Abstract
Our vision of plants is changing dramatically: from insensitive and static objects to complex living beings able to sense the environment and to use the information collected to adapt their behavior. At all times humans imitate ideas and concepts from nature to resolve technological problems. Solutions coming from plants have the potential to face challenges and difficulties of modern engineering design. Characteristic concepts of the plant world such as reiteration, modularity and swarm behavior could be of great help resolving technological problems. On the other hand a biorobotic approach would facilitate the resolution of many biological problems. In this paper, the concept of a plant-inspired robot is proposed for the investigation of both biological and technological issues.
Somewhere between 400–1,000 million years ago, plants (and some animals such as corals) initiated a sessile lifestyle, taking advantage of the ubiquity of light as a source of energy as well as devising ways of compensating for body losses suffered because of browsing predators. Among the primary advances made by plants and sessile animals to survive predation was the evolution of different modular structures.Citation1 Roots, leaves, branches, shoots, buds, flowers, are reiterated many times during the development of a single plant body, to ensure that in case of environmental damage or predation some module of the body can survive and regenerate the individual. In general, as a consequence of this primordial decision for a sessile and modular lifestyle, the specialization of tissues and cells in plants is minimized, if compared with animals, to limit predatory damages.
Another consequence of the “sessile decision” was the need of a well-organized sensing system which allows plants to explore efficiently the environment and to react rapidly to potential dangerous circumstances. Below ground, roots can sense a multitude of abiotic and biotic signals, providing all the time the appropriate responses. Actually, roots behave almost like active animals,Citation2,Citation3 performing efficient exploratory movements, with the root apices that drive the root growth in search for air, nutrients and water to feed the whole plant body. Interestingly, modularity, reiteration and evolved sensing systems are among the most important problems of today robotics.
The perspective we are looking at plants in the last years is changing dramatically, tending away from seeing them as passive entities subject to environmental forces and organisms that are designed solely for accumulation of photosynthetic products. The new vision, by contrast, is that plants are dynamic and highly sensitive organisms, with complex behaviorsCitation4–Citation6 actively and competitively foraging for limited resources both above and belowground. They are also organisms, which accurately compute their circumstances, use sophisticated cost-benefit analysis and take defined actions to mitigate and control diverse environmental insults.Citation7–Citation9 Therefore, plants can be considered as information-processing organisms with complex communication throughout the individual body. In addition, the architecture of their body and their physiological attitudes make plants an unlimited source of inspiration for robotic scientists. In the next paragraphs we will discuss the possibility that plants could be very useful for robotic studies with return for both robotic and biological sciences.
Biorobotics
Biorobotics is a new scientific and technological area with a unique interdisciplinary character, aimed at increasing knowledge on how biological systems work. This objective may be obtained by (1) analyzing living organisms from a biomechatronic perspective and (2) exploiting the obtained knowledge to develop innovative methodologies and technologies (). Biorobotics encompasses the dual use of a biorobot as a tool for biologists studying living organisms’ behavior and as test-bed in the study and evaluation of biological models for potential applications in engineering. As a result, the interaction between biological science and robotics becomes two-fold: on one hand, biology provides the knowledge of the biological systems needed to build biorobots, on the other hand, bio-inspired robots represent a helpful platform for experimental validation of theories and hypotheses formulated by scientists.Citation10
In plant science, biorobots can be a valuable tool when the plant is studied as a system. Hypotheses are formulated on the overall working principle of the plant, including the interrelations among the different organs. In these studies the use of a biorobot may result advantageous, being programmable and reconfigurable to test different models and enabling plant scientists to study the complex biological processes occurring in plants in their wholeness, adapting “design-based engineering principles” to biological systems.
Robotics and biology can thus combine together in a common research program, which leads to better scientific understanding of plants, animals and humans. shows the loop from an hypothesized formal model of the biological system, to the experimental implementation and test, and then to the formal revision, that ultimately leads to the validation of the formal model as a satisfactory explanation of the biological system. This scheme allows one to ideally establish the roles of roboticists and biologists in the explanation path. Ideally, (1) biologists hypothesize the functional formal model, (2) roboticists implement the model; (3) a joint collaboration of roboticists and biologists is required during the experimental monitoring phase and finally (4) biologists do the revision.
