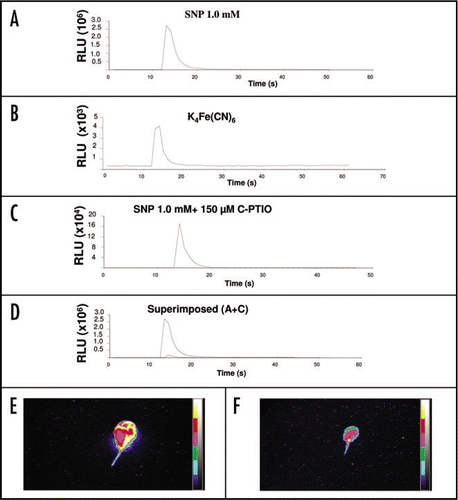
References
- Berridge MJ. Inositol triphosphate and calcium signaling. Nature 1993; 361:315 - 325
- Chrispeels MJ, Holuigue L, Latorre R, Luan S, Orellana A, Peña-Cortes H, et al. Signal transduction networks and biology of plant cells. Biol Res 1999; 32:35 - 60
- Bush DS. Calcium regulation of in plant cells and its role in signaling. Annu Rev Plant Physiol Plant Mol Biol 1995; 46:95 - 122
- Ward JM, Pei ZM, Schroeder JI. Roles of ion channels in initiation of signal transduction in higher plants. Plant Cell 1995; 7:833 - 844
- Poovaiah BW, Reddy ASN. Calcium and signal transduction in plants. Cri Rev Plant Sci 1993; 12:185 - 211
- Malho R. Spatial characteristics to calcium signalling; the calcium wave as a basic unit in plant cell calcium signalling. Phil Trans R Soc Lond B 1998; 353:1463 - 1473
- Sanders D. Communicating with calcium. Plant Cell 1999; 11:691 - 706
- Trewavas A. Le calcium, C'est la Vie: Calcium makes waves. Plant Physiol 1999; 120:1 - 6
- Digonnet C, Aldon D, Leduc N, Dumas C, Rougier M. First evidence of a calcium transient in flowering plants at fertilization. Development 1998; 124:2867 - 2874
- Pierson ES, Miller DD, Callaham DA, van Aken J, Hackett G, Hepler PK. Tiplocalized calcium entry fluctuates during pollen tube growth. Developmental Biol 1996; 174:160 - 173
- Johnson CH, Knight MR, Kondo T, Masson P, Sedbrook J, Haley A, et al. Circadian oscillations of cytosolic and chloroplastic free calclium in plants. Science 1995; 269:1863 - 1865
- Price AH, Taylor A, Ripley SJ, Griffiths A, Trewavas AJ, Knight MR. Oxidative signals in tobacco increase cytosolic calcium. Plant Cell 1994; 6:1301 - 1310
- Levine A, Pennell RI, Alvarez ME, Palmer R, Lamb C. Calcium-mediated apoptosis in plant hypersensitive disease resistance response. Curr Biol 1996; 6:427 - 437
- Xu H, Heath MC. Role of calcium in signal transduction during the hypersensitive response caused by basidiospore-derived infection of the cowpea rust fungus. Plant Cell 1998; 10:585 - 597
- Trewavas AJ, Malho R. Signal perception and transduction: The origin of the phenotype. Plant Cell 1997; 9:1181 - 1195
- Trewavas AJ, Malho R. Ca2+ signaling in plant cells: the big network!. Curr Opin Plant Biol 1998; 1:428 - 433
- Loitto VM, Nilsson H, Sundqvist T, Magnusson KE. Nitric oxide induces dosedependent Ca2+ transients and causes temporal morphological hyperpolarization in human neutrophils. J Cell Physiol 2000; 182:402 - 413
- Pan L, Zhang X, Song K, Wu X, Xu J. Exogenous nitric oxide-induced release of calcium from intracellular IP3 receptor-sensitive stores via S-nitrosylation in respiratory burst-dependent neutrophiles. Biochem Biophys Res Commun 2008; 377:1320 - 1325
- Gould KS, Lamotte O, Klinguer A, Pugin A, Wendehenne D. Nitric oxide production in tobacco leaf cells: A generalized stress response?. Plant Cell Environ 2003; 26:1851 - 1862