The Plantoid—A Plant-Inspired Robot
Plants represent an excellent source of inspiration for developing a new generation of technologies, for example robots. Traditionally, robotics look to the animals for drawing inspiration in designing and developing new classes of biologically inspired robots. We think that the plant world could represent a real source of inspiration in robotics as well. Plant specific behaviors, sensing and communication capabilities, processing, control and energy storage systems, make these organisms unique and excellent examples to imitate in developing innovative and high-technology solutions.
By inspiring to the plant life a robotic system would be able to explore the above and belowground environment, acquiring information about vital parameters. A plantoid artefact with branching sensory roots would explore the soil in a more efficient way by taking inspiration by and implementing the amazing movement and growing features of plant roots. A plantoid robot would include root and shoot systems being able to change its geometrical configuration and size according to the environmental conditions.
Innumerable would be the practical applications of a plant-inspired robotic artefacts: in situ monitoring analysis and chemical detections, water searching, anchoring capabilities, as well as in developing new communication strategies or processing, and control algorithms inspired by plants. Last but not least, a plantoid would represent an excellent tool for the scientific study of the plant behavior by building physical models. This new robot generation can be considered as larger systems whose overall functionalities are designed to study the complex biological processes occurring in plants in their entirety, adapting “design-based engineering principles” to biological systems: the key principles of synthetic biology and biorobotics.
Why bother building robots instead of just using computer/numerical models? The answer to this question comes from the complexity of the sensory world represented by plants and living organisms in general. A hypothesis implemented on a robot operating in a real environment can be tested more rigorously and in a much more rational and systematic way than in simulation because the hypothesis will be challenged with real, complex and often unmodelable stimuli. Moreover, it is possible to obtain orders of magnitude more data from a robot, compared to a plant, on its actions, its sensory input, and its internal states.
A typical “biomechatronic” system () can be considered to be composed of a mechanical part (usually an articulated structure with many degrees of freedom); of a number of proprioand extero-ceptive sensors; of actuators; of energy sources; of a network of microprocessors (usually “embedded”) and of analog and digital signal processing boards; of control interfaces and of communication units.
Following the biomechatronic structure, even the plantoid can be divided in three main sub-systems: (1) a main body, carrying batteries, electronics and radio systems; (2) the root system, with electro-osmotic actuators; (3) the root apex, with sensors. Robot “leaves” would include photovoltaic cells, to assure an energy power proper for carrying out operative functionalities. Citation11 The plantoid roots will be able to grow according to the different stimuli, such as gravity and water or chemical gradients (possibly, different sensors can be included in the root). The system will include actuators for the steering of the apices according to the data coming from the sensory apparatus. Each apex will embed a microcontroller module for the emulation of the roots behavior through the local implementation of models.Citation12
Taking inspiration by plants, new communication, coordination and interaction strategies can be designed and developed. Communication in plant could be only preliminary seen as a transposition of swarm-like intelligence to: (a) a single connected organism consisting of a high number of heterogeneous, low-profiled and multifunctional parts; and (b) different organisms of this kind which can interact.
Reiteration and Swarm Behavior of Roots
Many animals when acting collectively show remarkable group behaviors. Swarming insects or flocking birds, by changing shape and direction appear to move as a single coherent organism. This kind of aggregate motion, known as “swarm behaviour”, is studied by biologists that want to understand how social animals interact or by computer scientists that try to apply the resulting “swarm intelligence” to optimize problems in fields as, for example, telecommunication, robotic, and transportation systems. Citation13–Citation15,Citation28 The advantage of using such techniques is double as they produce robust behaviors without they use of a centralized synchronization center and with very simple communication protocols among single agents. In fact, computer simulations demonstrated that both central coordination and global information are not necessary for collective behavior.Citation16–Citation18 Recently, CouzinCitation19 developed a robust model describing digitally the behavior of fish schools by identifying a minimum amount of information (repulsion, attraction, heading alignment).