- Lamotte O, Gould K, Lecourieux D, Sequeira-Legrand A, Lebrun-Garcia A, Durner J, et al. Analysis of nitric oxide signaling functions in tobacco cells challenged by the elicitor cryptogein. Plant Physiol 2004; 135:516 - 530
- Lamotte O, Courtois C, Dobrowolska G, Besson A, Pugin A, Waendehenne D. Mechanism of nitric-oxide-induced increase of free cytosolic Ca2+ concentration in Nicotiana plumbaginifolia cells. Free Radic Biol Med 2006; 40:1369 - 1376
- Vandelle E, Poinssot B, Wendehenne D, Bente'jac M, Pugin A. Integrated signaling network involving calcium, nitric oxide, and active oxygen species but not mitogen-activated protein kinases in BcPG1-elicited grapevine defences. Mol Plant Microbe Interact 2006; 19:429 - 440
- Arasimowicz M, Floryszak-Wieczorek J. Nitric oxide as a bioactive signaling molecule in plant stress responses. Plant Science 2007; 172:876 - 887
- Knight MR, Read ND, Campbell AK, Trewavas AJ. Imaging calcium dynamics in living plants using semi-synthetic recombinant aequorins. J Cell Biol 1993; 121:83 - 90
- Campbell AK. Interacellular calcium-its universal role as regulator 1983; Chichester, UK Wiley 556 pp
- Tester M. Plant ion channels—Whole cell and single channel studies. New Phytol 1990; 114:305 - 340
- Knight MR, Campbell AK, Smith SM, Trewavas AJ. Transgenic plant aequorin reports the effects of cold shock and elicitors on cytoplasmic calcium. Nature 1991; 352:524 - 526
- Poole RK, Hughes MN. New functions for the ancient globin family: bacterial responses to nitric oxide and nitrosative stress. Mol Microbiol 2000; 36:775 - 783
- Delledonne M, Xia Y, Dixon RA, Lamb C. Nitric oxide functions as a signal in plant disease resistance. Nature 1998; 394:585 - 588
- Durner J, Wendehenne D, Klessig DF. Defense gene induction of tobacco by nitric oxide, cyclic GMP and cyclic ADP-ribose. Proc Natl Acad Sci USA 1998; 95:10328 - 10333
- Cessna S, Chandra S, Low PS. Hypo-osmotic shock of tobacco cells stimulates Ca2+ fluxes deriving first from external and then internal Ca2+ stores. J Biol Chem 1998; 273:27286 - 27291
- García-Mata C, Lamattina L. Abscisic acid, nitric oxide and stomatal closure—Is nitrate reductase one of the missing links?. Trends Plant Sci 2003; 8:314 - 315
- Garcia-Mata C, Lamattina L. Abscisic acid (ABA) inhibits light-induced stomatal opening through calcium- and nitric oxide-mediated signaling pathways. Nitric Oxide 2007; 17:143 - 151
- Garcia-Mata C, Gay R, Sokolovski S, Hills A, Lamattina L, Blatt MR. Nitric oxide regulates K+ and Cl− channels in guard cells through a subset of abscisic acid-evoked signaling pathways. Proc Natl Acad Sci USA 2003; 100:11116 - 11121
- Sokolovski S, Hills A, Gay R, Garcia-Mata C, Lamattina L, Blatt MR. Protein phosphorylation is a prerequisite for intracellular Ca2+ release and ion channel control by nitric oxide and abscisic acid in guard cells. Plant J 2005; 43:520 - 529
- Lanteri ML, Pagnussat GC, Lamattina L. Calcium and calcium-dependent protein kinases are involved in nitric oxide- and auxin-induced adventitious root formation in cucumber. J Exp Bot 2006; 57:1341 - 1351