If we look at the growing and explorative behavior of roots in the soil, we cannot escape to notice that despite the lacking of a central nervous system and with few evidences of communication among root apices,29 the growth pattern of the root apparatus is not chaotic at all. On the contrary it looks coordinated and efficiently shaped to exploit soil resources () and to avoid hazards. In addition, considering a plant as a colony of modular parts is not a new idea as already the Greek philosopher Theophrastus wrote that repetition is “the essence of a plant”. In the eighteenth century botanists such as Bradley, von GoetheCitation26 and Erasmus DarwinCitation20 thought that trees could be regarded as a colony of repeating parts. More recently, plants have been described as metameric organismsCitation21 i.e., their body is composed by a collection of unitary parts. Although reiterated elements have mostly been considered as leafy branch systems, HalléCitation22 posed the premise that these units would include also the root system. Effectively, because of the recursive formulation and hierarchical levels found in plant root systems, the study and the simulation of the root growth have been frequently based on the fractal analysis.Citation23,Citation24
Plant roots exhibit an exceptional capacity to sense very weak oxygen, water, temperature and nutrient gradients in the soil.Citation25 However, how they manage to navigate toward the source of the resource without being distracted by local variations, remain unclear. An interesting analogy can be found in the collective behavior adopted by birds during a long-range migration. The navigation following a very weak gradient, due to local variations, it is an almost impossible task for an individual bird, whereas a flock acting collectively overtake this obstacle by working as an integrated array of sensors.Citation27 Consequently, it is tempting to propose that a collective behavior of root tips could emerge even in plant roots from the individual activity of the single root apex. In the same way of birds, for example, individual root apex could adopt a collective behaviour that minimize the influence of local fluctuations, for exploration purposes. The “swarm rules” come out as simple rules for the different organisms: information left by the chemical traces produced by every single apex and instruction of local density may be considered the basis of such rules for roots.
Conclusions
In conclusion, robotic artefacts will reproduce a plant-like structure and the system will be designed as a distributed architecture both from the hardware and the software viewpoint. The plantoid will consist of many modules, which will represent their natural counterparts structure and functionalities, namely, leaves, branches, stem, roots, apexes. Each module will be computationally low-profiled and task-specialized (as a root apex for instance), with a high degree of autonomy, integrating its own processing unit with a stored set of basic behavioral rules that in general will depend from the specific module. The idea leads also to concepts of robustness and flexibility of the system: a plantoid composed of several distributed and self-organized modules would grant a higher probability of survival compared to a centralized system disposing of few functionalized parts, as well known from multi-agent or swarm intelligence theory.
This functionality may be used in solving such problems as e.g., energy harvesting and management, supporting of internal homeostasis, reconfiguration, sensor fusion, collective environmental awareness and others. On the basis of its own strategies and behavioural rules each functional module in the plantoid will communicate with all the other units composing the robot, making the whole plantoid a complex and highly-evolved system where decisions emerges in the form of the best compromise between a large amount of independent and integrated internal stimuli and requests.
The outcome of such a plantoid would not be limited to develop emergent decision strategies for a complex robotic system aimed at autonomously monitoring environment for extended periods of time, but it would eventually allow an invaluable feedback on the scientific knowledge of control and communications strategies intra- and inter-plants.
Figures and Tables
Figure 1 A schematic view of the loop for the formulation of a formal model of a biological system, by experimental validation.
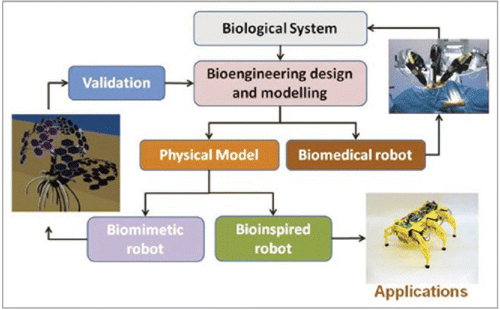
Figure 2 Conceptual scheme of the biomechatronics scientific/technological paradigm: a typical mechatronic system is characterized by the smooth and effective integration of its fundamental components (mechanisms, sensors, control, actuators, power supply), and by the fact that such integration is included in the components and system design process, from the very beginnings; biomechatronics considers the system together with its interactions with the external world and with the human operator, which become a source of biological inspiration, on one side, and a reference for functional specifications, in systems for biomedical applications, on the other.
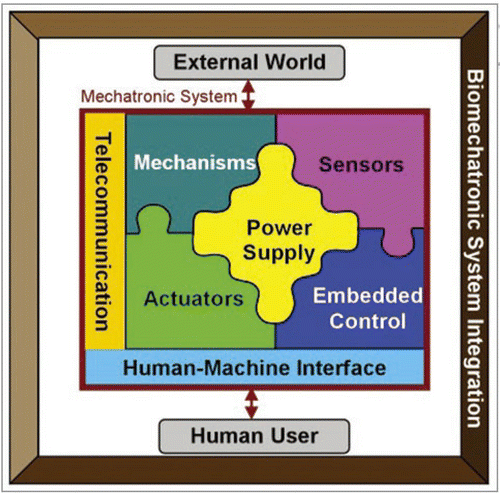
References
- Vuorisalo TO, Mutikainen PK. Vuorisalo, Mutikainen. Modularity and plant life story. Life history evolution in plants 1999; Springer
- Hodge A. Root decisions. Plant Cell Environ 2009; 32:7 - 9
- McNickle GG, Cassady St, Clair C, Cahill JF Jr. Focusing the metaphor: plant root foraging behaviour. Trends Ecol Evol 2009; 24:419 - 426
- Brenner ED, Stahlberg R, Mancuso S, Vivanco J, Baluška F, Van Volkenburgh E. Plant Neurobiology: an Integrated View of Plant Signaling. Trends Plant Sci 2006; 11:413 - 419
- Baluska F, Mancuso S. Plant neurobiology as paradigm shift not only in plant sciences. Plant Signal Behav 2007; 2:205 - 207
- Trewavas A. What is plant behaviour?. Plant Cell Environ 2009; 32:606 - 616
- Trewavas A. Plant intelligence. Naturwissenschaften 2005; 92:401 - 413
- Baluska F, Mancuso S. Plant neurobiology: from sensory biology, via plant communication, to social plant behavior. Cogn Process 2009; 10:3 - 7
- Calvo P, Keijzer F. Baluska F. Cognition in plants. Plant-environment interactions 2009; Springer
- Dario P. Biorobotics. IEEE Robotics & Automation Magazine 2003; 10:4 - 5
- Dario P, Laschi C, Mazzolai B, Corradi P, Mattoli V, Mondini A, et al. Bio-inspiration from Plants’ Roots. ESA Study Report 2008; ACT-RPT-BIOARI-066301
- Mazzolai B, Corradi P, Mondini A, Mattoli V, Laschi C, Mancuso S, et al. Inspiration from plant roots: a robotic root apex for soil exploration. Proceedings of Biological Approaches for Engineering, University of Southampton 2008; 50 - 53
- Beni G, Wang J. Swarm intelligence in cellular robotics systems. Proceeding of NATO Advanced Workshop on Robots and Biological System 1989;
- Arkin R. Behavior-Based Robotics 1998; Cambridge, MA MIT Press
- Bonabeau E, Dorigo M, Theraulaz G. Swarm Intelligence: From Natural to Artificial Systems 1999; Oxford Univ Press
- Aoki I. A simulation study on the schooling mechanism in fish. Bull Jap Soc Sci Fish 1982; 48:1081 - 1088
- Huth A, Wissel C. The simulation of the movement of fish schools. J Theor Biol 1992; 156:365 - 385
- Czirok A, Vicsek M, Vicsek T. Collective motion of organisms in three dimensions. Physica A 1999; 264:299 - 304
- Couzin ID, Krause J, Franks NR, Levin SA. Effective leadership and decision making in animal groups on the move. Nature 2005; 433:513 - 516
- Darwin E. Phytologia: or the Philosophy of Agriculture and Gardening London J. Johnson 1800
- White J. The plant as a metapopulation. Annual Review of Ecology and Systematics 1979; 10:109 - 145
- Hallé F. Lowman MD, Nadkarni NM. Canopy architecture in tropical trees: a pictorial approach. Forest Canopies 1995; London Academic Press, 27 - 44
- Pagès L, Jordan MO, Picard D. Simulation model of the three-dimensional architecture of the maize root system. Plant and Soil 1989; 119:147 - 154
- Ozier-Lafontaine H, Lecompte F, Sillon JF. Fractal analysis of the root architecture of Gliricidia sepium for the spatial prediction of root branching, size and mass: model development and evaluation in agroforestry. Plant and Soil 1999; 209:167 - 179
- Gilroy S, Trewavas A. Signal processing and transduction in plant cells: the end of the beginning?. Nat Rev Mol Cell Biol 2001; 2:307 - 314
- von Goethe JW. Versuch die Metamorphose der Pflanzen zu erklären 1790; Gotha
- Couzin I. Collective minds. Nature 2007; 445:715
- Krause J, Ruxton GD, Krause S. Swarm intelligence in animals and humans. Trends Ecol Evol 2009; 25:28 - 34